Hybrid (biology)
In biology, a hybrid is the offspring resulting from combining the qualities of two organisms of different breeds, varieties, species or genera through sexual reproduction. Hybrids are not always intermediates between their parents (such as in blending inheritance), but can show hybrid vigour, sometimes growing larger or taller than either parent. The concept of a hybrid is interpreted differently in animal and plant breeding, where there is interest in the individual parentage. In genetics, attention is focused on the numbers of chromosomes. In taxonomy, a key question is how closely related the parent species are.
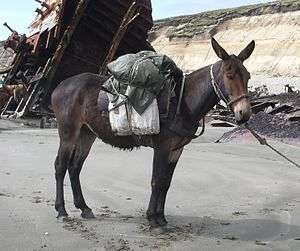
Species are reproductively isolated by strong barriers to hybridisation, which include genetic and morphological differences, differing times of fertility, mating behaviors and cues, and physiological rejection of sperm cells or the developing embryo. Some act before fertilization and others after it. Similar barriers exist in plants, with differences in flowering times, pollen vectors, inhibition of pollen tube growth, somatoplastic sterility, cytoplasmic-genic male sterility and the structure of the chromosomes. A few animal species and many plant species, however, are the result of hybrid speciation, including important crop plants such as wheat, where the number of chromosomes has been doubled.
Human impact on the environment has resulted in an increase in the interbreeding between regional species, and the proliferation of introduced species worldwide has also resulted in an increase in hybridisation. This genetic mixing may threaten many species with extinction, while genetic erosion in crop plants may be damaging the gene pools of many species for future breeding. A form of often intentional human-mediated hybridisation is the crossing of wild and domesticated species. This is common in both traditional horticulture and modern agriculture; many commercially useful fruits, flowers, garden herbs, and trees have been produced by hybridisation. One such flower, Oenothera lamarckiana, was central to early genetics research into mutationism and polyploidy. It is also more occasionally done in the livestock and pet trades; some well-known wild × domestic hybrids are beefalo and wolfdogs. Human selective breeding of domesticated animals and plants has resulted is the development of distinct breeds (usually called cultivars in reference to plants); crossbreeds between them (without any wild stock) are sometimes also imprecisely referred to as "hybrids".
Hybrid humans existed in prehistory. For example, Neanderthals and anatomically modern humans are thought to have interbred as recently as 40,000 years ago.
Mythological hybrids appear in human culture in forms as diverse as the Minotaur, blends of animals, humans and mythical beasts such as centaurs and sphinxes, and the Nephilim of the Biblical apocrypha described as the wicked sons of fallen angels and attractive women.
Etymology
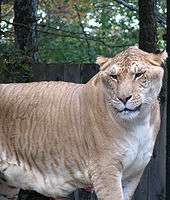
The term hybrid is derived from Latin hybrida, used for crosses such as of a tame sow and a wild boar. The term came into popular use in English in the 19th century, though examples of its use have been found from the early 17th century.[1] Conspicuous hybrids are popularly named with portmanteau words, starting in the 1920s with the breeding of tiger–lion hybrids (liger and tigon).[2]
As seen by different disciplines
Animal and plant breeding
From the point of view of animal and plant breeders, there are several kinds of hybrid formed from crosses within a species, such as between different breeds.[3] Single cross hybrids result from the cross between two true-breeding organisms which produces an F1 hybrid (first filial generation). The cross between two different homozygous lines produces an F1 hybrid that is heterozygous; having two alleles, one contributed by each parent and typically one is dominant and the other recessive. Typically, the F1 generation is also phenotypically homogeneous, producing offspring that are all similar to each other.[4] Double cross hybrids result from the cross between two different F1 hybrids (i.e., there are four unrelated grandparents).[5] Three-way cross hybrids result from the cross between an F1 hybrid and an inbred line. Triple cross hybrids result from the crossing of two different three-way cross hybrids.[6] Top cross (or "topcross") hybrids result from the crossing of a top quality or pure-bred male and a lower quality female, intended to improve the quality of the offspring, on average.[7]
Population hybrids result from the crossing of plants or animals in one population with those of another population. These include interspecific hybrids or crosses between different breeds.[8]
In horticulture, the term stable hybrid is used to describe an annual plant that, if grown and bred in a small monoculture free of external pollen (e.g., an air-filtered greenhouse) produces offspring that are "true to type" with respect to phenotype; i.e., a true-breeding organism.[9]
Biogeography
Hybridisation can occur in the hybrid zones where the geographical ranges of species, subspecies, or distinct genetic lineages overlap. For example, the butterfly Limenitis arthemis has two major subspecies in North America, L. a. arthemis (the white admiral) and L. a. astyanax (the red-spotted purple). The white admiral has a bright, white band on its wings, while the red-spotted purple has cooler blue-green shades. Hybridisation occurs between a narrow area across New England, southern Ontario, and the Great Lakes, the "suture region". It is at these regions that the subspecies were formed.[10] Other hybrid zones have formed between described species of plants and animals.
Genetics
_(7164908226).jpg)
From the point of view of genetics, several different kinds of hybrid can be distinguished.[11][12] A genetic hybrid carries two different alleles of the same gene, where for instance one allele may code for a lighter coat colour than the other.[11][12] A structural hybrid results from the fusion of gametes that have differing structure in at least one chromosome, as a result of structural abnormalities.[11][12] A numerical hybrid results from the fusion of gametes having different haploid numbers of chromosomes.[11][12] A permanent hybrid results when only the heterozygous genotype occurs, as in Oenothera lamarckiana,[13] because all homozygous combinations are lethal.[11][12] In the early history of genetics, Hugo de Vries supposed these were caused by mutation.[14][15]
Taxonomy
From the point of view of taxonomy, hybrids differ according to their parentage. Hybrids between different subspecies (such as between the Dog and Eurasian wolf) are called intra-specific hybrids.[16] Interspecific hybrids are the offspring from interspecies mating;[17] these sometimes result in hybrid speciation.[18] Intergeneric hybrids result from matings between different genera, such as between sheep and goats.[19] Interfamilial hybrids, such as between chickens and guineafowl or pheasants, are reliably described but extremely rare.[20] Interordinal hybrids (between different orders) are few, but have been made with the sea urchin Strongylocentrotus purpuratus (female) and the sand dollar Dendraster excentricus (male).[21]
Biology
Expression of parental traits
.jpg)
When two distinct types of organisms breed with each other, the resulting hybrids typically have intermediate traits (e.g., one plant parent has red flowers, the other has white, and the hybrid, pink flowers).[22] Commonly, hybrids also combine traits seen only separately in one parent or the other (e.g., a bird hybrid might combine the yellow head of one parent with the orange belly of the other).[22]
Mechanisms of reproductive isolation
Interspecific hybrids are bred by mating individuals from two species, normally from within the same genus. The offspring display traits and characteristics of both parents, but are often sterile, preventing gene flow between the species.[23] Sterility is often attributed to the different number of chromosomes between the two species. For example, donkeys have 62 chromosomes, horses have 64 chromosomes, and mules or hinnies have 63 chromosomes. Mules, hinnies, and other normally sterile interspecific hybrids cannot produce viable gametes, because differences in chromosome structure prevent appropriate pairing and segregation during meiosis, meiosis is disrupted, and viable sperm and eggs are not formed. However, fertility in female mules has been reported with a donkey as the father.[24]
A variety of mechanisms limit the success of hybridisation, including the large genetic difference between most species. Barriers include morphological differences, differing times of fertility, mating behaviors and cues, and physiological rejection of sperm cells or the developing embryo. Some act before fertilization; others after it.[25][26][27][28]
In plants, some barriers to hybridisation include blooming period differences, different pollinator vectors, inhibition of pollen tube growth, somatoplastic sterility, cytoplasmic-genic male sterility and structural differences of the chromosomes.[29]
Speciation
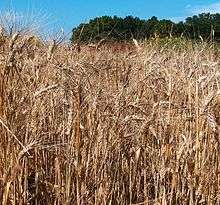
A few animal species are the result of hybridization. The Lonicera fly is a natural hybrid. The American red wolf appears to be a hybrid of the gray wolf and the coyote,[31] although its taxonomic status has been a subject of controversy.[32][33][34] The European edible frog is a semi-permanent hybrid between pool frogs and marsh frogs; its population requires the continued presence of at least one of the parent species.[35] Cave paintings indicate that the European bison is a natural hybrid of the aurochs and the steppe bison.[36][37]
Plant hybridization is more commonplace compared to animal hybridization. Many crop species are hybrids, including notably the polyploid wheats: some have four sets of chromosomes (tetraploid) or six (hexaploid), while other wheat species have (like most eukaryotic organisms) two sets (diploid), so hybridization events likely involved the doubling of chromosome sets, causing immediate genetic isolation.[38]
Hybridization may be important in speciation in some plant groups. However, homoploid hybrid speciation (not increasing the number of sets of chromosomes) may be rare: by 1997, only 8 natural examples had been fully described. Experimental studies suggest that hybridization offers a rapid route to speciation, a prediction confirmed by the fact that early generation hybrids and ancient hybrid species have matching genomes, meaning that once hybridization has occurred, the new hybrid genome can remain stable.[39]
Many hybrid zones are known where the ranges of two species meet, and hybrids are continually produced in great numbers. These hybrid zones are useful as biological model systems for studying the mechanisms of speciation. Recently DNA analysis of a bear shot by a hunter in the North West Territories confirmed the existence of naturally-occurring and fertile grizzly–polar bear hybrids.[40]
Hybrid vigour
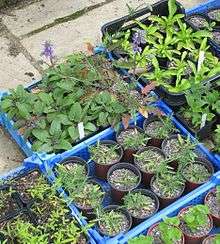
Hybridization between reproductively isolated species often results in hybrid offspring with lower fitness than either parental. However, hybrids are not, as might be expected, always intermediate between their parents (as if there were blending inheritance), but are sometimes stronger or perform better than either parental lineage or variety, a phenomenon called heterosis, hybrid vigour, or heterozygote advantage. This is most common with plant hybrids.[41] A transgressive phenotype is a phenotype that displays more extreme characteristics than either of the parent lines.[42] Plant breeders use several techniques to produce hybrids, including line breeding and the formation of complex hybrids. An economically important example is hybrid maize (corn), which provides a considerable seed yield advantage over open pollinated varieties. Hybrid seed dominates the commercial maize seed market in the United States, Canada and many other major maize-producing countries.[43]
In a hybrid, any trait that falls outside the range of parental variation (and is thus not simply intermediate between its parents) is considered heterotic. Positive heterosis produces more robust hybrids, they might be stronger or bigger; while the term negative heterosis refers to weaker or smaller hybrids.[44] Heterosis is common in both animal and plant hybrids. For example, hybrids between a lion and a tigress ("ligers") are much larger than either of the two progenitors, while "tigons" (lioness × tiger) are smaller. Similarly, the hybrids between the common pheasant (Phasianus colchicus) and domestic fowl (Gallus gallus) are larger than either of their parents, as are those produced between the common pheasant and hen golden pheasant (Chrysolophus pictus).[45] Spurs are absent in hybrids of the former type, although present in both parents.[46]
Human influence
Anthropogenic hybridization
Hybridization is greatly influenced by human impact on the environment,[47] through effects such as habitat fragmentation and species introductions.[48] Such impacts make it difficult to conserve the genetics of populations undergoing introgressive hybridization. Humans have introduced species worldwide to environments for a long time, both intentionally for purposes such as biological control, and unintentionally, as with accidental escapes of individuals. Introductions can drastically affect populations, including through hybridization.[12][49]
Management
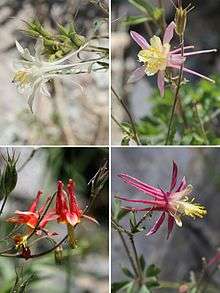
There is a kind of continuum with three semi-distinct categories dealing with anthropogenic hybridization: hybridization without introgression, hybridization with widespread introgression (backcrossing with one of the parent species), and hybrid swarms (highly variable populations with much interbreeding as well as backcrossing with the parent species). Depending on where a population falls along this continuum, the management plans for that population will change. Hybridization is currently an area of great discussion within wildlife management and habitat management. Global climate change is creating other changes such as difference in population distributions which are indirect causes for an increase in anthropogenic hybridization.[47]
Conservationists disagree on when is the proper time to give up on a population that is becoming a hybrid swarm, or to try and save the still existing pure individuals. Once a population becomes a complete mixture, the goal becomes to conserve those hybrids to avoid their loss. Conservationists treat each case on its merits, depending on detecting hybrids within the population. It is nearly impossible to formulate a uniform hybridization policy, because hybridization can occur beneficially when it occurs "naturally", and when hybrid swarms are the only remaining evidence of prior species, they need to be conserved as well.[47]
Genetic mixing and extinction
Regionally developed ecotypes can be threatened with extinction when new alleles or genes are introduced that alter that ecotype. This is sometimes called genetic mixing.[50] Hybridization and introgression, which can happen in natural and hybrid populations, of new genetic material can lead to the replacement of local genotypes if the hybrids are more fit and have breeding advantages over the indigenous ecotype or species. These hybridization events can result from the introduction of non-native genotypes by humans or through habitat modification, bringing previously isolated species into contact. Genetic mixing can be especially detrimental for rare species in isolated habitats, ultimately affecting the population to such a degree that none of the originally genetically distinct population remains.[51][52]
Effect on biodiversity and food security
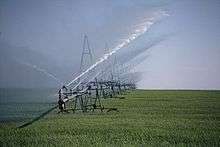
In agriculture and animal husbandry, the Green Revolution's use of conventional hybridization increased yields by breeding "high-yielding varieties". The replacement of locally indigenous breeds, compounded with unintentional cross-pollination and crossbreeding (genetic mixing), has reduced the gene pools of various wild and indigenous breeds resulting in the loss of genetic diversity.[54] Since the indigenous breeds are often well-adapted to local extremes in climate and have immunity to local pathogens, this can be a significant genetic erosion of the gene pool for future breeding. Therefore, commercial plant geneticists strive to breed "widely adapted" cultivars to counteract this tendency.[55]
In different taxa
In animals
Mammals
Familiar examples of equid hybrids are the mule, a cross between a female horse and a male donkey, and the hinny, a cross between a female donkey and a male horse. Pairs of complementary types like the mule and hinny are called reciprocal hybrids.[56] Among many other mammal crosses are hybrid camels, crosses between a bactrian camel and a dromedary.[57] There are many examples of felid hybrids, including the liger.
The first known instance of hybrid speciation in marine mammals was discovered in 2014. The clymene dolphin (Stenella clymene) is a hybrid of two Atlantic species, the spinner and striped dolphins.[58] In 2019, scientists confirmed that a skull found 30 years earlier was a hybrid between the beluga whale and narwhal; dubbed the narluga.[59]
Birds
Cagebird breeders sometimes breed bird hybrids known as mules between species of finch, such as goldfinch × canary.[60]
Amphibians
Among amphibians, Japanese giant salamanders and Chinese giant salamanders have created hybrids that threaten the survival of Japanese giant salamanders because of competition for similar resources in Japan.[61]
Fish
Among fish, a group of about fifty natural hybrids between Australian blacktip shark and the larger common blacktip shark was found by Australia's eastern coast in 2012.[62]
Russian sturgeon and American paddlefish were hybridized in captivity when sperm from the paddlefish and eggs from the sturgeon were combined, unexpectedly resulting in viable offspring. This hybrid is called a sturddlefish.[63][64]
Invertebrates
Among insects, so-called killer bees were accidentally created during an attempt to breed a strain of bees that would both produce more honey and be better adapted to tropical conditions. It was done by crossing a European honey bee and an African bee.[65]
The Colias eurytheme and C. philodice butterflies have retained enough genetic compatibility to produce viable hybrid offspring.[66] Hybrid speciation may have produced the diverse Heliconius butterflies,[67] but that is disputed.[68]
In plants
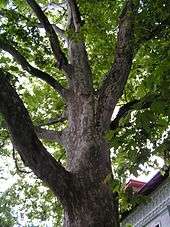
Plant species hybridize more readily than animal species, and the resulting hybrids are fertile more often. Many plant species are the result of hybridization, combined with polyploidy, which duplicates the chromosomes. Chromosome duplication allows orderly meiosis and so viable seed can be produced.[69]
Plant hybrids are generally given names that include an "×" (not in italics), such as Platanus × acerifolia for the London plane, a natural hybrid of P. orientalis (oriental plane) and P. occidentalis (American sycamore).[70][71] The parent's names may be kept in their entirety, as seen in Prunus persica × Prunus americana, with the female parent's name given first, or if not known, the parent's names given alphabetically.[72]
Plant species that are genetically compatible may not hybridize in nature for various reasons, including geographical isolation, differences in flowering period, or differences in pollinators. Species that are brought together by humans in gardens may hybridize naturally, or hybridization can be facilitated by human efforts, such as altered flowering period or artificial pollination. Hybrids are sometimes created by humans to produce improved plants that have some of the characteristics of each of the parent species. Much work is now being done with hybrids between crops and their wild relatives to improve disease-resistance or climate resilience for both agricultural and horticultural crops.[73]
Some crop plants are hybrids from different genera (intergeneric hybrids), such as Triticale, × Triticosecale, a wheat–rye hybrid.[74] Most modern and ancient wheat breeds are themselves hybrids; bread wheat, Triticum aestivum, is a hexaploid hybrid of three wild grasses.[30] Several commercial fruits including loganberry (Rubus × loganobaccus)[75] and grapefruit (Citrus × paradisi)[76] are hybrids, as are garden herbs such as peppermint (Mentha × piperita),[77] and trees such as the London plane (Platanus × acerifolia).[78][79] Among many natural plant hybrids is Iris albicans, a sterile hybrid that spreads by rhizome division,[80] and Oenothera lamarckiana, a flower that was the subject of important experiments by Hugo de Vries that produced an understanding of polyploidy.[13]
- A sterile hybrid between Trillium cernuum and T. grandiflorum
- An ornamental lily hybrid known as Lilium 'Citronella'[81]
Sterility in a non-polyploid hybrid is often a result of chromosome number; if parents are of differing chromosome pair number, the offspring will have an odd number of chromosomes, which leaves them unable to produce chromosomally-balanced gametes.[82] While that is undesirable in a crop such as wheat, for which growing a crop that produces no seeds would be pointless, it is an attractive attribute in some fruits. Triploid bananas and watermelons are intentionally bred because they produce no seeds and are also parthenocarpic.[83]
In humans
There is evidence of hybridisation between modern humans and other species of the genus Homo. In 2010, the Neanderthal genome project showed that 1–4% of DNA from all people living today, apart from most Sub-Saharan Africans, is of Neanderthal heritage. Analyzing the genomes of 600 Europeans and East Asians found that combining them covered 20% of the Neanderthal genome that is in the modern human population.[84] Ancient human populations lived and interbred with Neanderthals, Denisovans, and at least one other extinct Homo species.[85] Thus, Neanderthal and Denisovan DNA has been incorporated into human DNA by introgression.[86]
In 1998, a complete prehistorical skeleton found in Portugal, the Lapedo child, had features of both anatomically modern humans and Neanderthals.[87] Some ancient human skulls with especially large nasal cavities and unusually shaped braincases represent human-Neanderthal hybrids. A 37,000- to 42,000-year-old human jawbone found in Romania's Oase cave contains traces of Neanderthal ancestry[lower-alpha 1] from only four to six generations earlier.[89] All genes from Neanderthals in the current human population are descended from Neanderthal fathers and human mothers.[90] A Neanderthal skull unearthed in Italy in 1957 reveals Neanderthal mitochondrial DNA, which is passed on through only the maternal lineage, but the skull has a chin shape similar to modern humans. It is proposed that it was the offspring of a Neanderthal mother and a human father.[91]
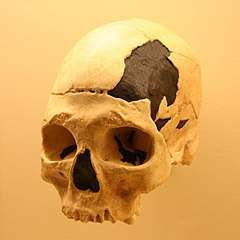
In mythology
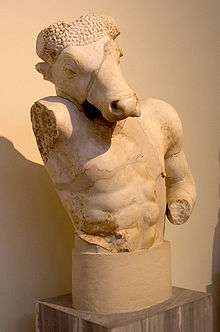
Folk tales and myths sometimes contain mythological hybrids; the Minotaur was the offspring of a human, Pasiphaë, and a white bull.[92] More often, they are composites of the physical attributes of two or more kinds of animals, mythical beasts, and humans, with no suggestion that they are the result of interbreeding, as in the centaur (man/horse), chimera (goat/lion/snake), hippocamp (fish/horse), and sphinx (woman/lion).[93] The Old Testament mentions a first generation of half-human hybrid giants, the Nephilim,[94][95] while the apocryphal Book of Enoch describes the Nephilim as the wicked sons of fallen angels and attractive women.[96]
Human-animal hybrids portray humanity's conflicted or divided nature.[97] For example, the centaur, which has the face, arms, and trunk of a human with the body of a horse, depicts the unbridled tendencies within humans. The sphinx, which in its Greek form has the face of a woman and the body of a lion with wings, represents the cunning, trickster side of humans. Sophocles' Oedipus narratives highlight this trait when the sphinx of Thebes poses a riddle that appears unsolvable but is solved by Oedipus. The manticore has the head of a human with three rows of shark-like teeth, a body of a lion, and a tail with venomous spines similar to porcupine quills or a scorpion-like tail. The manticore portrays the bestial and vicious nature of humans. In the Book of Revelation, hybrids portray what this world would look like if evil gained the upper hand and reigned uncontested. Specifically, the locusts of Revelation 9 are human-animal hybrids released from the abyss (the demonic underworld) and represent the reverse side of this world. Their destructive nature portrays what this world would be like if God allowed demonic powers to have free, unrestrained reign.[98]
See also
- Canid hybrid
- Chimera (genetics)
- Chloroplast capture (botany)
- Eukaryote hybrid genome
- Felid hybrids
- Genetic admixture
- Genetic erosion
- Grex (horticulture)
- Hybridogenesis
- Hybrot
- Inbreeding
- Interspecific pregnancy
- List of plant hybrids
- List of genetic hybrids
- Macropod hybrids
- Purebred
- Selective breeding
Notes
- Signs of Neanderthal lineage include a wide jaw and large teeth that get bigger toward the back of the mouth.[88]
References
- "Hybrid". Online Etymology Dictionary. Retrieved 28 June 2017.
- "When the sire is a lion the result is termed a Liger, whilst the converse is a Tigon." Edward George Boulenger, World natural history, B. T. Batsford ltd., 1937, p. 40
- Wricke, Gunter; Weber, Eberhard (1986). Quantitative genetics and selection in plant breeding. W. de Gruyter. p. 257.
- Runge, Marschall S.; Patterson, Cam, eds. (2006). Principles of Molecular Medicine. Humana Press. p. 58. ISBN 978-1-58829-202-5.
- Rawlings, J. O.; Cockerham, C. Clark (June 1962). "Analysis of Double Cross Hybrid Populations". Biometrics. 18 (2): 229–244. doi:10.2307/2527461. JSTOR 2527461.
- Thompson, K. F. (1964). "Triple-cross hybrid kale". Euphytica. 13: 173. doi:10.1007/BF00033306 (inactive 29 April 2020).
- "Topcross". Merriam-Webster. Retrieved 20 March 2017.
- McCarthy, Eugene M. "Hybrid Populations". Macroevolution. Retrieved 20 March 2017.
- Toogood, A., ed. (1999). Plant Propagation. American Horticultural Society. p. 21. ISBN 978-0-7894-5520-8.
- Ries, Leslie; Mullen, Sean P. (2008). "A Rare Model Limits the Distribution of Its More Common Mimic: A Twist on Frequency-Dependent Batesian Mimicry". Evolution. 62 (7): 1798–803. doi:10.1111/j.1558-5646.2008.00401.x. PMID 18410533.
- Rieger, R.; Michaelis A.; Green, M. M. (1991). Glossary of Genetics: Classical and Molecular (5th ed.). Springer-Verlag. p. 256. ISBN 978-0-387-52054-4.
- Allendorf, Fred (2007). Conservation and the Genetics of Populations. Blackwell. p. 534.
- Sirks, M. J. (2013). General Genetics. Springer. p. 408. ISBN 978-94-015-7587-4.
- De Vries, Hugo (1901–1903). Die mutationstheorie. Vol I and II. Von Veit, Leipzig.
- de Vries, Hugo (January 1919). "Oenothera rubrinervis; A Half Mutant". Botanical Gazette. 67 (1): 1–26. doi:10.1086/332396. JSTOR 2468868.
- Allendorf, Fred (2007). Conservation and the Genetics of Populations. Blackwell. pp. 421–448.
- Keeton, William T. (1980). Biological Science. Norton. p. A9. ISBN 978-0-393-95021-2.
- Arnold, M.L. (1996). Natural Hybridisation and Evolution. Oxford University Press. p. 232. ISBN 978-0-19-509975-1.
- Kelk, Dawn A.; Gartley, Cathy J.; Buckrell, Brian C.; King, W. Allan (1997). "The interbreeding of sheep and goats". Canadian Veterinary Journal. 38 (4): 235–237. PMC 1576582. PMID 9105723.
- Johnsgard, Paul A. (1983). "Hybridisation & Zoogeographic Patterns in Pheasants". University of Nebraska - Lincoln. p. 5. Retrieved 20 March 2017.
- Giudice, Giovanni (2012). Developmental Biology of the Sea Urchin Embryo. Elsevier. p. 171. ISBN 978-0-323-14878-8.
- McCarthy, Eugene M. (2006). Handbook of Avian Hybrids of the World. Oxford University Press. pp. 16–17.
- Keeton, William T. 1980. Biological science. New York: Norton. ISBN 0-393-95021-2, p. 800
- Rong, R.; Chandley, A. C.; Song, J.; McBeath, S.; Tan, P. P.; Bai, Q.; Speed, R. M. (1988). "A fertile mule and hinny in China". Cytogenetics and Cell Genetics. 47 (3): 134–9. doi:10.1159/000132531. PMID 3378453.
- Baker, H. G. (1959). "Reproductive methods as factors in speciation in flowering plants". Cold Spring Harb Symp Quant Biol. 24: 177–191. doi:10.1101/sqb.1959.024.01.019. PMID 13796002.
- Barton N.; Bengtsson B. O. (1986), "The barrier to genetic exchange between hybridising populations", Heredity, 57 (3): 357–376, doi:10.1038/hdy.1986.135, PMID 3804765
- Strickberger, M. 1978. Genética. Omega, Barcelona, España, p.: 874–879. ISBN 84-282-0369-5.
- Futuyma, D. 1998. Evolutionary biology (3ª edición). Sinauer, Sunderland.
- Hermsen, J. G. Th.; Ramanna, M. S. (January 1976), "Barriers to hybridisation of Solanum bulbocastanumDun. and S. VerrucosumSchlechtd. and structural hybridity in their F1 plants", Euphytica, 25 (1): 1–10, doi:10.1007/BF00041523
- Gornicki, Piotr; et al. (2014). "The chloroplast view of the evolution of polyploid wheat". New Phytologist. 204 (3): 704–714. doi:10.1111/nph.12931. PMID 25059383.
- Esch, Mary (31 May 2011). "Study: Eastern wolves are hybrids with coyotes". Boston.com. Associated Press. Retrieved 22 March 2017.
- Rutledge, Linda Y.; et al. (2012). "Conservation genomics in perspective: A holistic approach to understanding Canis evolution in North America" (PDF). Biological Conservation. 155: 186–192. doi:10.1016/j.biocon.2012.05.017.
- Chambers, Steven M.; et al. (2012). "An account of the taxonomy of North American wolves from morphological and genetic analyses". North American Fauna. 77: 1–67. doi:10.3996/nafa.77.0001.
- Dumbacher, J., Review of Proposed Rule Regarding Status of the Wolf Under the Endangered Species Act, NCEAS (January 2014)
- Frost, Grant; et al. (15 March 2006). "The amphibian tree of life". Bulletin of the American Museum of Natural History. 297 (297): 1–291. doi:10.1206/0003-0090(2006)297[0001:TATOL]2.0.CO;2.
- Soubrier, Julien; et al. (18 October 2016). "Early cave art and ancient DNA record the origin of European bison". Nature Communications. 7: 13158. Bibcode:2016NatCo...713158S. doi:10.1038/ncomms13158. PMC 5071849. PMID 27754477.
- Daley, Jason. "Cave Paintings Help Unravel the Mystery of the 'Higgs Bison'". Smithsonian. Retrieved 20 October 2016.
- Hancock, James F. (2004). Plant Evolution and the Origin of Crop Species. CABI Publishing. ISBN 978-0-85199-685-1.
- Rieseberg, Loren H. (1997). "Hybrid Origins of Plant Species". Annual Review of Ecology, Evolution, and Systematics. 28: 359–389. CiteSeerX 10.1.1.718.9871. doi:10.1146/annurev.ecolsys.28.1.359.
- "Hybrid bear shot dead in Canada". BBC News. 13 May 2006.
- Stokes, David (July 2007), "Evaluating the utility of Arabidopsis thaliana as a model for understanding heterosis in hybrid crops", Euphytica, 156 (1–2): 157–171, doi:10.1007/s10681-007-9362-1
- Rieseberg, Loren H.; Margaret A. Archer; Robert K. Wayne (July 1999). "Transgressive segregation, adaptation and speciation". Heredity. 83 (4): 363–372. doi:10.1038/sj.hdy.6886170. PMID 10583537.
- Smith, C. Wayne (2004). Corn: Origin, History, Technology, and Production. Wiley. p. 332.
- McCarthy, Eugene M. (2006). Handbook of Avian Hybrids of the World. Oxford University Press. p. 17. ISBN 978-0-19-518323-8.
- Darwin, Charles (1868). Variation of Animals and Plants under Domestication. II. p. 125.
- Spicer, J. W. G. (1854). "Note on hybrid gallinaceous birds". The Zoologist. 12: 4294–4296.
- Allendorf, Fred W.; Leary, R. F.; Spruell, P.; Wenburg, J. K. (2001). "The problems with hybrids: setting conservation guidelines". Trends in Ecology & Evolution. 16 (11): 613–622. doi:10.1016/S0169-5347(01)02290-X.
- Ehrlich, Paul; John Holdren (26 March 1971). "Impact of population Growth". Science. 171 (3977): 1212–1216. Bibcode:1971Sci...171.1212E. doi:10.1126/science.171.3977.1212. PMID 5545198.
- Vitousek, Peter; et al. (1997). "Introduced Species: A Significant Component of Human-cause Global Change". New Zealand Journal of Ecology. 21 (1): 1–16.
- Mooney, H. A.; Cleland, E. E. (2001). "The evolutionary impact of invasive species". PNAS. 98 (10): 5446–5451. Bibcode:2001PNAS...98.5446M. doi:10.1073/pnas.091093398. PMC 33232. PMID 11344292.
- Rhymer, J. M.; Simberloff, D. (1996). "Extinction by Hybridization and Introgression". Annual Review of Ecology and Systematics. 27: 83–109. doi:10.1146/annurev.ecolsys.27.1.83.
- Potts, Brad M.; Barbour, Robert C.; Hingston, Andrew B. (2001) Genetic Pollution from Farm Forestry using eucalypt species and hybrids Archived 2 January 2004 at the Wayback Machine; A report for the RIRDC/L&WA/FWPRDC; Joint Venture Agroforestry Program; RIRDC Publication No 01/114; RIRDC Project No CPF - 3A; ISBN 0-642-58336-6; ISSN 1440-6845; Australian Government, Rural Industrial Research and Development Corporation
- Farmer, B. H. (1986). "Perspectives on the 'Green Revolution' in South Asia". Modern Asian Studies. 20 (1): 175–199. doi:10.1017/s0026749x00013627.
- "Genetic Pollution: The Great Genetic Scandal" Devinder Sharma Archived 18 May 2009 at the Wayback Machine; Bulletin 28
- Troyer, A. Forrest. Breeding Widely Adapted Cultivars: Examples from Maize. Encyclopedia of Plant and Crop Science, 27 February 2004.
- Griesbach, Robert J. (1986). "That Reciprocal Cross — Is It a Mule or Hinny?" (PDF). Awards Quarterly. 17 (3): 149.
- Bulliet, R.W. (1975). The Camel and the Wheel. Columbia University Press. pp. 164–175.
- Bhanoo, Sindya (13 January 2014). "Scientists Find Rare Hybrid of Two Other Dolphin Species". The New York Times. Retrieved 20 January 2014.
- Kovrind, Mikkel; Eight Others. "Hybridization between two high Arctic cetaceans confirmed by genomic analysis".
- "British Mule/Hybrid". Severn Counties Foreign & British Bird Society. Retrieved 19 March 2017.
- "Godzilla vs. Godzilla—How the Chinese Giant Salamander is taking a toll on its Japanese Comic Counterpart". Amphibians.org. Archived from the original on 30 June 2017. Retrieved 12 March 2017.
- Voloder, Dubravka (3 January 1012). "Print Email Facebook Twitter More World-first hybrid sharks found off Australia". ABC News. Retrieved 5 January 2012.
- Roth, Annie (15 July 2020). "Scientists Accidentally Bred the Fish Version of a Liger". The New York Times. ISSN 0362-4331. Retrieved 16 July 2020.
- Káldy, Jenő; Mozsár, Attila; Fazekas, Gyöngyvér; Farkas, Móni; Fazekas, Dorottya Lilla; Fazekas, Georgina Lea; Goda, Katalin; Gyöngy, Zsuzsanna; Kovács, Balázs; Semmens, Kenneth; Bercsényi, Miklós (6 July 2020). "Hybridization of Russian Sturgeon (Acipenser gueldenstaedtii, Brandt and Ratzeberg, 1833) and American Paddlefish (Polyodon spathula, Walbaum 1792) and Evaluation of Their Progeny". Genes. 11 (7): 753. doi:10.3390/genes11070753. ISSN 2073-4425.
- Hall, H. Glenn; Zettel-Nalen, Catherine; Ellis, James D. "African Honey Bee: What You Need to Know". University of Florida IFAS Extension. Retrieved 19 March 2017.
- Grula, John W.; Taylor, Orley R. (1980). "The Effect of X-Chromosome Inheritance on Mate-Selection Behavior in the Sulfur Butterflies, Colias eurytheme and C. Philodice". Evolution. 34 (4): 688–95. doi:10.2307/2408022. JSTOR 2408022.
- Mallet, J.; Beltrán, M.; Neukirchen, W.; Linares, M. (2007). "Natural hybridization in heliconiine butterflies: The species boundary as a continuum". BMC Evolutionary Biology. 7: 28. doi:10.1186/1471-2148-7-28. PMC 1821009. PMID 17319954.
- Brower, A.V.Z. (2011). "Hybrid speciation in Heliconius butterflies? A review and critique of the evidence". Genetica. 139 (2): 589–609. doi:10.1007/s10709-010-9530-4. PMC 3089819. PMID 21113790.
- Goulet, Benjamin E.; Roda, Federico; Hopkins, Robin (2016). "Hybridization in Plants: Old Ideas, New Techniques". Plant Physiology. 173 (1): 65–78. doi:10.1104/pp.16.01340. PMC 5210733. PMID 27895205.
- McNeill, J.; et al. (Barrie, F.R.; Buck, W.R.; Demoulin, V.; Greuter, W.; Hawksworth, D.L.; Herendeen, P.S.; Knapp, S.; Marhold, K.; Prado, J.; Prud'homme Van Reine, W.F.; Smith, G.F.; Wiersema, J.H.; Turland, N.J.) (2012). International Code of Nomenclature for algae, fungi, and plants (Melbourne Code) adopted by the Eighteenth International Botanical Congress Melbourne, Australia, July 2011. Regnum Vegetabile 154. A.R.G. Gantner Verlag KG. ISBN 978-3-87429-425-6.
- "'Columbia' and 'Liberty' Planetree" (PDF). U.S. National Arboretum. 1999. Retrieved 19 March 2017.
- Gledhill, David (2008). The Names of Plants. Cambridge: Cambridge University Press. p. 23. ISBN 9780521685535.
- Warschefsky, E.; Penmetsa, R. V.; Cook, D. R.; von Wettberg, E. J. B. (8 October 2014). "Back to the wilds: Tapping evolutionary adaptations for resilient crops through systematic hybridization with crop wild relatives". American Journal of Botany. 101 (10): 1791–1800. doi:10.3732/ajb.1400116. PMID 25326621.
- Stace, C. A. (1987). "Triticale: A Case of Nomenclatural Mistreatment". Taxon. 36 (2): 445–452. doi:10.2307/1221447. JSTOR 1221447.
- Darrow, G.M. (1955). "Blackberry—raspberry hybrids". Journal of Heredity. 46 (2): 67–71. doi:10.1093/oxfordjournals.jhered.a106521.
- Carrington, Sean; Fraser, HenryC (2003). "Grapefruit". A~Z of Barbados Heritage. Macmillan Caribbean. pp. 90–91. ISBN 978-0-333-92068-8.
- "Mint Genomics Resource: Species". Lange Laboratory, Washington State University. Archived from the original on 21 March 2017. Retrieved 20 March 2017.
- Hull, R. (2009). "A Short Guide to the London Plane" (PDF). Retrieved 2 February 2016.
- Venables, B. (4 March 2015). "The Secret History of the London Plane Tree". Londonist. Archived from the original on 2 February 2016. Retrieved 2 February 2016.
- "Legacy Bulbs Six". Pacific Bulb Society. Retrieved 20 March 2017.
- "Lilium Hybrids". Pacific Bulb Society. Retrieved 22 March 2015.
- "University of Colorado Principles of Genetics (MCDB 2150) Lecture 33: Chromosomal changes: Monosomy, Trisomy, Polyploidy, Structural Changes". University of Colorado. 21 November 2000. Archived from the original on 14 October 2012.
- Burr, Benjamin; Burr, Frances (2 October 2000). "How do seedless fruits arise and how are they propagated?". Scientific American.
- Vernot, B.; Akey, J. M. (2014). "Resurrecting Surviving Neandertal Lineages from Modern Human Genomes". Science. 343 (6174): 1017–1021. Bibcode:2014Sci...343.1017V. doi:10.1126/science.1245938. PMID 24476670.
- Green, R.E.; Krause, J.; Briggs, A.W.; Maricic, T.; Stenzel, U.; Kircher, M.; et al. (2010). "A Draft Sequence of the Neandertal Genome". Science. 328 (5979): 710–722. Bibcode:2010Sci...328..710G. doi:10.1126/science.1188021. PMC 5100745. PMID 20448178.
- Huerta-Sánchez, Emilia; Jin, Xin; Asan; Bianba, Zhuoma; Peter, Benjamin M.; Vinckenbosch, Nicolas; Liang, Yu; Yi, Xin; He, Mingze; Somel, Mehmet; Ni, Peixiang; Wang, Bo; Ou, Xiaohua; Huasang; Luosang, Jiangbai; Cuo, Zha XiPing; Li, Kui; Gao, Guoyi; Yin, Ye; Wang, Wei; Zhang, Xiuqing; Xu, Xun; Yang, Huanming; Li, Yingrui; Wang, Jian; Wang, Jun; Nielsen, Rasmus (2014). "Altitude adaptation in Tibetans caused by introgression of Denisovan-like DNA". Nature. 512 (7513): 194–197. Bibcode:2014Natur.512..194H. doi:10.1038/nature13408. PMC 4134395. PMID 25043035.
- Duarte, Cidalia; et al. (22 June 1999). "The early Upper Paleolithic human skeleton from the Abrigo do Lagar Velho (Portugal) and modern human emergence in Iberia". PNAS. 96 (13): 7604–7609. Bibcode:1999PNAS...96.7604D. doi:10.1073/pnas.96.13.7604. PMC 22133. PMID 10377462.
- Bower, Bruce (5 October 2016). "Animal hybrids may hold clues to Neandertal-human interbreeding". Sciencenews.
- Fu, Qiaomei; et al. (13 August 2015). "An early modern human from Romania with a recent Neanderthal ancestor". Nature. 524 (7564): 216–219. Bibcode:2015Natur.524..216F. doi:10.1038/nature14558. PMC 4537386. PMID 26098372.
- Wang, C.C.; Farina, S.E.; Li, H. (2013) [Online 2012]. "Neanderthal DNA and modern human origins". Quaternary International. 295: 126–129. Bibcode:2013QuInt.295..126W. doi:10.1016/j.quaint.2012.02.027.
- Condeme, Silvana; et al. (27 March 2013). "Possible Interbreeding in Late Italian Neanderthals? New Data from the Mezzena Jaw (Monti Lessini, Verona, Italy)". PLOS ONE. 9 (1): e59781. Bibcode:2013PLoSO...859781C. doi:10.1371/journal.pone.0059781. PMC 3609795. PMID 23544098.
- "Minotauros". Theoi. Retrieved 20 March 2017.
- "Bestiary". Theoi. Retrieved 20 March 2017.
- Kugel, James L. (2009). Traditions of the Bible: A Guide to the Bible As It Was at the Start of the Common Era. Harvard University Press. p. 198. ISBN 9780674039766.
- Kugel, James L. (1997). The Bible as it was. Harvard University Press. p. 110. ISBN 9780674069411.
- Boyd, Gregory A. God at War: The Bible & Spiritual Conflict. IVP Academic. p. 177.
- James L. Resseguie, "A Glossary of New Testament Narrative Criticism with Illustrations," in Religions, 10 (3: 217), 5-6.
- Craig Koester, Revelation and the End of All Things, 2nd ed. (Grand Rapids: Eerdmans, 2018), 102.
External links
![]() |
Wikimedia Commons has media related to Hybrids. |
- Artificial Hybridisation – Artificial Hybridisation in orchids
- Domestic Fowl Hybrids
- Hybridisation in animals Evolution Revolution: Two Species Become One, Study Says (nationalgeographic.com)
- Scientists Create Butterfly Hybrid – Creation of new species through hybridization was thought to be common only in plants, and rare in animals.
- What is a human admixed embryo?