Ligand-gated ion channel
Ligand-gated ion channels (LICs, LGIC), also commonly referred to as ionotropic receptors, are a group of transmembrane ion-channel proteins which open to allow ions such as Na+, K+, Ca2+, and/or Cl− to pass through the membrane in response to the binding of a chemical messenger (i.e. a ligand), such as a neurotransmitter.[1][2][3]
Neurotransmitter-gated ion-channel transmembrane region | |||||||||
---|---|---|---|---|---|---|---|---|---|
![]() Ligand-gated ion channel | |||||||||
Identifiers | |||||||||
Symbol | Neur_chan_memb | ||||||||
Pfam | PF02932 | ||||||||
InterPro | IPR006029 | ||||||||
PROSITE | PDOC00209 | ||||||||
SCOPe | 1cek / SUPFAM | ||||||||
TCDB | 1.A.9 | ||||||||
OPM superfamily | 14 | ||||||||
OPM protein | 2bg9 | ||||||||
|
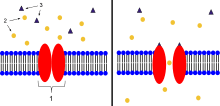
- Ion-channel-linked receptor
- Ions
- Ligand (such as acetylcholine)
When a presynaptic neuron is excited, it releases a neurotransmitter from vesicles into the synaptic cleft. The neurotransmitter then binds to receptors located on the postsynaptic neuron. If these receptors are ligand-gated ion channels, a resulting conformational change opens the ion channels, which leads to a flow of ions across the cell membrane. This, in turn, results in either a depolarization, for an excitatory receptor response, or a hyperpolarization, for an inhibitory response.
These receptor proteins are typically composed of at least two different domains: a transmembrane domain which includes the ion pore, and an extracellular domain which includes the ligand binding location (an allosteric binding site). This modularity has enabled a 'divide and conquer' approach to finding the structure of the proteins (crystallising each domain separately). The function of such receptors located at synapses is to convert the chemical signal of presynaptically released neurotransmitter directly and very quickly into a postsynaptic electrical signal. Many LICs are additionally modulated by allosteric ligands, by channel blockers, ions, or the membrane potential. LICs are classified into three superfamilies which lack evolutionary relationship: cys-loop receptors, ionotropic glutamate receptors and ATP-gated channels.
Cys-loop receptors
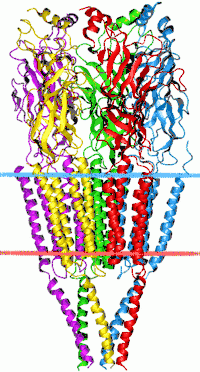
The cys-loop receptors are named after a characteristic loop formed by a disulfide bond between two cysteine residues in the N terminal extracellular domain. They are part of a larger family of pentameric ligand-gated ion channels that usually lack this disulfide bond, hence the tentative name "Pro-loop receptors".[4][5] A binding site in the extracellular N-terminal ligand-binding domain gives them receptor specificity for (1) acetylcholine (AcCh), (2) serotonin, (3) glycine, (4) glutamate and (5) γ-aminobutyric acid (GABA) in vertebrates. The receptors are subdivided with respect to the type of ion that they conduct (anionic or cationic) and further into families defined by the endogenous ligand. They are usually pentameric with each subunit containing 4 transmembrane helices constituting the transmembrane domain, and a beta sheet sandwich type, extracellular, N terminal, ligand binding domain.[6] Some also contain an intracellular domain like shown in the image.
The prototypic ligand-gated ion channel is the nicotinic acetylcholine receptor. It consists of a pentamer of protein subunits (typically ααβγδ), with two binding sites for acetylcholine (one at the interface of each alpha subunit). When the acetylcholine binds it alters the receptor's configuration (twists the T2 helices which moves the leucine residues, which block the pore, out of the channel pathway) and causes the constriction in the pore of approximately 3 angstroms to widen to approximately 8 angstroms so that ions can pass through. This pore allows Na+ ions to flow down their electrochemical gradient into the cell. With a sufficient number of channels opening at once, the inward flow of positive charges carried by Na+ ions depolarizes the postsynaptic membrane sufficiently to initiate an action potential.
While single-cell organisms like bacteria would have little apparent need for the transmission of an action potential, a bacterial homologue to an LIC has been identified, hypothesized to act nonetheless as a chemoreceptor.[4] This prokaryotic nAChR variant is known as the GLIC receptor, after the species in which it was identified; Gloeobacter Ligand-gated Ion C channel.
Structure
Cys-loop receptors have structural elements that are well conserved, with a large extracellular domain (ECD) harboring an alpha-helix and 10 beta-strands. Following the ECD, four transmembrane segments (TMSs) are connected by intracellular and extracellular loop structures.[7] Except the TMS 3-4 loop, their lengths are only 7-14 residues. The TMS 3-4 loop forms the largest part of the intracellular domain (ICD) and exhibits the most variable region between all of these homologous receptors. The ICD is defined by the TMS 3-4 loop together with the TMS 1-2 loop preceding the ion channel pore.[7] Crystallization has revealed structures for some members of the family, but to allow crystallization, the intracellular loop was usually replaced by a short linker present in prokaryotic cys-loop receptors, so their structures as not known. Nevertheless, this intracellular loop appears to function in desensitization, modulation of channel physiology by pharmacological substances, and posttranslational modifications. Motifs important for trafficking are therein, and the ICD interacts with scaffold proteins enabling inhibitory synapse formation.[7]
Cationic cys-loop receptors
Type | Class | IUPHAR-recommended protein name [8] |
Gene | Previous names |
---|---|---|---|---|
Serotonin (5-HT) |
5-HT3 | 5-HT3A 5-HT3B 5-HT3C 5-HT3D 5-HT3E |
HTR3A HTR3B HTR3C HTR3D HTR3E |
5-HT3A 5-HT3B 5-HT3C 5-HT3D 5-HT3E |
Nicotinic acetylcholine (nAChR) |
alpha | α1 α2 α3 α4 α5 α6 α7 α9 α10 |
CHRNA1 CHRNA2 CHRNA3 CHRNA4 CHRNA5 CHRNA6 CHRNA7 CHRNA9 CHRNA10 |
ACHRA, ACHRD, CHRNA, CMS2A, FCCMS, SCCMS |
beta | β1 β2 β3 β4 |
CHRNB1 CHRNB2 CHRNB3 CHRNB4 |
CMS2A, SCCMS, ACHRB, CHRNB, CMS1D EFNL3, nAChRB2 | |
gamma | γ | CHRNG | ACHRG | |
delta | δ | CHRND | ACHRD, CMS2A, FCCMS, SCCMS | |
epsilon | ε | CHRNE | ACHRE, CMS1D, CMS1E, CMS2A, FCCMS, SCCMS | |
Zinc-activated ion channel (ZAC) |
ZAC | ZACN | ZAC1, L2m LICZ, LICZ1 |
Anionic cys-loop receptors
Type | Class | IUPHAR-recommended protein name[8] |
Gene | Previous names |
---|---|---|---|---|
GABAA | alpha | α1 α2 α3 α4 α5 α6 |
GABRA1 GABRA2 GABRA3 GABRA4 GABRA5 GABRA6 |
EJM, ECA4 |
beta | β1 β2 β3 |
GABRB1 GABRB2 GABRB3 |
ECA5 | |
gamma | γ1 γ2 γ3 |
GABRG1 GABRG2 GABRG3 |
CAE2, ECA2, GEFSP3 | |
delta | δ | GABRD | ||
epsilon | ε | GABRE | ||
pi | π | GABRP | ||
theta | θ | GABRQ | ||
rho | ρ1 ρ2 ρ3 |
GABRR1 GABRR2 GABRR3 |
GABAC[9] | |
Glycine (GlyR) |
alpha | α1 α2 α3 α4 |
GLRA1 GLRA2 GLRA3 GLRA4 |
STHE |
beta | β | GLRB |
Ionotropic glutamate receptors
The ionotropic glutamate receptors bind the neurotransmitter glutamate. They form tetramers with each subunit consisting of an extracellular amino terminal domain (ATD, which is involved tetramer assembly), an extracellular ligand binding domain (LBD, which binds glutamate), and a transmembrane domain (TMD, which forms the ion channel). The transmembrane domain of each subunit contains three transmembrane helices as well as a half membrane helix with a reentrant loop. The structure of the protein starts with the ATD at the N terminus followed by the first half of the LBD which is interrupted by helices 1,2 and 3 of the TMD before continuing with the final half of the LBD and then finishing with helix 4 of the TMD at the C terminus. This means there are three links between the TMD and the extracellular domains. Each subunit of the tetramer has a binding site for glutamate formed by the two LBD sections forming a clamshell like shape. Only two of these sites in the tetramer need to be occupied to open the ion channel. The pore is mainly formed by the half helix 2 in a way which resembles an inverted potassium channel.
Type | Class | IUPHAR-recommended protein name [8] |
Gene | Previous names |
---|---|---|---|---|
AMPA | GluA | GluA1 GluA2 GluA3 GluA4 |
GRIA1 GRIA2 GRIA3 GRIA4 |
GLUA1, GluR1, GluRA, GluR-A, GluR-K1, HBGR1 GLUA2, GluR2, GluRB, GluR-B, GluR-K2, HBGR2 GLUA3, GluR3, GluRC, GluR-C, GluR-K3 GLUA4, GluR4, GluRD, GluR-D |
Kainate | GluK | GluK1 GluK2 GluK3 GluK4 GluK5 |
GRIK1 GRIK2 GRIK3 GRIK4 GRIK5 |
GLUK5, GluR5, GluR-5, EAA3 GLUK6, GluR6, GluR-6, EAA4 GLUK7, GluR7, GluR-7, EAA5 GLUK1, KA1, KA-1, EAA1 GLUK2, KA2, KA-2, EAA2 |
NMDA | GluN | GluN1 NRL1A NRL1B |
GRIN1 GRINL1A GRINL1B |
GLUN1, NMDA-R1, NR1, GluRξ1 |
GluN2A GluN2B GluN2C GluN2D |
GRIN2A GRIN2B GRIN2C GRIN2D |
GLUN2A, NMDA-R2A, NR2A, GluRε1 GLUN2B, NMDA-R2B, NR2B, hNR3, GluRε2 GLUN2C, NMDA-R2C, NR2C, GluRε3 GLUN2D, NMDA-R2D, NR2D, GluRε4 | ||
GluN3A GluN3B |
GRIN3A GRIN3B |
GLUN3A, NMDA-R3A, NMDAR-L, chi-1 GLU3B, NMDA-R3B | ||
‘Orphan’ | (GluD) | GluD1 GluD2 |
GRID1 GRID2 |
GluRδ1 GluRδ2 |
AMPA receptor
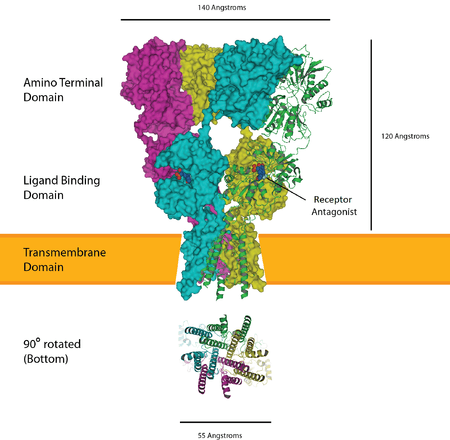
The α-amino-3-hydroxy-5-methyl-4-isoxazolepropionic acid receptor (also known as AMPA receptor, or quisqualate receptor) is a non-NMDA-type ionotropic transmembrane receptor for glutamate that mediates fast synaptic transmission in the central nervous system (CNS). Its name is derived from its ability to be activated by the artificial glutamate analog AMPA. The receptor was first named the "quisqualate receptor" by Watkins and colleagues after a naturally occurring agonist quisqualate and was only later given the label "AMPA receptor" after the selective agonist developed by Tage Honore and colleagues at the Royal Danish School of Pharmacy in Copenhagen.[10] AMPARs are found in many parts of the brain and are the most commonly found receptor in the nervous system. The AMPA receptor GluA2 (GluR2) tetramer was the first glutamate receptor ion channel to be crystallized.
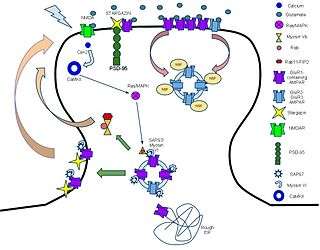
Ligands:
- Agonists: Glutamate, AMPA, 5-Fluorowillardiine, Domoic acid, Quisqualic acid, etc.
- Antagonists: CNQX, Kynurenic acid, NBQX, Perampanel, Piracetam, etc.
- Positive allosteric modulators: Aniracetam, Cyclothiazide, CX-516, CX-614, etc.
- Negative allosteric modulators: Ethanol, Perampanel, Talampanel, GYKI-52,466, etc.
NMDA receptors

The N-methyl-D-aspartate receptor (NMDA receptor) – a type of ionotropic glutamate receptor – is a ligand-gated ion channel that is gated by the simultaneous binding of glutamate and a co-agonist (i.e., either D-serine or glycine).[11] Studies show that the NMDA receptor is involved in regulating synaptic plasticity and memory.[12][13]
The name "NMDA receptor" is derived from the ligand N-methyl-D-aspartate (NMDA), which acts as a selective agonist at these receptors. When the NMDA receptor is activated by the binding of two co-agonists, the cation channel opens, allowing Na+ and Ca2+ to flow into the cell, in turn raising the cell's electric potential. Thus, the NMDA receptor is an excitatory receptor. At resting potentials, the binding of Mg2+ or Zn2+ at their extracellular binding sites on the receptor blocks ion flux through the NMDA receptor channel. "However, when neurons are depolarized, for example, by intense activation of colocalized postsynaptic AMPA receptors, the voltage-dependent block by Mg2+ is partially relieved, allowing ion influx through activated NMDA receptors. The resulting Ca2+ influx can trigger a variety of intracellular signaling cascades, which can ultimately change neuronal function through activation of various kinases and phosphatases".[14]
Ligands:
- Primary endogenous co-agonists: glutamate and either D-serine or glycine
- Other agonists : aminocyclopropanecarboxylic acid; D-cycloserine; L-aspartate; quinolinate, etc.
- Partial agonists : N-methyl-D-aspartic acid (NMDA); NRX-1074; 3,5-dibromo-L-phenylalanine,[15] etc.
- Antagonists: ketamine, PCP, dextropropoxyphene, ketobemidone, tramadol, kynurenic acid (endogenous), etc.
GABA receptors
GABA receptors are major inhibitory neurotransmitter expressed in the major interneurons in animal cortex.
GABAA receptor
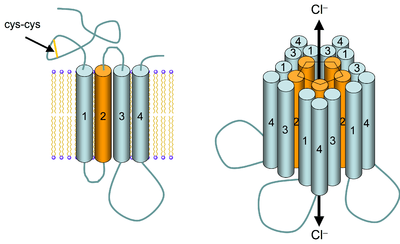
GABAA receptors are ligand-gated ion channels. GABA (gamma-aminobutyric acid), the endogenous ligand for these receptors, is the major inhibitory neurotransmitter in the central nervous system. When activated, it mediates Cl– flow into the neuron, hyperpolarizing the neuron. GABAA receptors occur in all organisms that have a nervous system. Due to their wide distribution within the nervous system of mammals, they play a role in virtually all brain functions.[16]
Various ligands can bind specifically to GABAA receptors, either activating or inhibiting the Cl– channel.
Ligands:
- Agonists: GABA, muscimol, progabide, gaboxadol
- Antagonists: bicuculine, gabazine
- Partial agonist: piperidine-4-sulfonic acid
5-HT3 receptor
The pentameric 5-HT3 receptor is permeable to sodium (Na), potassium (K), and calcium (Ca) ions.
ATP-gated channels
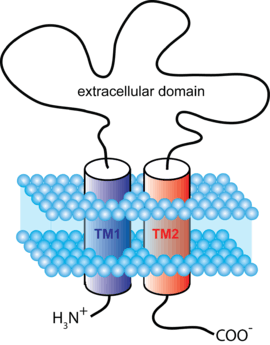
ATP-gated channels open in response to binding the nucleotide ATP. They form trimers with two transmembrane helices per subunit and both the C and N termini on the intracellular side.
Type | Class | IUPHAR-recommended protein name [8] |
Gene | Previous names |
---|---|---|---|---|
P2X | N/A | P2X1 P2X2 P2X3 P2X4 P2X5 P2X6 P2X7 |
P2RX1 P2RX2 P2RX3 P2RX4 P2RX5 P2RX6 P2RX7 |
P2X1 P2X2 P2X3 P2X4 P2X5 P2X6 P2X7 |
PIP2-gated channels
Phosphatidylinositol 4,5-bisphosphate (PIP2) binds to and directly activates inwardly rectifying potassium channels (Kir).[17] PIP2 is a cell membrane lipid, and its role in gating ion channels represents a novel role for the molecule.[18][19]
Indirect modulation
In contrast to ligand-gated ion channels, there are also receptor systems in which the receptor and the ion channel are separate proteins in the cell membrane, instead of a single molecule. In this case, ion channels are indirectly modulated by activation of the receptor, instead of being gated directly.
G-protein-linked receptors
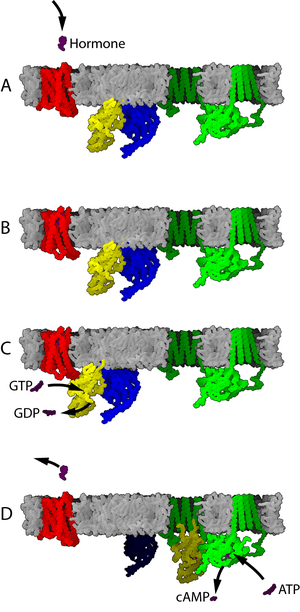
Also called G protein-coupled receptor, seven-transmembrane domain receptor, 7 TM receptor, constitute a large protein family of receptors that sense molecules outside the cell and activate inside signal transduction pathways and, ultimately, cellular responses. They pass through the cell membrane 7 times. G-protein-Linked receptors are a huge family that have hundreds of members identified. Ion-channel-linked receptors (e.g. GABAB, NMDA, etc.) are only a part of them.
Table 1. Three major families of Trimeric G Proteins[20]
FAMILY | SOME FAMILY MEMBERS | ACTION MEDIATED BY | FUNCTIONS |
---|---|---|---|
I | GS | α | Activate adenylyl cyclase activates Ca2+ channels |
Golf | α | Activates adenylyl cyclase in olfactory sensory neurons | |
II | Gi | α | Inhibits adenylyl cyclase |
βγ | Activates K+ channels | ||
G0 | βγ | Activates K+ channels; inactivate Ca2+ channels | |
α and βγ | Activates phospholipase C-β | ||
Gt (transducin) | α | Activate cyclic GMP phosphodiesterase in vertebrate rod photoreceptors | |
III | Gq | α | Activates phospholipase C-β |
GABAB receptor
GABAB receptors are metabotropic transmembrane receptors for gamma-aminobutyric acid. They are linked via G-proteins to K+ channels, when active, they create hyperpolarized effect and lower the potential inside the cell.[21]
Ligands:
Gα signaling
The cyclic-adenosine monophosphate (cAMP)-generating enzyme adenylate cyclase is the effector of both the Gαs and Gαi/o pathways. Ten different AC gene products in mammals, each with subtle differences in tissue distribution and/or function, all catalyze the conversion of cytosolic adenosine triphosphate (ATP) to cAMP, and all are directly stimulated by G-proteins of the Gαs class. Interaction with Gα subunits of the Gαi/o type, on the contrary, inhibits AC from generating cAMP. Thus, a GPCR coupled to Gαs counteracts the actions of a GPCR coupled to Gαi/o, and vice versa. The level of cytosolic cAMP may then determine the activity of various ion channels as well as members of the ser/thr-specific protein kinase A (PKA) family. As a result, cAMP is considered a second messenger and PKA a secondary effector.
The effector of the Gαq/11 pathway is phospholipase C-β (PLCβ), which catalyzes the cleavage of membrane-bound phosphatidylinositol 4,5-biphosphate (PIP2) into the second messengers inositol (1,4,5) trisphosphate (IP3) and diacylglycerol (DAG). IP3 acts on IP3 receptors found in the membrane of the endoplasmic reticulum (ER) to elicit Ca2+ release from the ER, DAG diffuses along the plasma membrane where it may activate any membrane localized forms of a second ser/thr kinase called protein kinase C (PKC). Since many isoforms of PKC are also activated by increases in intracellular Ca2+, both these pathways can also converge on each other to signal through the same secondary effector. Elevated intracellular Ca2+ also binds and allosterically activates proteins called calmodulins, which in turn go on to bind and allosterically activate enzymes such as Ca2+/calmodulin-dependent kinases (CAMKs).
The effectors of the Gα12/13 pathway are three RhoGEFs (p115-RhoGEF, PDZ-RhoGEF, and LARG), which, when bound to Gα12/13 allosterically activate the cytosolic small GTPase, Rho. Once bound to GTP, Rho can then go on to activate various proteins responsible for cytoskeleton regulation such as Rho-kinase (ROCK). Most GPCRs that couple to Gα12/13 also couple to other sub-classes, often Gαq/11.
Gβγ signaling
The above descriptions ignore the effects of Gβγ–signalling, which can also be important, in particular in the case of activated Gαi/o-coupled GPCRs. The primary effectors of Gβγ are various ion channels, such as G-protein-regulated inwardly rectifying K+ channels (GIRKs), P/Q- and N-type voltage-gated Ca2+ channels, as well as some isoforms of AC and PLC, along with some phosphoinositide-3-kinase (PI3K) isoforms.
Clinical relevance
Ligand-gated ion channels are likely to be the major site at which anaesthetic agents and ethanol have their effects, although unequivocal evidence of this is yet to be established.[23][24] In particular, the GABA and NMDA receptors are affected by anaesthetic agents at concentrations similar to those used in clinical anaesthesia.[25]
By understanding the mechanism and exploring the chemical/biological/physical component that could function on those receptors, more and more clinical applications are proven by preliminary experiments or FDA.
Memantine is approved by the U.S. F.D.A and the European Medicines Agency for the treatment of moderate-to-severe Alzheimer's disease,[26] and has now received a limited recommendation by the UK's National Institute for Health and Care Excellence for patients who fail other treatment options.[27]
- Antidepressant treatment
Agomelatine, is a type of drug that acts on a dual melatonergic-serotonergic pathway, which have shown its efficacy in the treatment of anxious depression during clinical trails,[28][29] study also suggests the efficacy in the treatment of atypical and melancholic depression.[30]
See also
- Receptor (biochemistry)
- Action potential
- Voltage-dependent calcium channel
- Calcium-activated potassium channel
- Cyclic nucleotide-gated ion channel
- Acid-sensing ion channel
- Ryanodine receptor
- Inositol trisphosphate receptor
References
- "Gene Family: Ligand gated ion channels". HUGO Gene Nomenclature Committee.
- "ligand-gated channel" at Dorland's Medical Dictionary
- Purves, Dale, George J. Augustine, David Fitzpatrick, William C. Hall, Anthony-Samuel LaMantia, James O. McNamara, and Leonard E. White (2008). Neuroscience. 4th ed. Sinauer Associates. pp. 156–7. ISBN 978-0-87893-697-7.CS1 maint: multiple names: authors list (link)
- Tasneem A, Iyer LM, Jakobsson E, Aravind L (2004). "Identification of the prokaryotic ligand-gated ion channels and their implications for the mechanisms and origins of animal Cys-loop ion channels". Genome Biology. 6 (1): R4. doi:10.1186/gb-2004-6-1-r4. PMC 549065. PMID 15642096.
- Jaiteh M, Taly A, Hénin J (2016). "Evolution of Pentameric Ligand-Gated Ion Channels: Pro-Loop Receptors". PLOS ONE. 11 (3): e0151934. Bibcode:2016PLoSO..1151934J. doi:10.1371/journal.pone.0151934. PMC 4795631. PMID 26986966.
- Cascio M (May 2004). "Structure and function of the glycine receptor and related nicotinicoid receptors". The Journal of Biological Chemistry. 279 (19): 19383–6. doi:10.1074/jbc.R300035200. PMID 15023997.
- Langlhofer G, Villmann C (2016-01-01). "The Intracellular Loop of the Glycine Receptor: It's not all about the Size". Frontiers in Molecular Neuroscience. 9: 41. doi:10.3389/fnmol.2016.00041. PMC 4891346. PMID 27330534.
- Collingridge GL, Olsen RW, Peters J, Spedding M (January 2009). "A nomenclature for ligand-gated ion channels". Neuropharmacology. 56 (1): 2–5. doi:10.1016/j.neuropharm.2008.06.063. PMC 2847504. PMID 18655795.
- Olsen RW, Sieghart W (September 2008). "International Union of Pharmacology. LXX. Subtypes of gamma-aminobutyric acid(A) receptors: classification on the basis of subunit composition, pharmacology, and function. Update". Pharmacological Reviews. 60 (3): 243–60. doi:10.1124/pr.108.00505. PMC 2847512. PMID 18790874.
- Honoré T, Lauridsen J, Krogsgaard-Larsen P (January 1982). "The binding of [3H]AMPA, a structural analogue of glutamic acid, to rat brain membranes". Journal of Neurochemistry. 38 (1): 173–8. doi:10.1111/j.1471-4159.1982.tb10868.x. PMID 6125564.
- Malenka RC, Nestler EJ, Hyman SE (2009). "Chapter 5: Excitatory and Inhibitory Amino Acids". In Sydor A, Brown RY (eds.). Molecular Neuropharmacology: A Foundation for Clinical Neuroscience (2nd ed.). New York, USA: McGraw-Hill Medical. pp. 124–125. ISBN 9780071481274.
At membrane potentials more negative than approximately −50 mV, the Mg2+ in the extracellular fluid of the brain virtually abolishes ion flux through NMDA receptor channels, even in the presence of glutamate. ... The NMDA receptor is unique among all neurotransmitter receptors in that its activation requires the simultaneous binding of two different agonists. In addition to the binding of glutamate at the conventional agonist-binding site, the binding of glycine appears to be required for receptor activation. Because neither of these agonists alone can open this ion channel, glutamate and glycine are referred to as coagonists of the NMDA receptor. The physiologic significance of the glycine binding site is unclear because the normal extracellular concentration of glycine is believed to be saturating. However, recent evidence suggests that D-serine may be the endogenous agonist for this site.
- Li F, Tsien JZ (July 2009). "Memory and the NMDA receptors". The New England Journal of Medicine. 361 (3): 302–3. doi:10.1056/NEJMcibr0902052. PMC 3703758. PMID 19605837.
- Cao X, Cui Z, Feng R, Tang YP, Qin Z, Mei B, Tsien JZ (March 2007). "Maintenance of superior learning and memory function in NR2B transgenic mice during ageing". The European Journal of Neuroscience. 25 (6): 1815–22. doi:10.1111/j.1460-9568.2007.05431.x. PMID 17432968.
- Dingledine R, Borges K, Bowie D, Traynelis SF (March 1999). "The glutamate receptor ion channels". Pharmacological Reviews. 51 (1): 7–61. PMID 10049997.
- Yarotskyy V, Glushakov AV, Sumners C, Gravenstein N, Dennis DM, Seubert CN, Martynyuk AE (May 2005). "Differential modulation of glutamatergic transmission by 3,5-dibromo-L-phenylalanine". Molecular Pharmacology. 67 (5): 1648–54. doi:10.1124/mol.104.005983. PMID 15687225.
- Wu C, Sun D (April 2015). "GABA receptors in brain development, function, and injury". Metabolic Brain Disease. 30 (2): 367–79. doi:10.1007/s11011-014-9560-1. PMC 4231020. PMID 24820774.
- Hansen SB, Tao X, MacKinnon R (August 2011). "Structural basis of PIP2 activation of the classical inward rectifier K+ channel Kir2.2". Nature. 477 (7365): 495–8. Bibcode:2011Natur.477..495H. doi:10.1038/nature10370. PMC 3324908. PMID 21874019.
- Hansen SB (May 2015). "Lipid agonism: The PIP2 paradigm of ligand-gated ion channels". Biochimica et Biophysica Acta (BBA) - Molecular and Cell Biology of Lipids. 1851 (5): 620–8. doi:10.1016/j.bbalip.2015.01.011. PMC 4540326. PMID 25633344.
- Gao Y, Cao E, Julius D, Cheng Y (June 2016). "TRPV1 structures in nanodiscs reveal mechanisms of ligand and lipid action". Nature. 534 (7607): 347–51. Bibcode:2016Natur.534..347G. doi:10.1038/nature17964. PMC 4911334. PMID 27281200.
- Lodish, Harvey. Molecular cell biology. Macmillan, 2008.
- Chen K, Li HZ, Ye N, Zhang J, Wang JJ (October 2005). "Role of GABAB receptors in GABA and baclofen-induced inhibition of adult rat cerebellar interpositus nucleus neurons in vitro". Brain Research Bulletin. 67 (4): 310–8. doi:10.1016/j.brainresbull.2005.07.004. PMID 16182939.
- Urwyler S, Mosbacher J, Lingenhoehl K, Heid J, Hofstetter K, Froestl W, Bettler B, Kaupmann K (November 2001). "Positive allosteric modulation of native and recombinant gamma-aminobutyric acid(B) receptors by 2,6-Di-tert-butyl-4-(3-hydroxy-2,2-dimethyl-propyl)-phenol (CGP7930) and its aldehyde analog CGP13501". Molecular Pharmacology. 60 (5): 963–71. doi:10.1124/mol.60.5.963. PMID 11641424.
- Krasowski MD, Harrison NL (August 1999). "General anaesthetic actions on ligand-gated ion channels". Cellular and Molecular Life Sciences. 55 (10): 1278–303. doi:10.1007/s000180050371. PMC 2854026. PMID 10487207.
- Dilger JP (July 2002). "The effects of general anaesthetics on ligand-gated ion channels". British Journal of Anaesthesia. 89 (1): 41–51. doi:10.1093/bja/aef161. PMID 12173240.
- Harris RA, Mihic SJ, Dildy-Mayfield JE, Machu TK (November 1995). "Actions of anesthetics on ligand-gated ion channels: role of receptor subunit composition" (abstract). FASEB Journal. 9 (14): 1454–62. doi:10.1096/fasebj.9.14.7589987. PMID 7589987.
- Mount C, Downton C (July 2006). "Alzheimer disease: progress or profit?". Nature Medicine. 12 (7): 780–4. doi:10.1038/nm0706-780. PMID 16829947.
- NICE technology appraisal January 18, 2011 Azheimer's disease - donepezil, galantamine, rivastigmine and memantine (review): final appraisal determination
- Heun, R; Coral, RM; Ahokas, A; Nicolini, H; Teixeira, JM; Dehelean, P (2013). "1643 – Efficacy of agomelatine in more anxious elderly depressed patients. A randomized, double-blind study vs placebo". European Psychiatry. 28 (Suppl 1): 1. doi:10.1016/S0924-9338(13)76634-3.
- Brunton, L; Chabner, B; Knollman, B (2010). Goodman and Gilman's The Pharmacological Basis of Therapeutics (12th ed.). New York: McGraw-Hill Professional. ISBN 978-0-07-162442-8.
- Avedisova, A; Marachev, M (2013). "2639 – The effectiveness of agomelatine (valdoxan) in the treatment of atypical depression". European Psychiatry. 28 (Suppl 1): 1. doi:10.1016/S0924-9338(13)77272-9.
External links
![]() |
Wikimedia Commons has media related to Ligand-gated ion channel. |
- Ligand-Gated Ion Channel database at European Bioinformatics Institute. Verified availability April 11, 2007.
- "Revised Recommendations for Nomenclature of Ligand-Gated Ion Channels". IUPHAR Database of Receptors and Ion Channels. International Union of Basic and Clinical Pharmacology.
- www.esf.edu
- www.genenames.org
As of this edit, this article uses content from "1.A.9 The Neurotransmitter Receptor, Cys loop, Ligand-gated Ion Channel (LIC) Family", which is licensed in a way that permits reuse under the Creative Commons Attribution-ShareAlike 3.0 Unported License, but not under the GFDL. All relevant terms must be followed.