Muscarinic acetylcholine receptor
Muscarinic acetylcholine receptors, or mAChRs, are acetylcholine receptors that form G protein-coupled receptor complexes in the cell membranes of certain neurons[1] and other cells. They play several roles, including acting as the main end-receptor stimulated by acetylcholine released from postganglionic fibers in the parasympathetic nervous system.


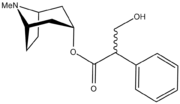
Muscarinic receptors are so named because they are more sensitive to muscarine than to nicotine.[2] Their counterparts are nicotinic acetylcholine receptors (nAChRs), receptor ion channels that are also important in the autonomic nervous system. Many drugs and other substances (for example pilocarpine and scopolamine) manipulate these two distinct receptors by acting as selective agonists or antagonists.[3]
Function
Acetylcholine (ACh) is a neurotransmitter found in the brain, neuromuscular junctions and the autonomic ganglia. Muscarinic receptors are used in the following roles:
Recovery receptors
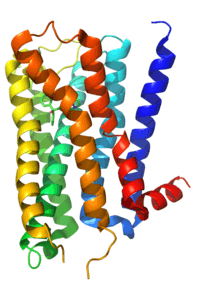
ACh is always used as the neurotransmitter within the autonomic ganglion. Nicotinic receptors on the postganglionic neuron are responsible for the initial fast depolarization (Fast EPSP) of that neuron. As a consequence of this, nicotinic receptors are often cited as the receptor on the postganglionic neurons at the ganglion. However, the subsequent hyperpolarization (IPSP) and slow depolarization (Slow EPSP) that represent the recovery of the postganglionic neuron from stimulation are actually mediated by muscarinic receptors, types M2 and M1 respectively (discussed below).
Peripheral autonomic fibers (sympathetic and parasympathetic fibers) are categorized anatomically as either preganglionic or postganglionic fibers, then further generalized as either adrenergic fibers, releasing noradrenaline, or cholinergic fibers, both releasing acetylcholine and expressing acetylcholine receptors. Both preganglionic sympathetic fibers and preganglionic parasympathetic fibers are cholinergic. Most postganglionic sympathetic fibers are adrenergic: their neurotransmitter is norepinephrine except postganglionic sympathetic fibers to the sweat glands, piloerectile muscles of the body hairs, and the skeletal muscle arterioles do not use adrenaline/noradrenaline.
The adrenal medulla is considered a sympathetic ganglion and, like other sympathetic ganglia, is supplied by cholinergic preganglionic sympathetic fibers: acetylcholine is the neurotransmitter utilized at this synapse. The chromaffin cells of the adrenal medulla act as "modified neurons", releasing adrenaline and noradrenaline into the bloodstream as hormones instead of as neurotransmitters. The other postganglionic fibers of the peripheral autonomic system belong to the parasympathetic division; all are cholinergic fibers, and use acetylcholine as the neurotransmitter.
Postganglionic neurons
Another role for these receptors is at the junction of the innervated tissues and the postganglionic neurons in the parasympathetic division of the autonomic nervous system. Here acetylcholine is again used as a neurotransmitter, and muscarinic receptors form the principal receptors on the innervated tissue.
Innervated tissue
Very few parts of the sympathetic system use cholinergic receptors. In sweat glands the receptors are of the muscarinic type. The sympathetic nervous system also has some preganglionic nerves terminating at the chromaffin cells in the adrenal medulla, which secrete epinephrine and norepinephrine into the bloodstream. Some believe that chromaffin cells are modified postganglionic CNS fibers. In the adrenal medulla, acetylcholine is used as a neurotransmitter, and the receptor is of the nicotinic type.
The somatic nervous system uses a nicotinic receptor to acetylcholine at the neuromuscular junction.
Higher central nervous system
Muscarinic acetylcholine receptors are also present and distributed throughout the local nervous system, in post-synaptic and pre-synaptic positions. There is also some evidence for postsynaptic receptors on sympathetic neurons allowing the parasympathetic nervous system to inhibit sympathetic effects.
Presynaptic membrane of the neuromuscular junction
It is known that muscarinic acetylcholine receptors also appear on the pre-synaptic membrane of somatic neurons in the neuro-muscular junction, where they are involved in the regulation of acetylcholine release.
Form of muscarinic receptors
Muscarinic acetylcholine receptors belong to a class of metabotropic receptors that use G proteins as their signaling mechanism. In such receptors, the signaling molecule (the ligand) binds to a receptor that has seven transmembrane regions; in this case, the ligand is ACh. This receptor is bound to intracellular proteins, known as G proteins, which begin the information cascade within the cell.[4]
By contrast, nicotinic receptors use a ligand-gated ion channel mechanism for signaling. In this case, binding of the ligands with the receptor causes an ion channel to open, permitting either one or more specific type(s) of ion (e.g., K+, Na+, Ca2+) to diffuse into or out of the cell.
Receptor isoforms
Classification
By the use of selective radioactively labeled agonist and antagonist substances, five subtypes of muscarinic receptors have been determined, named M1-M5 (using an upper case M and subscript number).[5] M1,M3,M5 receptors are coupled with Gq proteins, while M2 and M4 receptors are coupled with Gi/o proteins.[4] There are other classification systems. For example, the drug pirenzepine is a muscarinic antagonist (decreases the effect of ACh), which is much more potent at M1 receptors than it is at other subtypes. The acceptance of the various subtypes has proceeded in numerical order: therefore, sources that recognize only the M1/M2 distinction exist. More recent studies tend to recognize M3 and the most recent M4.
Genetic differences
Meanwhile, geneticists and molecular biologists have characterised five genes that appear to encode muscarinic receptors, named m1-m5 (lowercase m; no subscript number). The first four code for pharmacologic types M1-M4. The fifth, M5, corresponds to a subtype of receptor that had until recently not been detected pharmacologically. The receptors m1 and m2 were determined based upon partial sequencing of M1 and M2 receptor proteins. The others were found by searching for homology, using bioinformatic techniques.
Difference in G proteins
G proteins contain an alpha-subunit that is critical to the functioning of receptors. These subunits can take a number of forms. There are four broad classes of form of G-protein: Gs, Gi, Gq, and G12/13.[6] Muscarinic receptors vary in the G protein to which they are bound, with some correlation according to receptor type. G proteins are also classified according to their susceptibility to cholera toxin (CTX) and pertussis toxin (PTX, whooping cough). Gs and some subtypes of Gi (Gαt and Gαg) are susceptible to CTX. Only Gi is susceptible to PTX, with the exception of one subtype of Gi (Gαz) which is immune. Also, only when bound with an agonist, those G proteins normally sensitive to PTX also become susceptible to CTX.[7]
The various G-protein subunits act differently upon secondary messengers, upregulating Phospholipases, downregulating cAMP, and so on.
Because of the strong correlations to muscarinic receptor type, CTX and PTX are useful experimental tools in investigating these receptors.
Type | Gene | Function | PTX | CTX | Effectors | Agonists[8] | Antagonists[8] |
---|---|---|---|---|---|---|---|
M1 | CHRM1 |
|
no (yes) | no (yes) | Gq (Gi) (Gs): Slow EPSP. ↓ K+ conductance[11][14] |
| |
M2 | CHRM2 |
|
yes | no | Gi ↑ K+ conductance[11] ↓ Ca2+ conductance[11] | ||
M3 | CHRM3 |
|
no | no | Gq | ||
M4 | CHRM4 |
|
yes | ? | Gi ↑ K+ conductance[11] ↓ Ca2+ conductance[11] |
| |
M5 | CHRM5 |
|
no | ? | Gq |
M1 receptor
This receptor is found mediating slow EPSP at the ganglion in the postganglionic nerve, is common in exocrine glands and in the CNS.[21][22]
It is predominantly found bound to G proteins of class Gq,[23] which use upregulation of phospholipase C and, therefore, inositol trisphosphate and intracellular calcium as a signaling pathway. A receptor so bound would not be susceptible to CTX or PTX. However, Gi (causing a downstream decrease in cAMP) and Gs (causing an increase in cAMP) have also been shown to be involved in interactions in certain tissues, and so would be susceptible to PTX and CTX, respectively.
M2 receptor
The M2 muscarinic receptors are located in the heart, where they act to slow the heart rate down below the normal baseline sinus rhythm, by slowing the speed of depolarization. In humans under resting conditions vagal activity dominates over sympathetic activity. Hence inhibition of m2 receptors (e.g. by atropine) will cause a raise in heart rate. They also moderately reduce contractile forces of the atrial cardiac muscle, and reduce conduction velocity of the atrioventricular node (AV node). It also serves to slightly decrease the contractile forces of the ventricular muscle.
M2 muscarinic receptors act via a Gi type receptor, which causes a decrease in cAMP in the cell, inhibition of voltage-gated Ca2+ channels, and increasing efflux of K+, in general, leading to inhibitory-type effects.
M3 receptor
The M3 muscarinic receptors are located at many places in the body. They are located in the smooth muscles of the blood vessels, as well as in the lungs. Because the M3 receptor is Gq-coupled and mediates an increase in intracellular calcium, it typically causes contraction of smooth muscle, such as that observed during bronchoconstriction and bladder voiding.[24] However, with respect to vasculature, activation of M3 on vascular endothelial cells causes increased synthesis of nitric oxide, which diffuses to adjacent vascular smooth muscle cells and causes their relaxation, thereby explaining the paradoxical effect of parasympathomimetics on vascular tone and bronchiolar tone. Indeed, direct stimulation of vascular smooth muscle, M3 mediates vasconstriction in pathologies wherein the vascular endothelium is disrupted.[25] The M3 receptors are also located in many glands, which help to stimulate secretion in, for example, the salivary glands, as well as other glands of the body.
Like the M1 muscarinic receptor, M3 receptors are G proteins of class Gq that upregulate phospholipase C and, therefore, inositol trisphosphate and intracellular calcium as a signaling pathway.[4]
M4 receptor
M4 receptors are found in the CNS.
M4 receptors work via Gi receptors to decrease cAMP in the cell and, thus, produce generally inhibitory effects. Possible bronchospasm may result if stimulated by muscarinic agonists
M5 receptor
Location of M5 receptors is not well known.
Like the M1 and M3 muscarinic receptor, M5 receptors are coupled with G proteins of class Gq that upregulate phospholipase C and, therefore, inositol trisphosphate and intracellular calcium as a signaling pathway.
Pharmacological application
Ligands targeting the mAChR that are currently approved for clinical use include non-selective antagonists for the treatment of Parkinson's disease,[26] atropine (to dilate the pupil), scopolamine (used to prevent motion sickness), and ipratropium (used in the treatment of COPD).[3][27]
See also
References
- Eglen RM (July 2006). "Muscarinic receptor subtypes in neuronal and non-neuronal cholinergic function". Autonomic & Autacoid Pharmacology. 26 (3): 219–33. doi:10.1111/j.1474-8673.2006.00368.x. PMID 16879488.
- Ishii M, Kurachi Y (2006). "Muscarinic acetylcholine receptors". Current Pharmaceutical Design. 12 (28): 3573–81. doi:10.2174/138161206778522056. PMID 17073660. Archived from the original on 2009-02-05. Retrieved 2020-04-10.
- Purves, Dale, George J. Augustine, David Fitzpatrick, William C. Hall, Anthony-Samuel LaMantia, James O. McNamara, and Leonard E. White (2008). Neuroscience. 4th ed. Sinauer Associates. pp. 122–6. ISBN 978-0-87893-697-7.CS1 maint: multiple names: authors list (link)
- Qin K, Dong C, Wu G, Lambert NA (August 2011). "Inactive-state preassembly of G(q)-coupled receptors and G(q) heterotrimers". Nature Chemical Biology. 7 (10): 740–7. doi:10.1038/nchembio.642. PMC 3177959. PMID 21873996.
- Caulfield MP, Birdsall NJ (June 1998). "International Union of Pharmacology. XVII. Classification of muscarinic acetylcholine receptors". Pharmacological Reviews. 50 (2): 279–90. PMID 9647869.
- Simon MI, Strathmann MP, Gautam N (May 1991). "Diversity of G proteins in signal transduction". Science. 252 (5007): 802–8. Bibcode:1991Sci...252..802S. doi:10.1126/science.1902986. PMID 1902986.
- Dell'Acqua ML, Carroll RC, Peralta EG (March 1993). "Transfected m2 muscarinic acetylcholine receptors couple to G alpha i2 and G alpha i3 in Chinese hamster ovary cells. Activation and desensitization of the phospholipase C signaling pathway". The Journal of Biological Chemistry. 268 (8): 5676–85. PMID 8449930.
- Tripathi KD (2004). Essentials of Medical Pharmacology (5th ed.). India: Jaypee Brothers, Medical Publishers. pp. 890 pages. ISBN 978-81-8061-187-2. if nothing else mentioned in table
- Smith RS, Araneda RC (December 2010). "Cholinergic modulation of neuronal excitability in the accessory olfactory bulb". Journal of Neurophysiology. 104 (6): 2963–74. doi:10.1152/jn.00446.2010. PMC 3007668. PMID 20861438.
- Egorov AV, Hamam BN, Fransén E, Hasselmo ME, Alonso AA (November 2002). "Graded persistent activity in entorhinal cortex neurons". Nature. 420 (6912): 173–8. Bibcode:2002Natur.420..173E. doi:10.1038/nature01171. PMID 12432392.
- Rang HP, Dale MM, Ritter JM, Moore PK (2003). "Ch. 10". Pharmacology (5th ed.). Elsevier Churchill Livingstone. p. 139. ISBN 978-0-443-07145-4.
- Carlson, AB; Kraus, GP (2019), "article-19473", Physiology, Cholinergic Receptors, Treasure Island (FL): StatPearls Publishing, PMID 30252390, retrieved 7 January 2020
- Abrams P, Andersson KE, Buccafusco JJ, Chapple C, de Groat WC, Fryer AD, et al. (July 2006). "Muscarinic receptors: their distribution and function in body systems, and the implications for treating overactive bladder". British Journal of Pharmacology. Wiley. 148 (5): 565–78. doi:10.1038/sj.bjp.0706780. PMC 1751864. PMID 16751797.
- Uchimura N, North RA (March 1990). "Muscarine reduces inwardly rectifying potassium conductance in rat nucleus accumbens neurones". The Journal of Physiology. 422 (1): 369–80. doi:10.1113/jphysiol.1990.sp017989. PMC 1190137. PMID 1693682. Archived from the original on 2009-01-30. Retrieved 2008-02-25.
- Kitamura Y, Kaneda T, Nomura Y (January 1991). "Effects of nebracetam (WEB 1881 FU), a novel nootropic, as a M1-muscarinic agonist". Japanese Journal of Pharmacology. 55 (1): 177–80. doi:10.1254/jjp.55.177. PMID 2041225.
- Lameh J, Burstein ES, Taylor E, Weiner DM, Vanover KE, Bonhaus DW (August 2007). "Pharmacology of N-desmethylclozapine". Pharmacology & Therapeutics. 115 (2): 223–31. doi:10.1016/j.pharmthera.2007.05.004. PMID 17583355.
- Edwards Pharmaceuticals, Inc.; Belcher Pharmaceuticals, Inc. (May 2010). "DailyMed". U.S. National Library of Medicine. Retrieved January 13, 2013. Cite journal requires
|journal=
(help) - Servent D, Blanchet G, Mourier G, Marquer C, Marcon E, Fruchart-Gaillard C (November 2011). "Muscarinic toxins". Toxicon. 58 (6–7): 455–63. doi:10.1016/j.toxicon.2011.08.004. PMID 21906611.
- Karlsson E, Jolkkonen M, Mulugeta E, Onali P, Adem A (September 2000). "Snake toxins with high selectivity for subtypes of muscarinic acetylcholine receptors". Biochimie. 82 (9–10): 793–806. doi:10.1016/S0300-9084(00)01176-7. PMID 11086210.
- Melchiorre C, Angeli P, Lambrecht G, Mutschler E, Picchio MT, Wess J (December 1987). "Antimuscarinic action of methoctramine, a new cardioselective M-2 muscarinic receptor antagonist, alone and in combination with atropine and gallamine". European Journal of Pharmacology. 144 (2): 117–24. doi:10.1016/0014-2999(87)90509-7. PMID 3436364.
- Johnson, Gordon (2002). PDQ Pharmacology (2nd ed.). Hamilton, Ontario: BC Decker Inc. pp. 311 pages. ISBN 978-1-55009-109-0.
- Richelson, Elliott (2000). "Cholinergic Transduction, Psychopharmacology - The Fourth Generation of Progress". American College of Neuropsychopharmacology. Retrieved 2007-10-27.
- Burford NT, Nahorski SR (May 1996). "Muscarinic m1 receptor-stimulated adenylate cyclase activity in Chinese hamster ovary cells is mediated by Gs alpha and is not a consequence of phosphoinositidase C activation". The Biochemical Journal. 315 (3): 883–8. doi:10.1042/bj3150883. PMC 1217289. PMID 8645172.
- Moro C, Uchiyama J, Chess-Williams R (December 2011). "Urothelial/lamina propria spontaneous activity and the role of M3 muscarinic receptors in mediating rate responses to stretch and carbachol". Urology. 78 (6): 1442.e9–15. doi:10.1016/j.urology.2011.08.039. PMID 22001099.
- Keith Parker; Laurence Brunton; Goodman, Louis Sanford; Lazo, John S.; Gilman, Alfred (2006). Goodman & Gilman's the pharmacological basis of therapeutics (11th ed.). New York: McGraw-Hill. pp. 185. ISBN 978-0-07-142280-2.
- Langmead CJ, Watson J, Reavill C (February 2008). "Muscarinic acetylcholine receptors as CNS drug targets". Pharmacology & Therapeutics. 117 (2): 232–43. doi:10.1016/j.pharmthera.2007.09.009. PMID 18082893.
- Matera C, Tata AM (2014). "Pharmacological approaches to targeting muscarinic acetylcholine receptors". Recent Patents on CNS Drug Discovery. 9 (2): 85–100. doi:10.2174/1574889809666141120131238. PMID 25413004.
External links
- "Acetylcholine Receptors (Muscarinic)". IUPHAR Database of Receptors and Ion Channels. International Union of Basic and Clinical Pharmacology.
- Receptors,+Muscarinic at the US National Library of Medicine Medical Subject Headings (MeSH)