Mosasaurus
Mosasaurus (/ˌmoʊzəˈsɔːrəs/; "lizard of the Meuse River") is the type genus of the mosasaurs, an extinct group of aquatic squamate reptiles. The genus lived from about 82 to 66 million years ago during the Campanian and Maastrichtian stages of the Late Cretaceous. The earliest fossils known to science were found as skulls in a chalk quarry near the Dutch city of Maastricht in the late 1700s, which were initially thought to have been the bones of crocodiles or whales. One particular skull discovered at around 1780, and which was seized during the French Revolutionary Wars for its scientific value and transported to Paris, was famously nicknamed the "great animal of Maastricht". In 1808, naturalist Georges Cuvier concluded that it belonged to a giant marine lizard with similarities to monitor lizards but otherwise unlike any animal known today. This concept was revolutionary at the time and helped support the then-developing ideas of extinction. However, Cuvier did not designate a scientific name for the new animal; this task was completed by William Daniel Conybeare in 1822 when he named it Mosasaurus in reference to its origin in fossil deposits near the Meuse River. The relationships between Mosasaurus and modern reptiles are controversial and scientists continue to debate whether its closest living relatives are monitor lizards or snakes.
Mosasaurus | |
---|---|
![]() | |
Reconstructed skeleton of M. hoffmannii at the Maastricht Natural History Museum | |
Scientific classification ![]() | |
Kingdom: | Animalia |
Phylum: | Chordata |
Class: | Reptilia |
Order: | Squamata |
Superfamily: | †Mosasauroidea |
Family: | †Mosasauridae |
Tribe: | †Mosasaurini |
Genus: | †Mosasaurus Conybeare, 1822 |
Type species | |
†Mosasaurus hoffmannii Mantell, 1829 | |
Species | |
Species pending reassessment
| |
Synonyms | |
List of synonyms
|
Traditional interpretations have estimated the maximum length of Mosasaurus to be up to 17.6 meters (58 ft), making it one of the largest mosasaur genera. Its skull, which was either broad or slender depending on the species, was equipped with robust jaws capable of swinging back and forth and strong muscles capable of powerful bites using dozens of large teeth designed for cutting prey. Its tail was long and ended in a paddle-like fluke that bent downwards. Mosasaurus was a predator that had excellent vision to compensate for its poor sense of smell, and a high metabolic rate that suggests it was warm-blooded, an adaptation found in other mosasaurs but no other squamates. The classification of Mosasaurus was historically problematic due to an unclear diagnosis of the type specimen. As a result, over fifty different species have been attributed to the genus in the past. A rediagnosis of the type specimen in 2017 helped resolve the taxonomy issue and confirmed at least five species to be within the genus and another five species still nominally classified within Mosasaurus are planned to be reassessed in a future study. Each species was variable with unique anatomical features differentiating them from the robustly-built M. hoffmannii to the slender and serpentine M. lemonnieri.
Fossil evidence suggests that Mosasaurus inhabited much of the Atlantic Ocean and the seaways adjacent to it. Continents that have recovered Mosasaurus fossils include North America, South America, Europe, Africa, Western Asia, and Antarctica. This distribution encompassed a wide range of oceanic climates including tropical, subtropical, temperate, and subpolar climates. Mosasaurus was a common large predator in these oceans and a dominant genus positioned at the top of the food chain. Scientists believe that its diet would have included virtually anything; it likely preyed on bony fish, sharks, cephalopods, birds, and other marine reptiles including sea turtles and other mosasaurs. It likely preferred to hunt in open water near the surface. From an ecological standpoint, Mosasaurus probably had a profound impact in the structuring of marine ecosystems; its arrival in some locations such as the Western Interior Seaway in North America has been marked with a complete turnover of faunal assemblages and diversity. Mosasaurus faced competition with other large predatory mosasaurs such as Prognathodon and Tylosaurus—which are known to feed on similar prey—though they were able to coexist in the same ecosystems through niche partitioning. There were conflicts among them, as an attack on Mosasaurus by Tylosaurus has been documented. Several discovered fossils illustrated deliberate attacks on Mosasaurus individuals by another of the same species. Infighting likely took place in the form of snout grappling, similarly seen in modern crocodiles today.
Research history
First discoveries
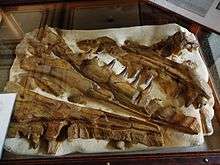
The first remains of Mosasaurus known to science are fragments of a skull discovered in 1764 at a subterranean chalk quarry under Mount Saint Peter, a hill near Maastricht, the Netherlands. It was collected by Lieutenant Jean Baptiste Drouin in 1766 and was procured in 1784 by museum director Martinus van Marum for the Teylers Museum at Haarlem. In 1790, van Marum published a description of the fossil, considering it to be a species of "big breathing fish" (in other words, a whale) under the classification Pisces cetacei.[12] This skull is still in the museum's collections and is cataloged as TM 7424.[13]
Around 1780,[lower-alpha 1] a second more complete skull was discovered at the same quarry. A retired Dutch army physician named Johann Leonard Hoffmann took a keen interest in this specimen, who corresponded with the famous biologist Petrus Camper regarding its identification. Hoffmann, who had previously collected various mosasaur bones in 1770, presumed that the animal was a crocodile.[14] Camper disagreed, and in 1786 he concluded that the remains were of an "unknown species of toothed whale"; he published his studies of the fossil that year in the Philosophical Transactions of the Royal Society of London,[16] which was considered to be the most prestigious scientific journal in the world at the time. As a result, the second skull gained international fame.[17] During this time, the fossil was under the possession of canon Theodorus Joannes Godding, who owned the portion of the land it was discovered in. Godding was struck by its beauty and took every measure to conserve it, eventually displaying it inside a grotto behind his house.[14]
Maastricht, an important Austrian fortress city at the time, was captured during the French Revolutionary Wars by the armies of General Jean-Baptiste Kléber in November 1794. Four days after the conquest, the fossil was looted from Godding's possession by French soldiers due to its international scientific value[17] under the orders of Kléber,[18] carried out by political commissar Augustin-Lucie de Frécine. According to an account by Godding's niece and heiress Rosa, Frécine first pretended to be interested in studying the famous remains and corresponded with Godding via letter to arrange a visit to his cottage to personally examine it. Frécine never visited, and instead sent six armed soldiers to forcefully confiscate the fossil under the pretext that he was ill and wanted to study it at his home.[14][17] Four days after the seizure, the National Convention decreed that the specimen was to be transported to the National Museum of Natural History, France. By the time it arrived at the museum, various parts of the skull were lost. In an 1816 reclamation request, Rosa claimed that she still possessed two of the missing lateral parts of the skull that were not taken by Frécine. However, the fate of these bones is unknown, and some historians believe that Rosa mentioned them in hopes of negotiating indemnity. The French government refused to return the fossil but recompensed Godding in 1827 by exempting him from war taxes.[17]
There is a popular legend regarding Godding's ownership of the specimen and its subsequent acquisition by the French, which is based on the account of geologist Barthélemy Faujas de Saint-Fond (one of the four men that arrived in Maastricht in January 1795 to confiscate any public objects of scientific value for France) in his 1798 publication Histoire naturelle de la montagne de Saint-Pierre de Maestricht (Natural history of the mountain of Saint-Pierre of Maastricht). According to Faujas, Hoffmann was the original owner of the specimen, which he purchased from the quarrymen and helped excavate. When the news of this discovery reached Godding, whom Faujas painted as a malevolent figure, he sought to take possession of the greatly valuable specimen for himself and filed a lawsuit against Hoffmann, claiming his rights as landowner. Due to Godding's position as a canon, he influenced the courts and was able to force Hoffmann to relinquish the fossil and pay for the costs of the lawsuit. When Maastricht was attacked by the French, the artillerymen were aware that the famous fossil was stored at Godding's house. Godding did not know his house was spared and he hid the specimen in a secret location in town. After the city's capture, Faujas personally helped secure the fossil while de Frécine offered a reward of 600 bottles of good wine to anyone who would locate and bring to him the skull undamaged. The next day, twelve grenadiers brought the fossil safely to de Frécine after assuring full compensation to Godding and collected their promised reward. Historians have found little evidence to back up Faujas' account. For example, there is no evidence that Hoffmann ever possessed the fossil, that a lawsuit involved him and Godding, or that Faujas was directly involved in acquiring the fossil. More reliable but contradictory accounts suggests that his narrative was mostly made up: Faujas was known to be a notorious liar who commonly embellished his stories, and it is likely that he falsified the story to disguise evidence of looting from a private owner (which was a war crime), to make French propaganda, or to simply impress others. Nevertheless, the legend created by Faujas' embellishment has helped elevate the second skull into one of cultural fame.[14][17]
Unlike its renowned contemporary, the first skull TM 7424 was not seized by the French after the capture of Maastricht. During Faujas and his three colleagues' mission in 1795, the collections of Teylers Museum, despite being famous, were protected from confiscation. It is possible that the four men were instructed to protect all private collections as "inviolable" unless its owner was declared a rebel, and were prohibited from seizing the Teylers Museum's collections. However, this protection may have also been due to van Marum's acquaintance with Faujas and André Thouin (another of the four men) since their first meeting in Paris in July 1795.[14]
Identification as an extinct marine lizard
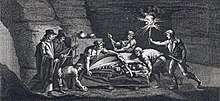
Before the second skull was seized by the French in late 1794, the two most popular hypotheses regarding its identification were that it represented the remains of either a crocodile or whale, as first argued by Hoffmann and Camper respectively. Hoffmann's identification as a crocodile was viewed by many at the time to be the most obvious answer; there were no widespread ideas of evolution and extinction at the time, and the skull superficially resembled a crocodile.[19] Moreover, among the various mosasaur bones Hoffmann collected in 1770 were phalanx bones which he assembled and placed onto a gypsum matrix; historians have noted that Hoffmann placed the reconstruction into the matrix in a way that distorted the view of some of the phalanges, creating an illusion that claws are present, which Hoffmann likely took as further evidence of a crocodile.[20] Camper's argument for a whale was based on the second skull's locality, features of the skull itself and other mosasaur bones he obtained, and its anatomical similarities with living animals. First, Camper noted that the skull's jawbones had a smooth texture and its teeth were solid at the root, similar to those in sperm whales and dissimilar to the crocodile's porous jawbones and hollow teeth. Second, Camper obtained mosasaur phalanges which he noted to be significantly different from those of crocodiles and instead suggested paddle-shaped limbs, which were another cetacean feature. Third, Camper noted the presence of teeth in the pterygoid bone of the skull, which he observed are not present in crocodiles but are present in many species of fish (Camper also thought that the rudimentary teeth of the sperm whale, which he erroneously believed was a species of fish, corresponded to pterygoid teeth). Lastly, Camper pointed out that all fossils from Maastricht are marine fossils, which indicates that the animal represented by the skull must have been a marine animal. Because he erroneously believed that crocodiles are entirely freshwater animals, Camper concluded by process of elimination that the animal could only be a whale.[19]
The second skull arrived at the National Museum of Natural History, France in 1795, where it is now cataloged as MNHN AC 9648. It attracted the attention of more scientists and was referred to as le grand animal fossile des carrières de Maestricht,[14] or the "great animal of Maastricht".[lower-alpha 2][13] One of the scientists was Camper's son Adriaan Gilles Camper. Originally intending to defend his father's arguments, Camper Jr. instead became the first to understand that the crocodile and cetacean hypotheses were both erroneous; based on his own examinations of MNHN AC 9648 and his father's fossils, he found that their anatomical features were more similar to squamates and varanoids. He concluded that the animal must have been a large marine lizard with varanoid affinities. In 1799, Camper Jr. discussed his conclusions with the French naturalist Georges Cuvier. Cuvier studied MNHN AC 9648, and in 1808 he confirmed Camper Jr.'s identification of a large marine lizard, but as an extinct form unlike any today.[19] The fossil had already become part of Cuvier's first speculations on the possibility of species going extinct, which paved the way for his theory of catastrophism or "consecutive creations", one of the predecessors of the theory of evolution. Prior to this, almost all fossils, when recognized as having come from once-living life forms, were interpreted as forms similar to those of the modern day. Cuvier's idea of the Maastricht specimen being a gigantic version of a modern animal unlike any species alive today seemed strange, even to him.[21] The idea was so important to Cuvier that in 1812 he proclaimed, "above all, the precise determination of the famous animal from Maastricht seems to us as important for the theory of zoological laws, as for the history of the globe."[14] Cuvier justified his concepts by trusting his techniques in the then-developing field of comparative anatomy, which he had already used to identify giant extinct members of other modern groups.[21]
Even though the binomial naming system was well established at the time, Cuvier never designated a scientific name to the new species and for a while, it remained being referred to as the "great animal of Maastricht". In 1822, English doctor James Parkinson published a conversation that included a suggestion made by Llandaff dean William Daniel Conybeare to refer to the species as the Mosasaurus, a portmanteau derived from the words Mosa (the Latin translation for the Meuse River that passed along Mount Saint Peter) and saurus (the romanization of the Ancient Greek σαῦρος, meaning "lizard"), as a temporary name until Cuvier decided on a permanent scientific name. Cuvier never made one; instead, he himself adopted Mosasaurus as the species' genus and designated MNHN AC 9648 as its holotype. In 1829, English paleontologist Gideon Mantell added the specific epithet hoffmannii in 1829 in honor of Hoffmann.[lower-alpha 3][13][7]
Later discoveries
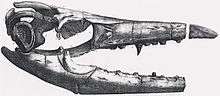
The first possible recorded discovery of a mosasaur in North America was of a partial skeleton described as "a fish" in 1804 by Meriwether Lewis and William Clark's Corps of Discovery during their 1804–1806 expedition across the western United States. It was found by Sergeant Patrick Gass on black sulfur bluffs near the Cedar Island alongside the Missouri River[22][23] and consisted of some teeth and a disarticulated vertebral column measuring 45 feet (14 m) in length. Four members of the expedition recorded the discovery in their journals including Clark and Gass.[23] Some parts of the fossil were collected and sent back to Washington, D.C., where it was lost before any proper documentation could be made. Paleontologist Mike Everhart of the Sternberg Museum speculated that the fossil may have been that of a mosasaur, possibly Mosasaurus.[22][24]
The earliest description of North American fossils firmly attributed to the genus Mosasaurus was made in 1818 by naturalist Samuel L. Mitchill of the New York Academy of Sciences (then called the Lyceum of Natural History). The described fossils were of a tooth and jaw fragment recovered from a marl pit from Monmouth County, New Jersey, which Mitchell described as "a lizard monster or saurian animal resembling the famous fossil reptile of Maestricht", implying that the fossils had affinities with the then-unnamed M. hoffmannii holotype from Maastricht. Cuvier was aware of this discovery but doubted whether it belonged to the genus Mosasaurus. Additionally, another foreign naturalist "unreservedly" declared that the fossils instead belonged to a species of Ichthyosaurus.[lower-alpha 4] In 1830, zoologist James Ellsworth De Kay, another member of the Lyceum, reexamined the specimen; he concluded that it was indeed a species of Mosasaurus and was considerably larger than the M. hoffmannii holotype, making it the largest fossil reptile ever discovered in the continent at the time.[25] Whether the two belonged to the same species or not remained unknown until 1838 when German paleontologist Heinrich Georg Bronn designated the New Jersey specimen as a new species and named it Mosasaurus dekayi in honor of De Kay's efforts.[26] However, the specimen was lost and the taxon was declared a nomen dubium in 2005.[7][2] There are some additional fossils from New Jersey that have been historically referred to as M. dekayi, but paleontologists have reidentified them as fossils of M. hoffmannii.[7][27]
The type specimen of the second species M. missouriensis was first discovered in the early 1830s, recovered by a fur trapper near the Big Bend of the Missouri River. This specimen, which consisted of some vertebrae and a partially complete articulated skull notably missing the end of its snout, was brought back to St. Louis, where it was purchased by an Indian agent as home decoration. This fossil caught the attention of German prince Maximilian of Weid-Neuwied during his 1832–1834 travels in the American West. He purchased the fossil and subsequently sent it to naturalist Georg August Goldfuss of the University of Bonn for research. It was kept in the university's collections under the specimen catalog number RFWUIP 1327. Goldfuss carefully prepared and described the specimen, which he concluded was of a new species of Mosasaurus and in 1845 named it M. maximiliani in honor of Maximilian.[22] However, earlier in 1834, American naturalist Richard Harlan published a description of a partial fossil snout he obtained from a trader from the Rocky Mountains who found it in the same locality as the Goldfuss specimen. Harlan thought it belonged to a species of Ichthyosaurus based on perceived similarities with the skeletons from England in features of the teeth and positioning of the nostrils and named it Ichthyosaurus missouriensis.[28] In 1839, he revised this identification after noticing differences in the premaxillary bone and pores between the snouts of the fossil and those of Ichthyosaurus and instead thought that the fossil actually pertained to a new genus of a frog or salamander-like amphibian, reassigning it to the genus Bactrachiosaurus.[29] For unknown reasons, a publication in the same year from the Société géologique de France documented Harlan alternatively reporting the new genus as Bactrachotherium.[30] Later authors argued that the snout belonged to neither an ichthyosaur nor an amphibian but to a mosasaur, and suspected that it may have been the snout that was missing in the Goldfuss skull. This could not be confirmed at the time because the fossil snout was lost. In 2004, it was rediscovered inside the collections of the National Museum of Natural History, France under the catalog number MNHN 958. The snout matched perfectly into the Goldfuss skull, confirming that it was the specimen's missing snout. Because of its earlier description, Harlan's taxon took priority, making the final scientific name M. missouriensis.[22]
Scientists have initially imagined that Mosasaurus had webbed feet and terrestrial limbs and thus was an amphibious marine reptile capable of both terrestrial and aquatic locomotion. At the time, scientists like Goldfuss argued that known skeletal features of Mosasaurus at the time such as an elastic vertebral column indicated a walking ability; if Mosasaurus was entirely aquatic, it would have been better supported by a stiff backbone. But in 1854, German zoologist Hermann Schlegel became the first to prove through anatomical evidence that Mosasaurus had flippers instead of feet. Using fossils of Mosasaurus phalanges including the gypsum-encased specimens collected by Hoffmann (which Schlegel extracted from the gypsum, noting that it may have misled previous scientists), he observed that they were broad and flat and showed no indication of muscle or tendon attachment, indicating that Mosasaurus was incapable of walking and instead had flipper-like limbs for a fully aquatic lifestyle. Schlegel's hypothesis was largely ignored by his contemporaries, but was more widely accepted in the 1870s when more complete mosasaur fossils in North America were discovered by American paleontologists Othniel Charles Marsh and Edward Drinker Cope.[19]

One of the earliest paleoart depictions of Mosasaurus is a life-size concrete sculpture constructed by natural history sculptor Benjamin Waterhouse Hawkins between 1852 and 1854 as part of the collection of sculptures of prehistoric animals on display at the Crystal Palace in London, the former house of the Great Exhibition. Hawkins sculpted the model under the direction of the English paleontologist Sir Richard Owen, who was informed on the possible appearance of Mosasaurus primarily based on the holotype skull. Given the knowledge of the possible relationships between Mosasaurus and monitor lizards, Hawkins depicted the prehistoric animal as essentially a water-going monitor lizard. The head was large and boxy, which was informed by Owen's estimations of the holotype skull's dimensions being 2.5 feet (0.76 m) × 5 feet (1.5 m), with dorsal posteriorly-placed nostrils, large volumes of soft tissue around the eyes, and lips reminiscent of monitor lizards. The skin was given a robust scaley texture similar to those found in larger monitor lizards such as the komodo dragon. Depicted limbs include a right single flipper, which reflected on the aquatic nature of Mosasaurus. However, the model was uniquely sculpted deliberately incomplete, with only the head, back, and single flipper having been constructed. This has been commonly attributed to Owen's lack of clear knowledge regarding the postcranial anatomy of Mosasaurus, but paleontologist and paleoartist Mark P. Witton found this unlikely given that Owen was able to guide a full speculative reconstruction of a Dicynodon sculpture (which was also known solely from skulls at the time). Witton instead suggested that time and financial constraints may have influenced Hawkins to cut corners and sculpt the Mosasaurus model in a way that would be incomplete but visually acceptable.[31] To hide the missing anatomical parts, the sculpture was partially submerged in the lake and placed near the Pterodactylus models at the far side of the main island.[32] Although some elements of the Mosasaurus sculpture such as the teeth have been accurately depicted, many elements of the model can be considered inaccurate, even at the time. The depiction of Mosasaurus with a boxy head, laterally-positioned nose, and flippers contradicted the studies of Goldfuss (1845), whose examinations of the vertebrae and near-complete and undistorted skull of M. missouriensis instead called for a narrower skull, dorsally-positioned nostrils, and amphibious terrestrial limbs (the latter of which is incorrect in modern standards). The ignorance of these findings may have been due to a general ignorance of Goldfuss's studies by other contemporaneous scientists.[31]
Confirmed species other than M. hoffmannii and M. missouriensis (considered to be the most well-known and studied species of the Mosasaurus genus) have been described.[5] In 1881, Cope described the third Mosasaurus species from fossils including a partial lower jaw, some teeth and vertebrae, and limb bones sent to him from a colleague who discovered them in deposits around Freehold Township, New Jersey;[33] these fossils now reside in the American Museum of Natural History as AMNH 1380.[11] Cope declared that the fossils represented a new species of Clidastes based on their slender build and named it Clidastes conodon.[33] But in 1966, paleontologists Donald Baird and Gerard R. Case reexamined the holotype fossils and found that the species belonged under Mosasaurus instead and renamed it Mosasaurus conodon.[11] Cope did not provide an etymology for the specific epithet conodon,[33] but etymologist Ben Creisler suggested that it may be a portmanteau meaning "cone tooth", derived from the Ancient Greek κῶνος (kônos, meaning "cone") and ὀδών (odṓn, meaning "tooth"), likely in reference to the smooth-surfaced conical teeth characteristic of the species.[34]
_(20248316020).jpg)
The fourth species M. lemonnieri was described in 1889 by Belgian paleontologist Louis Dollo from a fairly-complete skull recovered from a phosphate quarry owned by the Solvay S.A. company in the Ciply Basin of Belgium. The skull was one of many fossils donated to the Royal Belgian Institute of Natural Sciences by Alfred Lemonnier, the director of the quarry; as such, Dollo named the species in his honor.[35] In subsequent years, further mining of the quarry yielded additional well-preserved fossils of the species, some of which were described by Dollo in later papers. These fossils include multiple partial skeletons, which were enough to almost represent the entire skeleton of M. lemonnieri. All known fossils of the species reside in the collections of the same museum; the holotype skull is cataloged as IRSNB R28.[7][36] Despite being the most anatomically well-represented among the genus, the species was largely ignored by scientific literature. Paleontologist Theagarten Lingham-Soliar of Nelson Mandela University suggested two reasons for such neglect: the first reason was that M. lemonnieri fossils were endemic to Belgium and the Netherlands, areas that have generally not attracted the attention of mosasaur paleontologists. The second reason was that M. lemonnieri was overshadowed by its more famous and history-rich congeneric M. hoffmannii.[36] The species has historically been a controversial taxon, and some of the literature that gives attention to M. lemonnieri argue that it is synonymous with other species.[37] In 1967, research professor Dale Russell of North Carolina State University argued that differences between the fossils of M. lemonnieri and M. conodon were too minor to support species-level separation; per the principle of priority, Russell designated M. lemonnieri as a junior synonym of M. conodon.[38] In a study published in 2000, Lingham-Soliar refuted Russell's classification through a comprehensive examination of the Royal Belgian Institute's specimens, identifying significant differences in skull morphology. However, he declared that better studies of M. conodon would be needed to settle the issue of synonymy.[36] Such a study was done in a 2014 paper by Takehito Ikejiri of the University of Alabama and Spencer G. Lucas of the New Mexico Museum of Natural History and Science, who both examined the skull of M. conodon in detail and also argued that M. conodon and M. lemonnieri are distinct species.[11] Alternatively, paleontologists Eric Mulder, Dirk Cornelissen, and Louis Verding suggested in a 2004 discussion that M. lemonnieri could actually be juvenile representatives of M. hoffmannii; it was justified by the argument that differences between the two species can only be observable in "ideal cases", and that these differences could be explained by age-based variation. However, there are still some differences such as the exclusive presence of fluting in M. lemonnieri teeth that might indicate the two species being distinct. It has been expressed that better studies are still needed for more conclusive evidence of synonymy.[39]
The fifth species, M. beaugei, was described in 1952 by French paleontologist Camille Arambourg in part of a large-scale project since 1934 to study and provide paleontological and stratigraphic data of Morocco to phosphate miners such as the OCP Group.[40] The species was described from nine isolated teeth originating from phosphate deposits in the Oulad Abdoun Basin and the Ganntour Basin in Morocco[41] and was named in honor of OCP General Director Alfred Beaugé, who invited Arambourg to partake in the research project and helped provide local fossils.[40] The teeth are currently in the National Museum of Natural History, France. One of the teeth, which is cataloged as MNHN PMC 7, was designated as the holotype. In a 2004 study, paleontologists led by Nathalie Bardet of the same museum reexamined Arambourg's teeth and found that only three can be firmly attributed to M. beaugei. Two of the other teeth were described as having variations that may possibly be within the species but were ultimately not referred to M. beaugei, while the remaining four teeth were found to be unrelated to it and of uncertain identity. The study also described more complete M. beaugei fossils in the form of two well-preserved skulls recovered from the Oulad Abdoun Basin.[41]
Description
Size
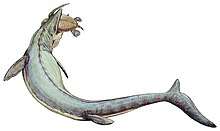
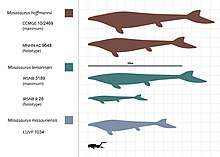
A common method that is used to determine the total length of mosasaur individuals is by measuring the length of the skull or lower jaw, which could then be used to yield the total length using a skull or lower jaw to body proportion. The most widely used proportion for M. hoffmannii is one recorded by Russell (1967), which equates the ratio between the lower jaw and the body being 1:10, although no rationale is given for this. When applying the ratio in the same study, Russell yielded a total length of 12.4 meters (41 ft) using the largest reliable skull he could access.[38] However, larger fossils are known, most notably a large M. hoffmannii lower jaw found in the Russian city of Penza in 1927. When it was re-examined in a 2014 study, it was found to measure 1,700 millimetres (67 in) in length and thus yielded a total length of approximately 17 meters (56 ft) using Russell's proportions.[42] In 1995, Lingham-Soliar applied Russell's proportions on a partial lower jaw measuring 900 millimeters (35 in) that reportedly "is reliably estimated at 1600 mm" and yielded a total length of 17.6 meters (58 ft).[43]
An alternative ratio used to determine the total length of Mosasaurus was proposed in 2014 by a study led by paleontologist Federico Fanti of the University of Bologna, which aimed to be more consistent with the proportions of related genera. Based on a near-complete skeleton of the mosasaurine and Mosasaurus relative Prognathodon overtoni, this proportion is of a 1:7 skull to body ratio. When applied to a Mosasaurus snout of comparable size to that from a skull measuring 145 centimeters (57 in) in length, the study yielded a total length of at least 11 meters (36 ft).[44] When applied to the Penza specimen, it yielded a total length of approximately 12 meters (39 ft).[42][44]
A body proportion ratio has also been recorded for M. lemonnieri. This ratio was recorded by Dollo in 1892 based on measurements of a partial skeleton of M. lemonnieri that consists of, but not limited to, a full-length skull and a nearly-complete partially articulated vertebral column; this skeleton is currently cataloged as IRSNB 3119. It equates the ratio between the skull and body being roughly 1:11.[45] When applying the ratio on various M. lemonnieri fossils in his access, Dollo recorded lengths ranging between 7–10 metres (23–33 ft) in total length.[38][45] The largest known skull of M. lemonnieri is currently IRSNB 3189, which measures approximately 114 centimetres (45 in) in length. When applying Dollo's ratio, it yields a total body length of around 12.5 metres (41 ft).[45][36]
The skulls of M. missouriensis, M. conodon, and M. beaugei are smaller in length than that of M. hoffmannii and large M. lemonnieri. Large individuals of M. missouriensis typically have skull lengths around or exceeding 1 meter (3.3 ft),[7] with one skull from the collections of the University of Kansas cataloged as KUVP 1034 being measured at 111 centimeters (44 in) in length. M. conodon is smaller, with its largest known skull measuring around 97.7 centimeters (38.5 in) in length.[11] The largest skull of M. beaugei described in scientific literature is incomplete but is estimated to have measured 110 centimeters (43 in) in length.[41]
Dentition
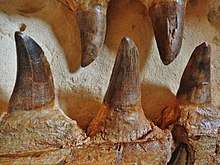
The features of teeth in Mosasaurus vary across species, but characteristics that unify the genus include highly prismatic surfaces (prism-shaped enamel circumference), two opposite cutting edges, and a design specialized for cutting prey.[11][41][46][47] Mosasaurus teeth are considered to be large and robust with the exception of in M. conodon and M. lemonnieri, which instead have more slender teeth.[11][41] The cutting edges of Mosasaurus can be serrated depending on the species: the cutting edges in M. hoffmannii and M. missouriensis are finely serrated,[5][10] while M. conodon and M. lemonnieri lack serrations;[lower-alpha 5][39] the cutting edges in M. beaugei are neither serrated nor smooth, but instead possesses minute crenulations.[41] The number of prisms, or flat sides on a prismatic tooth's circumference, in Mosasaurus teeth can slightly vary between tooth types and general patterns differ between species—M. hoffmannii had two to three prisms on the labial side (the side facing outwards) and no prisms on the lingual side (the side facing the tongue), M. missouriensis had four to six labial prisms and eight lingual prisms, M. lemonnieri had eight to ten labial prisms, and M. beaugei had three to five labial prisms and eight to nine lingual prisms.[lower-alpha 6][41]
Like all mosasaurs, Mosasaurus had four types of teeth which were named after the jaw bones they were located on. On the upper jaw, there were three types and were the premaxillary teeth, maxillary teeth, and pterygoid teeth. On the lower jaw, only one type, the dentary teeth, were present. A unified dental formula representing the Mosasaurus genus is 2.12–16.8–1614–17: this means that in each jaw row, from front to back, Mosasaurus had: two premaxillary teeth, twelve to sixteen maxillary teeth, and eight to sixteen pterygoid teeth on the upper jaw and fourteen to seventeen dentary teeth on the lower jaw.[9][11][41][48] The number of teeth in the maxillary, pterygoid, and dentary positions vary between species and sometimes even individuals—M. hoffmannii had fourteen to sixteen maxillary teeth, fourteen to fifteen dentary teeth, and eight pterygoid teeth;[11][42][43] M. missouriensis had fourteen to fifteen maxillary teeth, fourteen to fifteen dentary teeth, and eight to nine pterygoid teeth;[9][41][49] M. conodon had fourteen to fifteen maxillary teeth, sixteen to seventeen dentary teeth, and eight pterygoid teeth;[11][41] M. lemonnieri had fifteen maxillary teeth and fourteen to seventeen dentary teeth;[11][41] and M. beaugei had twelve to thirteen maxillary teeth and fourteen to sixteen dentary teeth.[41] One indeterminate specimen of Mosasaurus similar to M. conodon from the Pembina Gorge State Recreation Area in North Dakota was found to have an unusual count of sixteen pterygoid teeth, which is a count far greater than those in known species.[48]
Mosasaurus possessed a thecodont dentition, meaning that the roots of its teeth were deeply cemented within the jaw bone. Mosasaurus did not utilize permanent teeth and constantly shed them. Replacement teeth develop within the roots of the original tooth inside a resorption pit through an eight-stage process that is autapomorphic, or distinctly unique, to mosasaurs. The first stage is characterized by the mineralization of a small tooth crown developed elsewhere that descends into the resorption pit by the second stage. In the third stage, the developing crown firmly cements itself within the resorption pit and grows in size; by the fourth stage, it is of the same size as the crown in the original tooth. Stages five and six are characterized by the development of the replacement tooth's root: in stage five the root develops vertically, and in stage six the root expands in all directions, to the point that the replacement tooth becomes exposed and actively pushes on the original tooth. In the seventh stage, the original tooth is shed and the now-independent replacement tooth begins to anchor itself into the vacancy. In the eighth and final stage, the replacement tooth has grown to firmly anchor itself.[50] Chemical studies conducted on a M. hoffmannii maxillary tooth approximated the duration of the deposition of odontoblasts, the cells responsible for the formation of dentin, to be 511 days and the full development of dentin to take 233 days.[lower-alpha 7][51]
Skull
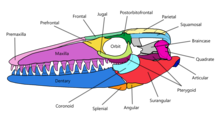
The skull of Mosasaurus is akin to a large cone that tapers off to a short and conical rostrum that extends a little beyond the premaxillary teeth. Above the gum line in both jaws, a single-file pattern of small pits known as foramina are lined parallel to the jawline; they are used to hold the terminal branches of jaw nerves. A number of foramina are also present along the rostrum in a pattern similar to that found in skulls of Clidastes.[43] The upper jaws in most species are robustly built, broad, and deep except in M. conodon, which are slender.[11] The premaxillary bar, the long portion of the premaxillary bone extending posteriorly to the premaxillary teeth, is robust and does not constrict near the middle like in typical mosasaur premaxillary bars.[10] The external nares are moderately sized and measure around 21–24% of the skull length in M. hoffmannii. They are positioned more posteriorly than any other mosasaur and begin above the fourth or fifth maxillary teeth; posterior positioning is only exceeded in Goronyosaurus.[43]
The palatal complex, which consists of the pterygoid bones, palatine bone, and nearby bones of other processes, was tightly packed to provide greater cranial stability. The neurocranium provided a brain that was narrow and relatively small compared to other mosasaurs. In contrast, the braincase of the mosasaur Plioplatecarpus marshi provided for a brain around twice the size of that in M. hoffmannii while being a species being only half the length of the latter. Spaces within the braincase providing for the occipital lobe and cerebral hemisphere are narrow and shallow, suggesting that such brain parts were relatively small. The parietal foramen in Mosasaurus, which is associated with the parietal eye, is the smallest in the Mosasauridae family.[43] The trachea likely stretched from the esophagus to below the back end of the mandible's coronoid process, where it split into smaller pairs of bronchi that extended parallel to each other.[9]
Postcranial skeleton

The most complete skeleton of Mosasaurus, whose species-level identification is debated[11][7] and is on display at the Museum of Geology at the South Dakota School of Mines and Technology under the catalog SDSM 452, has seven cervical vertebrae in the neck, thirty-eight dorsal vertebrae (which includes thoracic and lumbar vertebrae) in the back, and eight pygal vertebrae (front tail vertebrae lacking chevrons) followed by sixty-eight caudal vertebrae in the tail. All species of Mosasaurus have seven cervical vertebrae, but other vertebral counts vary among them. Various partial skeletons of M. conodon, M. hoffmannii (M. maximus), and M. missouriensis suggest that M. conodon likely had up to thirty-six dorsal vertebrae and nine pygal vertebrae; M. hoffmannii had likely up to thirty-two thoracic vertebrae and ten pygal vertebrae;[lower-alpha 8][11][36] and M. missouriensis around thirty-three dorsal vertebrae, eleven pygal vertebrae, and at least seventy-nine caudal vertebrae. M. lemmonieri had the most vertebrae in the genus, with up to around forty dorsal vertebrae, twenty-two pygal vertebrae, and ninety caudal vertebrae.[7][36] Compared to other mosasaurs, the rib cages of Mosasaurus are unusually deep and form an almost perfect semicircle, giving it a barrel-shaped chest. Extensive cartilage likely connected the ribs with the sternum, which would have facilitated breathing movements and compression when in deeper waters.[43] The texture of the bones is virtually identical with that in modern whales, which implies Mosasaurus enjoyed a high range of aquatic adaptability and neutral buoyancy seen in cetaceans.[52]
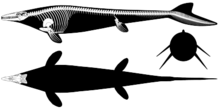
The tail is bilobed and hypocercal, which means that the tail vertebrae extends toward the lower lobe. The centra[lower-alpha 9] of the tail vertebrae gradually shorten around the center of the tail and lengthen behind the center, suggesting rigidness around the tail center and excellent flexibility posterior to it. Like most advanced mosasaurs, the tail bends slightly downwards as it approached the center, but this bend is at a small degree with little offset from the body. Mosasaurus also has large haemal arches in the caudal vertebrae that bend near the middle of the tail, which contrasts with the reduction of haemal arches in other marine reptiles such as ichthyosaurs. These and other features support a large and powerful paddle-like fluke in Mosasaurus.[52]
The forelimbs of Mosasaurus are wide and robust.[11][43] The scapula and humerus are fan-shaped and wider than tall. The radius and ulna are short, but the former is taller and larger than the latter.[11] The paddle is supported with five sets of finger-like metacarpals and phalanges with the fifth being shorter and offset from the rest. The overall structure of the paddle is compressed, similar to that in Plotosaurus, and is well-suited for utilization for faster swimming.[11][43] In the hindlimbs, the paddle is supported with four sets of digits. The ilium is rod-like and slender; in M. missouriensis, it is around 1.5 times longer than the femur. The femur itself is about twice as long than it is wide and ends in a pair of distinct articular facets that meet at an angle of approximately 120°.[9]
Classification
History of taxonomy
Because the rules of nomenclature were not well defined at the time, 19th century scientists did not give Mosasaurus a proper diagnosis during its first descriptions. This led to ambiguity regarding the definition of the genus, which led it to become a wastebasket taxon that contained as many as fifty different species. The taxonomic issue was so severe that there were cases of species that were found to be junior synonyms of species that were found to be junior synonyms themselves. This issue was recognized by many scientists at the time, but efforts to clean up the taxonomy of Mosasaurus were hindered due to a lack of a clear diagnosis.[5][7]
In 1967, Russell published Systematics and Morphology of American Mosasaurs, which contained one of the earliest proper diagnoses of Mosasaurus. Although his work is considered incomplete as he worked solely on North American representatives (and as a result did not examine European representatives such as M. hoffmannii), Russell was able to significantly revise the taxon and establish a more clear diagnosis of the genus. He identified eight species that he considered valid—M. hoffmannii, M. missouriensis, M. conodon, M. dekayi, M. maximus, M. gaudryi, M. lonzeensis, and M. ivoensis. Later scientists would revise this further: M. gaudryi was reclassified as a tylosaurine in 1990, M. maximus was synonymized with M. hoffmannii in 1999 (although some scientists maintain that it is a distinct species), the invalidated species M. lemonnieri was resurrected in 2000, M. ivoensis was moved to the genus Tylosaurus in 2002, and M. dekayi and M. lonzeensis became dubious. During the late 20th century, scientists described four additional species from fossils found in the Pacific—M. mokoroa, M. hobetsuensis, M. flemingi, and M. prismaticus.[5][7] In 1995, Lingham-Soliar published one of the earliest full diagnoses of M. hoffmannii, which provided detailed descriptions of the known anatomy of the type species based on a large variety of fossils from deposits around Maastricht.[43] However, it has garnered some criticism for its reliance on referred specimens rather than just the holotype, especially on IRSNB R12, a fossil skull from the Royal Belgian Institute that has been questionably attributed to the species.[5][7]
In 2016, the doctoral thesis of paleontologist Halle Street of the University of Alberta was published. This thesis, supervised by paleontologist Michael Caldwell, performed the first formal proper description and diagnosis of the Mosasaurus holotype since its identification over two hundred years prior.[7] The formal diagnosis of the holotype specimen allowed for a significant taxonomic revision of Mosasaurus. A phylogenetic study was performed in the thesis, which tested the relationships between M. hoffmannii and twelve candidate Mosasaurus species—M. missouriensis, M. dekayi, M. gracilis, M. maximus, M. conodon, M. lemonnieri, M. beaugei, M. ivoensis, M. mokoroa, M. hobetsuensis, M. flemingi, and M. prismaticus— with results representing a significant taxonomic revision of the Mosasaurus genus. Of the twelve candidate species, only M. missouriensis and M. lemonnieri were found to be distinct species within the genus. M. beaugei, M. dekayi, and M. maximus were recovered as junior synonyms of M. hoffmannii. The placement of M. gracilis and M. ivoensis outside of the Mosasaurinae subfamily was also reaffirmed. M. hobetsuensis and M. flemingi were recovered as representatives of Moanasaurus and were renamed accordingly. M. mokoroa and M. prismaticus were recovered as distinct genera, which were named Antipodinectes and Umikosaurus respectively. Representatives of M. conodon from the Midwestern United States were found to belong to M. missouriensis, while its East Coast representatives (including the holotype) were found to belong to a new genus that was subsequently named Aktisaurus. Lastly, the study found that the IRSNB R12 skull was a distinct species of Mosasaurus. It was named M. glycys, the specific epithet being a romanization of the Ancient Greek γλυκύς (ɡlykýs, meaning "sweet") in reference to the skull's residence in Belgium and the country's "reputation for chocolate production". Street stated in her thesis that its contents are intended to be published as scientific papers.[7]
The diagnosis of the Mosasaurus holotype was published in a 2017 paper co-authored with Caldwell.[5] The taxonomic revision of the genus has yet to be formally published but has been foreshadowed in Street and Caldwell (2017)[5] and by two abstracts presented during the 5th Triennial Mosasaur Meeting in 2016[53] and 5th Annual Meeting of the Canadian Society of Vertebrate Palaeontology in 2017.[54] Street and Caldwell (2017) also presented a brief preliminary taxonomic review of Mosasaurus that identified five likely valid species based on previous literature—[lower-alpha 10] M. hoffmannii, M. missouriensis, M. conodon, M. lemonnieri, and M. beaugei—and considered the four Pacific species to be possibly valid, pending formal reassessment in the future. M. dekayi was included in the list of possible valid taxa without its dubious status addressed, but was described as likely being a synonym of M. hoffmannii.[5]
Systematics and evolution
Mosasaurus is a member of the order Squamata (which comprises of lizards and snakes) and the type genus of the family Mosasauridae and subfamily Mosasaurinae. The genus also belongs to a tribe traditionally shared with the mosasaur genera Eremiasaurus, Plotosaurus,[55] and Moanasaurus,[lower-alpha 11][54] but the naming of this tribe is controversial. It was originally erected by Russell (1967) under the name Mosasaurini. Russell's description of the tribe was based on the belief that Plotosaurus was not closely related to Mosasaurus, which was classified into a separate tribe called the Plotosaurini. In a 1997 study, paleontologist Gorden Bell recovered Plotosaurus as a sister genus to Mosasaurus. This rendered the Mosasaurini tribe paraphyletic, which meant that it now contains a descendant lineage (Plotosaurini) that is not classified under it. Paraphylys are typically regarded as an unconformable way of classification and scientists often reclassify groups in order to eliminate such discrepancies if possible. In this case, Bell proposed that the Mosasaurini should be abandoned and that all members of the tribe should be incorporated into the Plotosaurini. While other scientists agree that a tribe containing Mosasaurus should be monophyletic, they argue that Mosasaurini should be the valid tribe. For example, in a 2012 study, Aaron LeBlanc of the University of Alberta, Caldwell, and Bardet argued that, while it is not necessarily invalid, abandoning Mosasaurini would not follow Linnean hierarchy principles and that the original diagnostics of the Plotosaurini is outdated.[55]
Relation with snakes or monitor lizards
The specific placement of mosasaurs within the Squamata, and thus the relationship of Mosasaurus with modern reptiles, has been controversial since its inception. Cuvier was the first scientist to deeply analyze the possible taxonomic placement of Mosasaurus. While his original 1808 hypothesis that the genus was a lizard with affinities to monitor lizards remained the most popular, Cuvier was uncertain, even at the time, about the accuracy of this placement. He simultaneously proposed a number of alternative hypotheses, with one such alternative suggesting that Mosasaurus instead had closer affinities with iguanas due to their shared presence of pterygoid teeth. With the absence of sufficient fossil evidence, researchers during the early and mid-19th century had little to work with. Instead, they primarily relied on stratigraphic associations and Cuvier's 1808 research on the holotype skull. Thus, in-depth research on the placement of Mosasaurus was not undertaken until the discovery of more complete mosasaur fossils during the late 19th century, which reignited research on the placement of mosasaurs as squamates.
In a span of about 30 to 40 years, paleontologists fiercely debated the issue, which created two major schools of thought: one that supported a monitor lizard relationship and one that supported a closer relationship with snakes.[57] The proposition of a snake relationship was spearheaded by Cope, who first published such a hypothesis in 1869 by proposing that mosasaurs, which he classified under a group called the Pythonomorpha, was the sister group of snakes. Some scientists went as far as to interpret mosasaurs as direct ancestors of snakes.[58] Opponents supporting a relationship with monitor lizards argued that mosasaurs should be placed within the infraorder Anguimorpha, with lower classifications varying from placing mosasaurs within Varanoidea or its sister taxa, or as true monitor lizards within Varanidae.[57] These debates spawned higher taxonomic groups that were erected in attempts to classify the placement of mosasaurs (although not all are compatible). One of these was the Mosasauria, a loosely-defined group erected by Marsh in 1880 but is still used by some researchers today that generally includes all descendants of the last common ancestor of Mosasaurus and some of its ancestral relatives, including the dolichosaurs Dolichosaurus and Coniasaurus and the marine squamate Adriosaurus.[59] In 1923, Charles Lewis Camp of the University of California, Berkeley, published Classification of the Lizards, in which he proposed through the review and rebuttal of previous arguments using his own anatomical observations that all taxa more closely related to Mosasaurus than Dolichosaurus should be classified into a superfamily called the Mosasauroidea, which would be a sister superfamily to the Varanoidea.[57][60] Camp's take on the subject virtually ended the debate for approximately 70 years, with nearly all subsequent studies supporting a relationship with monitor lizards. However, many studies continued to support placing mosasaurs within the Varanoidea.[57]
The debate was reignited with the publication of a 1997 cladistical study by paleontologist Michael S. Y. Lee of the University of Sydney, which recovered the Mosasauroidea as a sister taxon to the snake suborder Serpentes and resurrected the argument for a snake relationship.[57][61] Prior to Lee (1997), there had never been a modern phylogenetic analysis to specifically test the relationships between mosasaurs and snakes, nor any cladistical studies on squamates with both mosasaurs and snakes simultaneously included. Lee observed to be part of the reason for the trend of consistently classifying mosasaurs as varanoid lizards. Lee also resurrected the Pythonomorpha (which had long gone out of use) and redefined it to unify the Mosasauroidea and Serpentes under one clade.[61] This hypothesis was refined by multiple subsequent studies conducted by scientists such as Lee, Caldwell, and Alessandro Palci of the University of Modena and Reggio Emilia. In some of these studies, the Mosasauria clade was used to represent mosasaurs.[57][58] However, there still remained little consensus. For example, a large-scale 2008 phylogenetic study by paleontologist Jack Conrad of the American Museum of Natural History recovered the Mosasauria clade in a polytomy, or unresolved sister relationships, with monitor lizards and beaded lizards;[59] and a 2012 study led by Jacques Gauthier of Yale University recovered Mosasauria as a clade basal to both monitor lizards and snakes.[62]
During the 2010s, with the advent of the field of molecular genetics, some scientists argued that combining molecular data with morphological data can illustrate more accurate relationships between mosasaurs and living squamates.[63][64] An early study utilizing this technique was a 2009 study by Lee, which when using data extracted from nuclear and mitochondrial DNA in living squamates alongside morphological data recovered mosasaurs as a stem group to snakes,[65] which some later authors interpreted as placement of snakes within the Mosasauria clade itself.[63] However, a 2010 multi-author study led by John Weins of Stony Brook University attempted to replicate Lee (2009) using a larger dataset but instead yielded results that recovered the Mosasauria as a sister clade to the monitor lizards.[63] This, along with other studies concerning general squamate phylogeny, demonstrated many problems that molecular and morphological data presented. Convergent evolution is very common among unrelated squamates, which creates many rooms for data interpretation; many of these studies had results that often contradicted each other (i.e. completely different phylogenetic results by simply adding more datasets), which resulted in various conflicts creating even more uncertainty. As a result, some scientists chose to completely abandon the utilization of molecular data.[64][66] However, in recent years scientists have developed ways to resolve these conflicts. A prominent approach was utilized by a 2015 multi-author study led by Todd Reeder of San Diego State University: it closely integrated morphological, molecular, and paleontological data in a large dataset to overcome previous conflicts, which revealed less-obvious ("hidden") morphological support for molecular results including such that recovered Mosasauria as a sister clade to Serpentes.[64] Another approach was developed by biologist R. Alexander Pyron of The George Washington University in a 2016 study, which utilized a novel asymmetric approach of interpreting some problematic morphological datasets alongside molecular data, which ultimately also recovered Mosasauria as a sister clade to the Serpentes.[67]
Phylogeny and evolution of the genus
One of the earliest relevant attempts at an evolutionary study of Mosasaurus was done by Russell (1967),[68] which proposed that Mosasaurus evolved from a Clidastes-like mosasaur, and diverged into two lineages. Of these two hypothesized lineages, one gave rise to M. conodon and another led to a chronospecies sequence which contained in order of succession M. ivoensis, M. missouriensis, and M. maximus/hoffmanni.[38] However, Russell used a more primitive method of phylogenetics in his study as at the time the works giving rise to the modern field of cladistics had yet to be widely established.[68]
In 1997, Bell published the first cladistical study of North American mosasaurs. In it, he incorporated the species M. missouriensis, M. conodon, M. maximus, and an unnamed species identified as Mosasaurus sp.. Some findings of the study were in agreement with Russell (1967), such as Mosasaurus descending from an ancestral group which Clidastes is a member of and M. conodon being the most basal of the genus. Bell also found that Mosasaurus formed a sister relationship with another group that included Globidens and Prognathodon, and that M. maximus formed a sister relationship with Plotosaurus.[68] These findings are contrary to Russell (1967), which hypothesized that Prognathodon and Plotosaurus arose from different lineages.[38] The recovery of M. maximus as a sister species of Plotosaurus rendered Mosasaurus paraphyletic, but Bell (1997) nevertheless recognized Plotosaurus as a distinct genus.[68] Although some later studies have recovered the basal sister group of Mosasaurus to instead be Eremiasaurus or Plesiotylosaurus depending on the method of data interpretation used[55][69][56], with at least one study also recovering M. missouriensis to be the most basal species of the genus instead of M. conodon,[70] Bell's study served as a precedent for later studies that have mostly left the systematics of Mosasaurus unchanged.[9][7] However, a number of issues from this have been pointed out: first, the genus was severely underrepresented by incorporating only the three North American species M. hoffmannii/M. maximus, M. missouriensis, and M. conodon; by doing so, other important species such as M. lemonnieri, which is one of the most completely known in the genus, were neglected, which affected the results of the phylogenetic analyses.[7] Second, the studies relied on a still unclean and shaky taxonomy of the Mosasaurus genus due to the lack of a clear holotype diagnosis, which may be behind the paraphyletic status of Mosasaurus.[9][7] Third, there was still a lack of osteological comparative studies of large mosasaurines including Mosasaurus at the time, which also hampered accurate results.[9] These problems were addressed in the phylogenetic study in Street's doctoral thesis, which yielded different results.[7]
.jpg)

Conrad (2008) uniquely utilized only M. hoffmannii and M. lemonnieri in its phylogenetic analysis, which recovered M. hoffmannii as basal to a multitude of descending clades containing (in order of most to least basal) Globidens, M. lemonnieri, Goronyosaurus, and Plotosaurus. This result indicated that M. hoffmannii and M. lemonnieri are not related by genus and that M. lemonnieri did not belong in Mosasaurus.[59] However, the study utilized a method completely foreign to traditional phylogenetic studies on mosasaur species, namely the use of an artificial entity rather than a real taxon as the operational taxonomic unit outgroup because its focus was on higher rather than lower classification. As a result, it has been cautioned that lower-classification results such as the specific placement of Mosasaurus within the Mosasauria may contain technical problems that can affect its accuracy.[69]
The following cladogram on the left (Topology A) is modified from a maximum clade credibility tree inferred by a Bayesian analysis from the most recent major phylogenetic analysis of the Mosasaurinae subfamily by Madzia & Cau (2017), which has been self-described as a refinement of a larger phylogenetic study on mosasaurs by Simões et al., (2017).[56] The cladogram on the right (Topology B) is modified from Street's 2016 doctoral thesis that proposes a revision to the Mosasaurinae, with proposals for numerous new taxa and recombinations. Because Street (2016) is not a peer-reviewed publication,[7] it is not cited in Madzia & Cau (2017), but has been foreshadowed in the study.[56]
Topology A: Maximum clade credibility tree by Madzia & Cau (2017)[56]
|
Topology B: Proposed revision by Street (2016)[7]
|
Paleobiology
Head musculature and mechanics
Much of the knowledge on the musculature and mechanics of the Mosasaurus head are largely based on Lingham-Soliar's 1995 study on M. hoffmannii skulls. Because soft tissue like muscles do not easily fossilize, reconstruction of the head musculature is largely based on the properties of the skull, the nature of muscle scarring on the skull, and the musculature in extant monitor lizards.[43]
In modern lizards, the mechanical build of the skull is characterized by a four-pivot geometric structure in the cranium that allows flexible movement of the jaws, possibly to allow the animal to better position them when hunting and prevent deflection (the positioning of a prey in a way that increases its chance of escape from an attacking predator). However, the skull of M. hoffmannii is characterized by a rigid three-pivot geometric cranial structure, which indicates that its jaw mechanics were different than modern lizards; these cranial structures are united into strong interlocking sutures that can resist compression and shear forces caused by a downward thrust of the lower jaw muscles or an upward thrust of prey. This rigid but highly shock-absorbent structure of the cranium likely functioned to allow a powerful bite force during prey seizure.[43]
Like all mosasaurs, the lower jaws of Mosasaurus were capable of adduction, allowing it to swing back and forth. In many mosasaur genera such as Prognathodon and M. lemonnieri, this function mainly served to allow ratchet feeding, in which the pterygoid and jaws would "walk" captured prey into the mouth like a conveyor belt. However, especially compared to that in M. lemonnieri, the pterygoid teeth in M. hoffmannii are relatively small, which indicates ratchet feeding was rather insignificant when hunting and feeding.[43][36] Rather, M. hoffmannii likely swallowed its prey and used jaw adduction to assist in hard biting during prey seizure. The magnus adductor muscles, one of the muscles attaching the lower jaw to the cranium and has a major role in biting function, are massive, indicating that M. hoffmannii was capable of enormously powerful bite forces. The long, narrow, and heavy nature of the lower jaws and attachment of tendons at the coronoid process would have allowed quick opening and closing of the mouth with little energy input underwater, which may have contributed to the powerful bite force of M. hoffmannii and suggests that it would not have needed the strong magnus depressor muscles (jaw-opening muscles) seen in some plesiosaurs.[43]
Mobility and thermoregulation
Mosasaurus swam using its tail. The swimming style varied between species; M. hoffmannii utilized a sub-carangiform swimming style, which is best seen today in mackerels, while M. lemonnieri was more serpentine and likely moved in a more anguilliform eel-like style.[71] Its elongated paddle-like limbs functioned as a hydrofoil for maneuvering the animal. The paddle's steering function was enhanced by large muscle attachment from the outwards-facing side of the humerus to the radius and ulna and an enhanced ability of pronation allowed by modified joints. However, the powerful forces resulting from utilization of the paddles may have sometimes resulted in bone damage, as evidenced by a M. hoffmannii ilium with great separation damage from the bone's head to stem likely caused by frequent shearing forces at the articulation joint.[43]
The tissue structure of Mosasaurus bones suggests that it had a metabolic rate much higher than modern squamates and its basal metabolic rate was between the leatherback sea turtle' and the ichthyosaurs and plesiosaurs.[72] In order to keep up with its high metabolic requirements, Mosasaurus was likely endothermic and maintained a constant temperature of warm blood independent of the external environment. There is no direct evidence specific to the genus, but studies on the biochemistry of related endothermic mosasaur genera concluded that endothermy was likely present in all mosasaurs. Endothermy, which is unique among squamates, would have given several advantages to Mosasaurus, including increased stamina when foraging larger areas and pursuing prey.[73] It may have also been a factor that allowed Mosasaurus to thrive in the subpolar climates of locations such as Antarctica,[73][74][75][76] where ocean temperatures during the Maastrichtian had the lowest annual averages of around 4–5 °C (39–41 °F) with sea surface temperatures possibly dropping to below 0 °C (32 °F).[74]
Sensory functions
Mosasaurus had relatively large eye sockets[43] with large sclerotic rings occupying much of the socket's diameter,[36] suggesting that it had good vision. The eye sockets are located at the sides of the skull, which prevented good binocular vision but alternatively allowed excellent processing of a two-dimensional environment. This may have been particularly useful for Mosasaurus, which likely lived near the surface in open waters where three-dimensional environments are virtually nonexistent.[43]
Brain casts made from the cranium of Mosasaurus skulls show that the olfactory bulb and vomeronasal organ, which control the function of smell, are poorly developed and lack a number of components in M. hoffmannii; this indicates that the species had an extremely poor sense of smell. In M. lemonnieri, these olfactory organs, although still small, are better developed and has some of the components that M. hoffmannii lack, suggesting that it had a more improved sense of smell. The lack of strength in smell suggests that olfaction was not particularly important in Mosasaurus; instead, other senses like a well-developed sense of vision may have been more useful for the mosasaur.[43]
Intraspecific combat
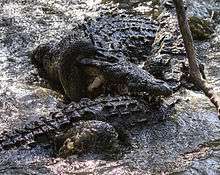
There is direct evidence that Mosasaurus engaged in aggressive and lethal intraspecific combat with other individuals of its kind. One particular fossil is of a partial M. conodon skeleton consisting of multiple cuts, breaks, and punctures on various bones, particularly in the posterior portions of the skull and neck, and a tooth from another M. conodon individual piercing through the quadrate bone. No injuries on the fossil show signs of healing, signifying that the mosasaur was killed by its attacker through a fatal blow in the skull.[77] Another direct example is of a M. missouriensis skeleton with a tooth from another M. missouriensis embedded in the lower jaw underneath the eye. In this case, there were signs of healing around the wound, signifying that the victim survived the attack.[78] There are multiple other known fossils of Mosasaurus skulls that show signs of severe injuries, some leading to infections and likely fatal, that were likely perpetrated by an attack by another Mosasaurus, although another possible explanation for some of these injuries included attempted biting on hard turtle shells. Lingham-Soliar suggests that if these injuries were indeed the result of an intraspecific attack, then it is notable that many of the injuries are concentrated in the skull. Modern crocodiles commonly attack other crocodiles by grappling their opponent's snout using their jaws, and it has been hypothesized that the concentration of such injuries in the skull indicates that Mosasaurus also employed snout-grappling during intraspecific combat. Another notability is that many of the fossils with injuries possibly attributable to intraspecific combat are of juvenile or sub-adult Mosasaurus, giving the possibility that attacks on smaller, weaker individuals may have been more common.[79] However, the attacking mosasaurs of the M. conodon and M. missouriensis specimens were likely similar in size with the victims.[77][78] Some scientists have speculated the possibility that Mosasaurus may have even occasionally engaged in cannibalism as a result of intraspecific aggression.[80]
Feeding
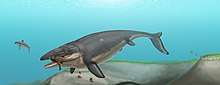
While there is little knowledge of the feeding habits of Mosasaurus, paleontologists generally agree that it was likely an active predator that preyed on a variety of marine animals.[43][46] It is unlikely that Mosasaurus was a scavenger as it had a poor sense of smell. Mosasaurus was among the largest marine animals of its time,[43] and with its large robust cutting teeth, scientists believe that larger members of the genus would have been able to handle virtually anything.[46] Lingham-Soliar (1995) suggested that Mosasaurus had a rather savage feeding behavior as demonstrated by large tooth marks on scutes of the giant sea turtle Allopleuron hoffmanni and fossils of re-healed fractured jaws in M. hoffmannii.[43] Fauna that was likely preyed on by the mosasaur include bony fish, sharks, cephalopods, birds, and marine reptiles such as other mosasaurs[46] and turtles. M. hoffmannii likely hunted near the ocean surface as an ambush predator, using its large two-dimensionally adapted eyes to more effectively spot and capture prey.[43] Chemical and structural data in the fossils of M. lemonnieri and M. conodon suggests that they may have also hunted at deeper waters.[81]
Carbon isotope studies on fossils of multiple M. hoffmannii individuals have found extremely low values of δ13C, and are the lowest in all mosasaurs. There are several implications for δ13C levels in the feeding ecology of mosasaurs. The relationship between δ13C levels in mosasaurs and their trophic levels are negatively correlated; mosasaurs with lower δ13C values tended to occupy higher trophic levels. One factor for this is dietary; a diet in prey with high lipid contents such as sea turtles and other large marine reptiles can lower δ13C values. With M. hoffmannii's low δ13C levels, this suggests that it likely fed on such prey and reinforces its likely position as an apex predator.[46]
Currently, there is only one known example of a Mosasaurus preserved with stomach contents: a well-preserved partial skeleton of a small M. missouriensis dated about 75 million years ago (Ma). Analysis of its stomach contents have found remains of a 1 meter (3.3 ft) long fish. This fish is much longer than the length of the mosasaur's skull, which was measured at 66 centimeters (26 in) in length, confirming that M. missouriensis was macrophagous and consumed prey larger than its head by dismembering and consuming bits at a time. The presence of other large mosasaurs which specialized in robust prey coexisting with the species strongly suggests that M. missouriensis likely specialized more on cutting-based prey (prey best fed on by teeth that have adapted to cutting) to ensure niche partitioning.[9]
There is a possibility that Mosasaurus may have taught their offspring how to hunt, as supported by a fossil nautiloid Argonautilus catarinae with bite marks from two conspecific mosasaurs, one being from a juvenile and the other being from an adult. The positioning of both bite marks are at the direction that the nautiloid would have been facing, indicating that it was incapable of escaping and was thus already sick or dead during the attacks; it is possible that this phenomenon was from a parent mosasaur teaching its offspring that cephalopods were an alternate source of prey and how to hunt one. An alternate explanation is that the bite marks are from one individual mosasaur that lightly bit the nautiloid at first, then proceeded to bite again with greater force; but differences in tooth spacing between both bites indicate different jaw sizes, which makes the first hypothesis more likely. Analysis of the tooth marks have concluded that the mosasaurs were either Mosasaurus or Platecarpus.[82]
Life history
It is likely that Mosasaurus was viviparous (giving live birth) like modern mammals today. There is no direct evidence specific for the genus, but there is such that at least a number of mosasaurs gave live birth;[83] examples include a skeleton of a pregnant basal mosasauroid Carsosaurus marchesetti,[83] a Plioplatecarpus primaevus fossil associated with fossils of two mosasaur embryos,[84] and fossils of newborn Clidastes from pelagic deposits.[83] Such fossil records, along with a total absence of any evidence suggesting external egg-based reproduction, indicates the likeliness of Mosasaurus viviparity.[83][84] Microanatomical studies on bones of juvenile Mosasaurus and related genera have found their bone structures are comparable to adults and did not exhibit bone mass increase (which is associated with a lifestyle in shallow water), signifying that Mosasaurus were already efficient swimmers and lived a fully functional lifestyle in open water at a very young age.[85] These structures indicate that Mosasaurus was likely born precocial in pelagic settings and did not utilize nursery areas to birth and raise young.[83][85] However, a number of localities in Europe and South Dakota have yielded concentrated assemblages of juvenile M. hoffmannii, M. missouriensis and/or M. lemonnieri. These localities are solely shallow ocean deposits, suggesting that juvenile Mosasaurus may have still utilized shallow waters.[86]
Paleopathology
With its evidently "savage" lifestyle,[79] there are a number of known fossils of M. hoffmannii that exhibit severe physically-inflicted damage. Two specimens from the Royal Belgian Institute of Natural Science cataloged as IRSNB R25 and IRSNB R27 with fractures and other pathologies in their dentary bones have been described by Lingham-Soliar in a 2004 study. The specimen IRSNB R25 preserves a complete fracture near the sixth tooth socket. Extensive amounts of bony callus almost overgrowing the tooth socket are present around the fracture along with various osteolytic cavities, abscess canals, foramina in a trigeminal nerve, and inflamed erosions signifying severe bacterial infection. There are two finely ulcerated scratches on the bone callus, which may have been developed as part of the healing process. Specimen IRSNB R27 has two fractures: one has almost fully healed and the other is an open fracture with nearby teeth broken off, which is likely associated with the dentary fracture. The fracture is covered with a nonunion formation of bony callus with shallow scratch marks and a large pit connected to an abscess canal. Both specimens show signs of deep bacterial infection alongside the fractures; some bacteria may have spread to nearby damaged teeth and caused tooth decay, which may have entered deeper tissue from prior post-traumatic or secondary infections. However, the conditions of the dentaries anterior to the fractures in both specimens are in good condition, indicating that the arteries and trigeminal nerves had not been damaged; if they were, those areas would have necrotized due to a lack of blood. The dentaries' condition suggests that the individuals may have had an efficient process of immobilization of the fracture during healing, which likely helped prevent damage to vital blood vessels and nerves. This, along with signs of healing, also signifies that the fractures were not imminently fatal. The cause of these injuries cannot be determined for certain, but two possibilities exist: One possibility may have been collateral damage from a bite on a hard surface such as a turtle shell, which would have caused intensified stress on the jawbones; another possibility is damage inflicted by another individual during intraspecific combat. The pit in IRSNB R27 has been described to resemble a tooth mark, which gives the possibility that such was the location of an attack by another mosasaur.[79]
In 2006, paleontologists led by Anne Schulp of Utrecht University published a study describing a fossil quadrate of M. hoffmannii with a massive chronic infection. The bone was extensively damaged, had multiple unnatural openings, and an estimated half-liter of bone tissue destroyed. It is likely that this was the result of a severe case of osteomyelitis initiated by septic arthritis, which progressed to the point that a large portion of the quadrate was reduced to voids of abscess. Extensive amounts of bone reparative tissue were also present, suggesting that the infection and subsequent healing process may have progressed for a few months. This level of bone infection would have likely been tremendously painful and severely hampered the mosasaur's ability to use its jaws. The location of the infection may likely have also interfered with respiration. Considering that the individual was able to survive such conditions for an extended period of time, it is likely that it switched to a foraging-type diet subsidizing on soft-bodied prey such as squid that could be swallowed whole to minimize jaw usage. The cause of the infection currently remains speculative, but if it were a result of an intraspecific attack then it is possible that one of the openings on the quadrate may have been the point of entry for an attacker's tooth from which the infection entered.[80]
Avascular necrosis has been reported by many studies to be invariably present in M. lemonnieri and M. conodon.[87][46][88] This condition is a common result of decompression illness, caused by bone damage from interference by formation of gaseous nitrogen bubbles that were produced from inhaled air decompressed during deep or repetitive diving. This indicates that both Mosasaurus species may have either been frequent deep-divers or repetitive divers. Paleontologist Agnete Weinreich Carlsen of the University of Copenhagen commented that it would be frugal to consider the appearance of such conditions being due to non-adaptation in the animal's original state, as fossils of other mosasaurs that also invariably suffer avascular necrosis show evidence of developed eardrums that protected itself from rapid changes in pressure.[88]
Paleoecology
Distribution, ecosystem, and ecological impact
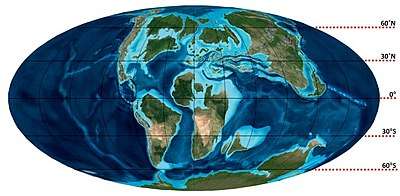
Excluding the Pacific species unassessed by Street and Caldwell (2017) and identified as separate genera in Street (2016), Mosasaurus was a transatlantic mosasaur with its fossils having been found in deposits at or nearby both sides of the Atlantic Ocean. These localities include the Midwestern and East Coast of the United States, Canada, Europe, Turkey, Russia, the Levant, the African coastline from Morocco to South Africa, Brazil, Argentina, and Antarctica.[5][75][89] During the Late Cretaceous, they made up the three seaways inhabited by Mosasaurus: the Atlantic Ocean, Western Interior Seaway, and Mediterranean Tethys.[89] Multiple oceanic climates encompass the seaways including tropical, subtropical, temperature, and subpolar climates.[89][90][91] The wide range of oceanic climates yield a large diversity of fauna that coexisted with Mosasaurus.
Mediterranean Tethys
The Mediterranean Tethys during the Maastrichtian was located in what is now Europe, Africa, and the Middle East. In recent studies, the confirmation of paleogeographical affinities extends this range to areas across the Atlantic including Brazil and the East Coast state of New Jersey. It is geographically subdivided into two biogeographic provinces that respectively include the northern and southern Tethyan margins. From an ecological view, the two mosasaurs Mosasaurus and Prognathodon appear to be the dominant taxa in the entire seaway, being very widespread and ecologically diversified throughout the Mediterranean Tethys. The northern Tethyan margin is located around the paleolatitudes of 30-40°N, consisting of what is now the European continent, Turkey, and New Jersey. At the time, Europe was a scattering of islands with most of the modern continental landmass being underwater. The northern Tethyan margin provides a warm-temperate climate that was dominated by mosasaurs and sea turtles. M. hoffmannii and Prognathodon sectorius were the dominant species in this province.[89] However, other Mosasaurus species such as M. lemonnieri have been found to be the dominant species in certain areas such as Belgium, where its occurrences greatly outnumber that of other large mosasaurs.[36] Other mosasaurs that have been found in the European side of the northern Tethyan margin include smaller mosasaurs such as Halisaurus, Plioplatecarpus, and Platecarpus; the shell-crusher Carinodens; and larger mosasaurs of similar trophic levels including Tylosaurus bernardi and four other species of Prognathodon. Sea turtles such as Allopleurodon hoffmanni and Glyptochelone suickerbuycki also dominated the area and other marine reptiles including undetermined elasmosaurs have been occasionally known. Marine reptile assemblages in the New Jersey region of the province are generally equivalent with those in Europe; mosasaur fauna are quite similar but exclude M. lemonnieri, Carinodens, Tylosaurus, and certain species of Halisaurus and Prognathodon and the exclusively feature M. conodon and some species of Halisaurus and Prognathodon.[89] Many species of sharks such as Squalicorax, Cretalamna, Serratolamna, and sand sharks,[92] as well as bony fish such as Cimolichthys, the saber-toothed herring Enchodus, and the swordfish Protosphyraena are represented in the northern Tethyan margin.[93]
The southern Tethyan margin is located along the equator between 20°N and 20°S, resulting in warmer tropical climates as typical in the province. Located around what is now Africa, Arabia, the Levant, and Brazil, seabeds bordering the cratons in Africa and Arabia provided vast shallow marine environments. These environments were also dominated by mosasaurs and marine side-necked turtles. Of the mosasaurs, Globidens phosphaticus is the characteristic species of the southern province; in the African and Arabian domain, Halisaurus arambourgi and Platecarpus' ptychodon were also the dominant mosasaurs.[89] Mosasaurus was not well-represented: the distribution of M. beaugei was restricted to Morocco and Brazil and isolated teeth from Syria suggested a possible presence of M. lemonnieri, although M. hoffmannii has some presence throughout the province.[5][89] Other mosasaurs from the southern Tethyan margin include the enigmatic Goronyosaurus, the shell-crushers Igdamanosaurus and Carinodens, Eremiasaurus, four other species of Prognathodon, and various other species of Halisaurus. Other marine reptiles such as the marine monitor lizard Pachyvaranus and sea snake Palaeophis are known there. Aside from Zarafasaura in Morocco, plesiosaurs were scarce. As a tropical area, bony fish such as Enchodus and Stratodus and various sharks were common throughout the southern Tethyan margin.[89]
Western Interior Seaway

Many of the earliest fossils of Mosasaurus were found in Campanian deposits in North America, including what was once the Western Interior Seaway, an inland sea that flowed through what is now the central United States and Canada and connected the Arctic Ocean to the modern-day Gulf of Mexico. The region was rather shallow for a seaway and had a depth of about 800–900 meters (2,600–3,000 ft) below the surface at its deepest.[94] Extensive drainage from the two neighboring Appalachia and Laramidia continents brought in vast amounts of sediments, and together with the formation of a nutrient-rich deepwater mass from the mixing of continental freshwater, Arctic waters from the north, and warmer saline Tethyan waters from the south, it created a warm and highly-productive seaway that supported a rich diversity of marine life.[24][95][96] In fact, many of the most famous marine assemblages of the Late Cretaceous are from deposits in the Western Interior Seaway.[24] However, fossil assemblages throughout these deposits suggest a complete faunal turnover by the time M. missouriensis and M. conodon appeared, and that the presence of Mosasaurus in the Western Interior Seaway had a profound impact in the restructuring of marine ecosystems.[97] The biogeography of the region is generally subdivided into two Interior Subprovinces characterized by different climates and faunal structures, which border around modern-day Kansas. The oceanic climate of the Northern Interior Subprovince was likely a cool temperate one, while the Southern Interior Subprovince had warm temperate to subtropical climates.[90] The faunal structure of both provinces prior to the appearance of Mosasaurus were generally much more diverse, and scientists have classified these periods of diversity as the Niobraran Age. During this age, the Northern Interior Subprovince was dominated by plesiosaurs, hesperornithid seabirds, and the mosasaur genus Platecarpus; and the Southern Interior Subprovince, which was much more diverse than the north in all groups, was dominated by sharks, turtles, and a large diversity of mosasaurs including Tylosaurus and Clidastes.[97][90]
The appearance of M. missouriensis and M. conodon in the Western Interior Seaway around 79.5 Ma marked the transition to the succeeding Navesinkan Age, which coincided with the collapse of the Niobraran order and a complete turnover of marine faunal structure.[97][98] In what is now modern-day Alabama within the Southern Interior Subprovince, most of the key genera including mosasaurs Clidastes, Tylosaurus, Globidens, Halisaurus, and Platecarpus and sharks such as Cretoxyrhina largely disappeared and were replaced by Mosasaurus.[97][99] The diversity of marine reptiles as a whole significantly declined and by then Mosasaurus dominated the entirety of the region, comprising around two-thirds of all mosasaur diversity with Plioplatecarpus and Prognathodon sharing the remaining third. The Northern Interior Subprovince also saw a restructuring of mosasaur assemblages by the beginning of the Navesinkan Age, characterized by the disappearance of mosasaurs like Platecarpus and their replacement by Mosasaurus and Plioplatecarpus.[97] However, Niobraran genera such as Tylosaurus,[100] Cretoxyrhina,[101] hesperornithids,[102] and plesiosaurs including elasmosaurs such as Terminonatator[103] and polycotylids like Dolichorhynchops[104] maintained their presence until around the end of the Campanian, during which the entire Western Interior Seaway started receding from the north.[24] Mosasaurus continued to be the dominant genus in the seaway until the end of the Navesinkan Age during the closure of the Cretaceous.[97] Although the appearance of Mosasaurus in the Western Interior Seaway marked a complete restructuring of marine communities centered around it, there were still many fauna that coexisted with Mosasaurus. These additional genera included sea turtles such as Protostega[99] and Archelon;[105] many species of sea birds including Baptornis,[102] Ichthyornis, and Halimornis; crocodilians such as Deinosuchus; and many genera of fish including sharks such as Cretalamna, Squalicorax, the goblin shark Scapanorhynchus, Pseudocorax, the sand tiger Odontaspis, Serratolamna, and the saw shark Ischyrhiza; and bony fish such as Enchodus, Protosphyraena, Stratodus, and the ichthyodectids Xiphactinus and Saurodon.[99][106]
Antarctica
Mosasaurus is known from Late Maastrichtian deposits in the Antarctic Peninsula, specifically the López de Bertodano Formation in Seymour Island.[75] This locality is estimated to have been located at around ~65°S latitude during the Maastrichtian. Being within the Antarctic polar circle, the Seymour Island locality likely provided a rather unique climate.[91] Chemical studies on oxygen-18 isotopes found in shells and benthic foraminifera have calculated intermediate-depth and deep-sea ocean temperatures at a mean average of 6 °C (43 °F) with fluctuations of up to 4–12 °C (39–54 °F) throughout the Maastrichtian; one of the same studies has also suggested that sea surface temperatures may have been colder, possibly dropping below freezing and forming sea ice at times.[74][107] Alternatively, a study using the MBT/CBT technique deriving data from cyclization and methylation processes in ancient bacterial membrane lipids yielded a slightly warmer temperature of 12 °C (54 °F) ±5 around 66 Ma. Nevertheless, these estimated climates characterize subpolar to cool temperate environments.[91]
At least two species of Mosasaurus have been described in Seymour Island, but the true number of species is unknown as remains are often fragmentary and specimens are described in open nomenclature. These species include one comparable with M. lemonnieri and another that appears to be closely related to M. hoffmannii. A number of Mosasaurus fossils known in the locality are considered too fragmentary to be identified to the species level. Nevertheless, the genus appears to be the most taxonomically diverse in the Maastrichtian Antarctica. Mosasaurus is not the only mosasaur from Seymour Island; at least four other genera have been found in similar or same deposits. These include Plioplatecarpus, the mosasaurines Moanasaurus and Liodon,[75] and the tylosaurine Kaikaifilu.[108] Prognathodon and Globidens are also expected to be present based on distribution trends of both genera, although conclusive fossils have yet to be found.[75] Other marine reptiles included elasmosaurid plesiosaurs such as Aristonectes and another indeterminable elasmosaurid unrelated to the former.[109] The fish assemblage of the López de Bertodano Formation was dominated by Enchodus and ichthyodectiformes, accounting for 21.95% and 45.6% of local fish diversity respectively. Of the remaining percentages, sand sharks made up 10.5%, the cow shark Notidanodon 6.8%, chimaeras 3.9%, saw sharks 2.7%, various other teleost fish 2.4%, and the remaining 6% were shared between other sharks such as Paraorthacodus, frilled sharks, Protosqualus, and Cretalamna.[110]
Habitat preference
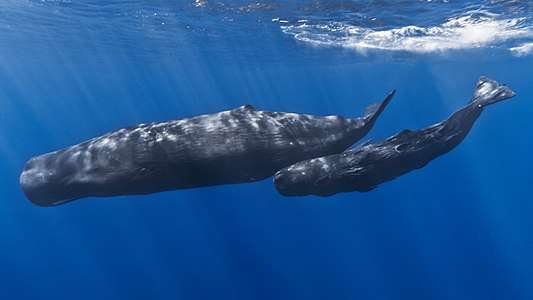
A traditional method of determining the habitat preference of fossil animals is by determining the habitat represented by the deposits they were from. Known fossils of Mosasaurus have typically been recovered from deposits that represented nearshore habitats during the Cretaceous period, with some fossils coming from deeper water deposits.[81][111] An early study on the habitat preference by Lingham-Soliar in 1995 elaborated on this, finding that Maastrichtian deposits in the Netherlands with M. hoffmannii occurrences, especially the Maastricht type locality, represented nearshore waters that were around 40–50 metres (130–160 ft) in depth. Changing temperatures and an abundance in marine life were characteristic of these localities. The morphological build of M. hoffmannii, nevertheless, was best adapted for a pelagic surface lifestyle. It had likely resided near the surface and exploited the rich marine assemblages provided by the locality.[43]
A more recently developing approach is through a biogeochemical one, Compared to the earlier method of measuring δ13C levels in the enamel of Mosasaurus teeth. Another known correlation with δ13C levels shows that δ13C typically depletes as the foraging habitat of the animal is farther from the shore, meaning that lower levels of the isotope can be correlated with feeding habitation in more open waters and vice-versa. This was tested on multiple Mosasaurus fossils in multiple studies which have yielded consistent results signifying that Mosasaurus fed in more offshore or open waters. However, it has been pointed out that measuring δ13C levels may not be the most accurate method of determining the preferred habitat of Mosasaurus.[111] This is because such isotope levels can also be partially determined by other factors in the animal's lifestyle. In M. hoffmannii, one such factor would be its diet[111] while the Bohr effect through diving behavior would have been another possible factor in M. lemonnieri and M. conodon.[81] As a result, isotope levels can misrepresent the actual habitat preferences of Mosasaurus due to such alterations by other factors.[111] As a solution, paleontologists T. Lynn Harrell Jr. and Alberto Perez-Huerta conducted a 2014 study that specifically examined the concentration ratios of neodymium, gadolinium, and ytterbium in M. hoffmannii fossils from Maastrichtian deposits in Alabama, a Mosasaurus specimen from the Campanian-age Demopolis Chalk, and a Mosasaurus fossil from the Hornerstown Formation in New Jersey. Previous studies have demonstrated that ratios in these three elements can act as a proxy for relative ocean depth of a fossil during early diagenesis without interference from biological processes, with each of the three elements signifying either shallow, deep, fresh, or highly saline waters. The rare earth element ratios were found to be very consistent throughout most of the examined Mosasaurus fossils (indicating consistent habitat preference), which were clustered towards a ratio representing offshore habitats with ocean depths between or deeper than 50–150 metres (160–490 ft). A few outliers existed that instead represented shallower waters 50 metres (160 ft) deep or less.[111]
Interspecific competition
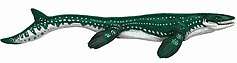
Mosasaurus was contemporaneous with other large predatory mosasaurs that were also considered apex predators, most prominent among them being the tylosaurines and Prognathodon.[43][46] Tylosaurus bernardi, the only surviving species of the genus during the Maastrichtian, measured up to 12.2 meters (40 ft) in length[112] while the largest coexisting species of Prognathodon such as P. saturator grew longer than 12 meters (39 ft).[46] The diets of these three types of mosasaurs converged as they all prey on similar animals such as on other marine reptiles.[9][43][46]
A study published in 2013 led by Schulp specifically tested how mosasaurs such as M. hoffmannii and P. saturator were able to coexist in the same localities through δ13C analysis. The scientists utilized an interpretation that differences in isotope values can help explain the level of resource partitioning due to the contributions of multiple environmental factors such as lifestyle, diet, and habitat preference in determining such. Comparisons between the δ13C levels in multiple teeth of M. hoffmannii and P. saturator from Maastricht Formation showed that while there was some convergence in isotopic levels between certain specimens, the average δ13C values between the two species were generally different. This is one indication of niche partitioning, where the two mosasaur genera likely foraged in different habitats or had different specific diets in order to coexist without direct competitive conflict as evidenced by the different biological factors driving their differing δ13C values. The morphological builds of the two species help add to the context of this finding. The teeth of P. saturator are much more robust than those in M. hoffmannii and are specifically equipped for preying on robust prey like turtles. While M. hoffmannii also preyed on turtles, its teeth were built to handle a wider range of prey that may be less suited for P. saturator.[46] Another case of presumed niche partitioning between Mosasaurus and Prognathodon from the Bearpaw Formation in Alberta has been examined in a 2014 study led by Takuya Konishi of the University of Cincinnati. The study found a dietary divide between M. missouriensis and Prognathodon overtoni based on stomach contents. Stomach contents of P. overtoni have included turtles and ammonites, which gives another example of a diet that specialized in harder prey. In contrast, M. missouriensis has been found with stomach contents consisting of fish indicative of a diet that specialized in softer prey. It was hypothesized that these adaptations were developed specifically to ensure resource partitioning between the two types of mosasaurs.[9]
However, this evidently did not entirely prevent competitive engagement. As with intraspecific combat amongst Mosasaurus individuals, there is also evidence of aggressive interspecific combat between Mosasaurus and other large mosasaur species, as shown from a fossil skull of a subadult M. hoffmannii with skull fractures caused by a massive concentrated blow to the braincase dealt from a ramming-attack by a Tylosaurus bernardi. This sort of attack has been compared to the defensive behavior of bottlenose dolphins using their beaks to kill or repel lemon sharks, and it has been speculated that T. bernardi possibly dealt the offensive attack via an ambush on an unsuspecting Mosasaurus. With the enormous known diets of Tylosaurus, it has been loosely implied that this may have been motivated for food or territorial defense.[113]
Extinction
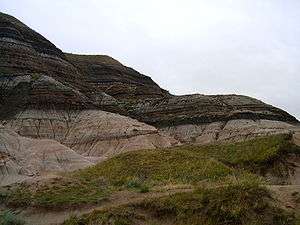
By the end of the Cretaceous, mosasaurs like Mosasaurus were at a height of radiation, and their extinction was a sudden and unexpected event.[43] The youngest fossils of Mosasaurus occur up to the Cretaceous-Paleogene boundary (K-Pg boundary) and its demise was a result of the Cretaceous-Paleogene extinction event that also wiped out the dinosaurs. Localities where Mosasaurus fossils have been found at least 15 meters (49 ft) below the boundary or less include but are not limited to the Maastricht Formation, the Davutlar Formation in Turkey, the Jagüel Formation in Argentina, Stevns Klint in Denmark, Seymour Island, and Missouri.[114] Prior to the boundary event, global sea levels dropped, which drained the continents of their nutrient-rich seaways and altered circulation and nutrient patterns, reducing the number of available habitats for Mosasaurus. The genus endured by accessing new habitats in more open waters.[115][116]
Mosasaurus fossils have been found within the K-Pg boundary itself between the Paleocene Clayton Formation and Cretaceous Owl Creek Formation in southeastern Missouri. Vertebra fossils from the layer were found with post-mortem fractures and the deposition of the layer itself was likely the result of a tsunamite, alternatively nicknamed the "Cretaceous cocktail deposit"; it was formed as a result of a combination of catastrophic seismic and geological disturbances, mega-hurricanes, and giant tsunamis as direct consequences of the impact of the Chicxulub asteroid. Aside from the physical kinetic destruction of these events, the impact also had subsequent environmental reverberations like blocking out sunlight that led to a collapse of marine food webs. Any Mosasaurus that may have survived the immediate cataclysms by taking refuge in deeper waters would have eventually died out due to starvation from a total loss of prey structure.[115]
One enigmatic occurrence is of Mosasaurus fossils found in the Hornerstown Formation, a deposit that is typically dated to be from the Paleocene Danian age, which was immediately after the Maastrichtian age. The discoveries have been found with an association of fossils of Squalicorax, Enchodus, and various ammonites within a uniquely fossil-rich bed at the base of the Hornerstown Formation known as the Main Fossiliferous Layer. This does not mean that Mosasaurus and its associated fauna may have survived the K-Pg extinction; there are several possible explanations as to why these animals have been found in nominally Cenozoic deposits. One argument proposes the fossils actually originated from an earlier Cretaceous deposit that was reworked into the Paleocene formation during its early deposition. Evidence of reworking typically comes from fossils that are worn down due to further erosion during its exposure at the time of its redeposition. Many of the Mosasaurus fossils from the Main Fossiliferous Layer consist of isolated bones that are commonly abraded and worn; however, the layer also yielded fresher Mosasaurus remains. Another explanation suggests that the Main Fossiliferous Layer is a Maastrichtian time-averaged remanié deposit, which means that it originated from a Cretaceous deposit with little sedimentation and was gradually winnowed into the overlying deposits. A third hypothesis proposes a representation of a lag deposit of Cretaceous sediments that were forced out by a strong impact event such as a tsunami and was subsequently refilled with Cenozoic fossils.[2]
See also
Paleontology portal
Notes
- The exact year is not fully certain due to multiple contradicting claims. An examination of existing historical evidence by Pieters et al., (2012) suggested that the most accurate date would be on or around 1780.[14] More recently, Limburg newspapers reported in 2015 that Ernst Homburg of the University of Maastricht discovered a Liège magazine issued in the October of 1778 reporting in detail a recent discovery of the second skull.[15]
- A more literal translation would be "the great fossil animal of the quarries of Maastricht".
- hoffmannii was the original spelling used by Mantell, ending with -ii. Later authors began to drop the final letter and spelled it as hoffmanni, as became the trend for specific epithets of similar structure in later years. However, recent scientists argue that the special etymological makeup of hoffmannii cannot be subjected to International Code of Zoological Nomenclature Articles 32.5, 33.4, or 34, which would normally protect similar respellings. This makes hoffmannii the valid spelling, although hoffmanni continues to be incorrectly used by many authors.[9]
- The identity of this foreign naturalist has not been recorded.[25]
- One specimen traditionally attributed to M. lemonnieri has serrate-like features in its cutting edges. However, scientists have expressed likeliness that this specimen belongs to a different species.[39]
- The number of prisms in M. conodon and number of lingual prisms in M. lemonnieri are uncertain.[41]
- This study was conducted on only one tooth and may not represent the exact durations of dentinogenesis in all Mosasaurus teeth.[51]
- The number of caudal vertebrae is not fully certain but at least ten vertebrae are known in an M. conodon tail and completely unknown in M. hoffmannii.[11]
- In the sense of English definition 2.
- As in independent of Street's thesis.[5]
- Some studies such as Madzia & Cau (2017) also place Prognathodon and Plesiotylosaurus within this tribe.[56]
References
- J. G. Ogg; L. A. Hinnov (2012). Cretaceous. The Geologic Time Scale 2012. pp. 793–853. doi:10.1016/B978-0-444-59425-9.00027-5. ISBN 9780444594259.
- William B. Gallagher (2005). "Recent mosasaur discoveries from New Jersey and Delaware, USA: stratigraphy, taphonomy and implications for mosasaur extinction". Netherlands Journal of Geosciences. 84 (3): 241–245. doi:10.1017/S0016774600021028.
- William B. Gallagher (1984). "Paleoecology of the Delaware Valley region, Part II: Cretaceous to Quaternary". The Mosasaur. 2 (1): 9–43.
- Christian C. Obasi; Dennis O. Terry Jr.; George H. Myer; David E. Grandstaff (2011). "Glauconite Composition and Morphology, Shocked Quartz, and the Origin of the Cretaceous(?) Main Fossiliferous Layer (MFL) in Southern New Jersey, U.S.A.". Journal of Sedimentary Research. 81 (1): 479–494. Bibcode:2011JSedR..81..479O. doi:10.2110/jsr.2011.42.
- Halle P. Street; Michael W. Caldwell (2017). "Rediagnosis and redescription of Mosasaurus hoffmannii (Squamata: Mosasauridae) and an assessment of species assigned to the genus Mosasaurus". Geological Magazine. 154 (3): 521–557. Bibcode:2017GeoM..154..521S. doi:10.1017/S0016756816000236.
- Joseph Leidy (1864). Cretaceous Reptiles of the United States. 14. Smithsonian Contributions to Knowledge. pp. 30–120.
- Halle P. Street (2016). A re-assessment of the genus Mosasaurus (Squamata: Mosasauridae) (PDF) (PhD). University of Alberta. doi:10.7939/R31N7XZ1K.
- T. L. Harrell Jr.; J. E. Martin (2014). "A mosasaur from the Maastrichtian Fox Hills Formation of the northern Western Interior Seaway of the United States and the synonymy of Mosasaurus maximus with Mosasaurus hoffmanni (Reptilia: Mosasauridae)". Netherlands Journal of Geosciences. 94 (1): 23–37. doi:10.1017/njg.2014.27.
- Takuya Konishi; Michael Newbrey; Michael Caldwell (2014). "A small, exquisitely preserved specimen of Mosasaurus missouriensis (Squamata, Mosasauridae) from the upper Campanian of the Bearpaw Formation, western Canada, and the first stomach contents for the genus". Journal of Vertebrate Paleontology. 34 (4): 802–819. doi:10.1080/02724634.2014.838573. S2CID 86325001.
- M. W. Caldwell; G. L. Bell Jr. (2005). "Of German princes and North American rivers: Harlan's lost mosasaur snout rediscovered". Netherlands Journal of Geosciences. 84 (3): 207–211. doi:10.1017/S0016774600020989.
- T. Ikejiri; S. G. Lucas (2014). "Osteology and taxonomy of Mosasaurus conodon Cope 1881 from the Late Cretaceous of North America". Netherlands Journal of Geosciences - Geologie en Mijnbouw. 94 (1): 39–54. doi:10.1017/njg.2014.28.
- M. van Marum (1790). Beschrijving der beenderen van den kop van eenen visch, gevonden in den St Pietersberg bij Maastricht, en geplaatst in Teylers Museum (in Dutch). 9. Verhandelingen Teylers Tweede Genootschap. pp. 383–389.
- Mike Everhart (1999). "Mosasaurus hoffmanni-The First Discovery of a Mosasaur?". Oceans of Kansas. Archived from the original on 2019-09-04. Retrieved 2019-11-06.
- Florence Pieters; Peggy G. W. Rompen; John W. M. Jagt; Nathalie Bardet (2012). "A new look at Faujas de Saint-Fond's fantastic story on the provenance and acquisition of the type specimen of Mosasaurus hoffmanni MANTELL, 1829". Bulletin de la Société Géologique de France. 183 (1): 55–65. doi:10.2113/gssgfbull.183.1.55.
- Vikkie Bartholomeus (2015-09-21). "Datum vondst mosasaurus ontdekt: in oktober 1778". 1Limburg (in Dutch). Archived from the original on 2020-03-07.
- Petrus Camper (1786). "Conjectures relative to the petrifactions found in St. Peter's Mountain near Maestricht". Philosophical Transactions of the Royal Society of London. 76 (2): 443–456. doi:10.1098/rstl.1786.0026. ISSN 2053-9223.
- Florence F. J. M. Pieters (2009). "Natural history spoils in the Low Countries in 1794/95: the looting of the fossil Mosasaurus from Maastricht and the removal of the cabinet and menagerie of stadholder William V". Napoleon's legacy: the rise of national museums in Europe, 1794-1830 (PDF). 27. Berlin: G+H Verlag. pp. 55–72. ISBN 9783940939111.
- Nathalie Bardet (2012). "The Mosasaur collections of the Muséum National d'Histoire Naturelle of Paris". Bulletin de la Société Géologique de France. 183 (1): 35–53. doi:10.2113/gssgfbull.183.1.35.
- Eric Mulder (2004). Maastricht Cretaceous finds and Dutch pioneers in vertebrate palaeontology. Royal Netherlands Academy of Arts and Sciences. pp. 165–176.
- Eric Mulder; Bert Theunissen (1986). "Hermann Schlegel's investigation of the Maastricht mosasaurs". Archives of Natural History. 13 (1): 1–6. doi:10.3366/anh.1986.13.1.1.
- Mark Evans (2010). "The roles played by museums, collections and collectors in the early history of reptile palaeontology". Geological Society, London, Special Publications. 343 (1): 5–29. Bibcode:2010GSLSP.343....5E. doi:10.1144/SP343.2. S2CID 84158087.
- Mike Everhart (2001). "The Goldfuss Mosasaur". Oceans of Kansas. Archived from the original on 2019-06-02. Retrieved 2019-11-10.
- "September 10, 1804". Journals of the Lewis & Clark Expedition. Archived from the original on 2019-11-11. Retrieved 2019-11-10.
- Michael J. Everhart (2017). Oceans of Kansas. Indiana University Press. pp. 24–263. ISBN 978-0253026323.
- James Ellsworth De Kay (1830). "On the Remains of Extinct Reptiles of the genera Mosasaurus and Geosaurus found in the secondary formation of New-Jersey; and on the occurrence of the substance recently named Coprolite by Dr. Buckland, in the same locality". Annals of the Lyceum of Natural History of New York. 3: 134–141.
- Heinrich Georg Bronn (1838). "Lethaea geognostica oder Abbildungen und Beschreibungen der für die Gebirgs-Formationen bezeichnendsten Versteinerungen" (in German). 2: 760. doi:10.5962/bhl.title.59080. Cite journal requires
|journal=
(help) - Eric W. A. Muler (1999). "Transatlantic latest Cretaceous mosasaurs (Reptilia, Lacertilia) from the Maastrichtian type area and New Jersey". Geologie en Mijnbouw. 78 (3/4): 281–300. doi:10.1023/a:1003838929257.
- Richard Harlan (1834). "Notice of the Discovery of the Remains of the Ichthyosaurus in Missouri, N. A.". Transactions of the American Philosophical Society. 4: 405–408. JSTOR 1004839.
- Richard Harlan (1839). "Notice of the discovery of Basilosaurus and Batrachotherium". Proceedings of the Geological Society of London. 3: 23–24.
- Richard Harlan (1839). "(Letter regarding Basilosaurus and Batrachotherium)". Bulletin de la Société Géologique de France (in French). 1 (10): 89–90.
- Mark Witton (2019). "The science of the Crystal Palace Dinosaurs, part 2: Teleosaurus, pterosaurs and Mosasaurus". Mark Witton.com. Archived from the original on 2019-06-03.
- "Mosasaurus". Friends of the Crystal Palace Dinosaurs. 2020. Archived from the original on 2020-06-18.
- Edward Drinker Cope (1881). "A new species of Clidastes from New Jersey". American Naturalist. 15: 587–588.
- Ben Creisler (2000). "Mosasauridae Translation and Pronunciation Guide". Dinosauria On-line. Archived from the original on 2008-05-02.
- Louis Dollo (1889). "Première note sur les Mosasauriens de Mesvin". Bulletin de la Société belge de géologie, de paléontologie et d'hydrologie (in French). 3: 271–304. ISSN 0037-8909.
- Theagarten Lingham-Soliar (2000). "The Mosasaur Mosasaurus lemonnieri (Lepidosauromorpha, Squamata) from the Upper Cretaceous of Belgium and The Netherlands". Paleontological Journal. 34 (suppl. 2): S225–S237.
- Pablo Gonzalez Ruiz; Marta S. Fernandez; Marianella Talevi; Juan M. Leardi; Marcelo A. Reguero (2019). "A new Plotosaurini mosasaur skull from the upper Maastrichtian of Antarctica. Plotosaurini paleogeographic occurrences". Cretaceous Research. 103 (2019): 104166. doi:10.1016/j.cretres.2019.06.012.
- Dale A. Russell (1967). Systematics and morphology of American mosasaurs (PDF). 23. Bulletin of the Peabody Museum of Natural History. pp. 1–124.
- Daniel Madzia (2019). "Dental variability and distinguishability in Mosasaurus lemonnieri (Mosasauridae) from the Campanian and Maastrichtian of Belgium, and implications for taxonomic assessments of mosasaurid dentitions". Historical Biology: 1–15. doi:10.1080/08912963.2019.1588892.
- Nathalie Bardet; Xabier Pereda Suberbiola; Stephane Jouve; Estelle Bourdon; Peggy Vincent; Alexandra Houssaye; Jean-Claude Rage; N.-E Jalil; B. Bouya; M. Amaghzaz (2010). "Reptilian assemblages from the latest Cretaceous – Palaeogene phosphates of Morocco: from Arambourg to present time". Historical Biology. 22 (1–3): 186–199. doi:10.1080/08912961003754945.
- Nathalie Bardet; Xabier Pereda Suberbiola; Mohamed Iarochene; Fatima Bouyahyaoui; Baadi Bouya; Mbarek Amaghzaz (2004). "Mosasaurus beaugei Arambourg, 1952 (Squamata, Mosasauridae) from the Late Cretaceous phosphates of Morocco". Geobios. 37 (2004): 315–324. doi:10.1016/j.geobios.2003.02.006.
- D.V. Grigoriev (2014). "Giant Mosasaurus hoffmanni (Squamata, Mosasauridae) from the Late Cretaceous (Maastrichtian) of Penza, Russia" (PDF). Proceedings of the Zoological Institute RAS. 318 (2): 148–167.
- Theagarten Lingham-Soliar (1995). "Anatomy and functional morphology of the largest marine reptile known, Mosasaurus hoffmanni (Mosasauridae, Reptilia) from the Upper Cretaceous, Upper Maastrichtian of The Netherlands". Philosophical Transactions of the Royal Society B. 347 (1320): 155–172. Bibcode:1995RSPTB.347..155L. doi:10.1098/rstb.1995.0019.
- Fedrico Fanti; Andrea Cau; Alessandra Negri (2014). "A giant mosasaur (Reptilia, Squamata) with an unusually twisted dentition from the Argille Scagliose Complex (late Campanian) of Northern Italy". Cretaceous Research. 49 (2014): 91–104. doi:10.1016/j.cretres.2014.01.003.
- Louis Dollo (1892). "Nouvelle note sur l'osteologie des mosasauriens". Bulletin de la Société belge de géologie, de paléontologie et d'hydrologie (in French). 6: 219=259. ISSN 0037-8909.
- A.S. Schulp; H.B. Vonhof; J.H.J.L. van der Lubbe; R. Janssen; R.R. van Baal (2013). "On diving and diet: resource partitioning in type-Maastrichtian mosasaurs". Netherlands Journal of Geosciences. 92 (2–3): 165–170. doi:10.1017/S001677460000010X.
- Anne S. Schulp; Michael J. Polcyn; Octavio Mateus; Louis L. Jacobs; Maria Lusia Morais; Tatiana da Silva Tavares (2006). "New mosasaur material from the Maastrichtian of Angola, with notes on the phylogeny, distribution, and paleoecology of the genus Prognathodon" (PDF). Publicaties van Het Natuurhistorisch Genootschap in Limburg: 57–67. ISSN 0374-955X.
- Clint A. Boyd (2017). "A New Addition to the Cretaceous Seaway of North Dakota" (PDF). Geo News. Vol. 44 no. 1. North Dakota Geological Society. pp. 20–23.
- Mike Everhart (2001). "Samuel Wilson's Mosasaurus horridus". Oceans of Kansas. Retrieved 2019-11-23.
- Michael W. Caldwell (2007). "Ontogeny, anatomy and attachment of the dentition in mosasaurs (Mosasauridae: Squamata)". Zoological Journal of the Linnean Society. 149 (4): 687–700. doi:10.1111/j.1096-3642.2007.00280.x.
- Anusuya Chinsamy; Cemal Tunoǧlu; Daniel B. Thomas (2012). "Dental microstructure and geochemistry of Mosasaurus hoffmanni (Squamata: Mosasauridae) from the Late Cretaceous of Turkey". Bulletin de la Société Géologique de France. 183 (2): 85–92. doi:10.2113/gssgfbull.183.2.85.
- Johan Lindgren; Michael J. Polcyn; Bruce A. Young (2011). "Landlubbers to leviathans: evolution of swimming in mosasaurine mosasaurs". Paleobiology. 37 (3): 445–469. doi:10.1666/09023.1. S2CID 85165085.
- Halle P. Street; Michael W. Caldwell (2016), A reexamination of Mosasaurini based on a systematic and taxonomic revision of Mosasaurus (PDF). 5th Triannual Mosasaur Meeting- a global perspective on Mesozoic marine amonites. May 16–20. Abstract. p. 43-44.
- Halle P. Street. "Reassessing Mosasaurini based on a systematic revision of Mosasaurus". Vertebrate Anatomy Morphology Palaeontology. 4: 42. ISSN 2292-1389.
- Aaron R. H. LeBlanc; Michael W. Caldwell; Nathalie Bardet (2012). "A new mosasaurine from the Maastrichtian (Upper Cretaceous) phosphates of Morocco and its implications for mosasaurine systematics". Journal of Vertebrate Paleontology. 32 (1): 82–104. doi:10.1080/02724634.2012.624145. S2CID 130559113.
- Daniel Madzia; Andrea Cau (2017). "Inferring 'weak spots' in phylogenetic trees: application to mosasauroid nomenclature". PeerJ. 5: e3782. doi:10.7717/peerj.3782. PMC 5602675. PMID 28929018.
- Michael W. Caldwell (2012). "A challenge to categories: "What, if anything, is a mosasaur?"". Bulletin de la Societe Geologique de France. 183 (1): 17–34doi=10.2113/gssgfbull.183.1.7. doi:10.2113/gssgfbull.183.1.7.
- Alessandro Palci. On the Origin and Evolution of the Ophidia (PDF) (PhD). University of Alberta. doi:10.7939/R3NG4H314.
- Jack L. COnrad (2008). "Phylogeny And Systematics Of Squamata (Reptilia) Based On Morphology". Bulletin of the American Museum of Natural History. 310: 1–182. doi:10.1206/310.1.
- Charles Lewis Camp (1923). "Classification of the Lizards". Bulletin of the American Museum of Natural History. 48 (11): 289–481. hdl:2246/898.
- Michael S. Y. Lee (1997). "The Phylogeny of Varanoid Lizards and the Affinities of Snakes". Philosophical Transactions: Biological Sciences. 352 (1349): 53–91. Bibcode:1997RSPTB.352...53L. JSTOR 56451.
- Jacques A. Gauthier; Maureen Kearney; Jessica Anderson Maisano; Olivier Rieppel; Adam D.B. Behlke (2012). "Assembling the Squamate Tree of Life: Perspectives from the Phenotype and the Fossil Record". Bulletin of the Peabody Museum of Natural History. 53 (1): 3–308. doi:10.3374/014.053.0101.
- John J. Wiens; Caitlin A. Kuczynski; Ted Townsend; Tod W. Reeder; Daniel G. Mulcahy; Jack W. Sites Jr. (2012). "Combining Phylogenomics and Fossils in Higher-Level Squamate Reptile Phylogeny: Molecular Data Change the Placement of Fossil Taxa" (PDF). Systematic Biology. 59 (6): 674–88. doi:10.1093/sysbio/syq048. PMID 20930035.
- Tod W. Reeder; Ted M. Townsend; Daniel G. Mulcahy; Brice P. Noonan; Perry L. Wood, Jr.; Jack W. Sites, Jr.; John J. Wiens (2015). "Integrated Analyses Resolve Conflicts over Squamate Reptile Phylogeny and Reveal Unexpected Placements for Fossil Taxa". PLOS ONE. 10 (3): e0118199. Bibcode:2015PLoSO..1018199R. doi:10.1371/journal.pone.0118199. PMC 4372529. PMID 25803280.
- Michael S. Y. Lee (2009). "Hidden support from unpromising data sets strongly unites snakes with anguimorph 'lizards'". Journal of Evolutionary Biology. 22 (6): 1308–1316. doi:10.1111/j.1420-9101.2009.01751.x. PMID 19490385.
- N. B. Ananjeva (2019). "Current State of the Problems in the Phylogeny of Squamate Reptiles (Squamata, Reptilia)". Biology Bulletin Reviews. 9 (2): 119–128. doi:10.1134/s2079086419020026.
- A. Alexander Pyron (2016). "Novel Approaches for Phylogenetic Inference from Morphological Data and Total-Evidence Dating in Squamate Reptiles (Lizards, Snakes, and Amphisbaenians)" (PDF). Systematic Biology. 66 (1): 38–56. doi:10.1093/sysbio/syw068. PMID 28173602. S2CID 3697004.
- Gorden L. Bell Jr. (1997). "A Phylogenetic Revision of North American and Adriatic Mosasauroidea". Ancient Marine Reptiles. Academic Press. pp. 293–332. doi:10.1016/b978-012155210-7/50017-x. ISBN 978-0-12-155210-7.
- Tiago R. Simões; Oksana Vernygora; Ilaria Paparella; Paulina Jimenez-Huidobro; Michael W. Caldwell (2017). "Mosasauroid phylogeny under multiple phylogenetic methods provides new insights on the evolution of aquatic adaptations in the group". PLOS ONE. 12 (5): e0176773. Bibcode:2017PLoSO..1276773S. doi:10.1371/journal.pone.0176773. PMC 5415187. PMID 28467456.
- D. V. Grigoriev (2013). "Redescription of Prognathodon lutugini (Squamata, Mosasauridae)" (PDF). Proceedings of the Zoological Institute RAS. 317 (3): 246–261.
- Theagarten Lingham-Soliar (1991). "Locomotion in mosasaurs". Modern Geology. 16: 229–248.
- Alexandra Houssaye; Johan Lindgren; Rodrigo Pellegrini; Andrew H. Lee; Damien Germain; Michael J. Polcyn (2013). "Microanatomical and Histological Features in the Long Bones of Mosasaurine Mosasaurs (Reptilia, Squamata) – Implications for Aquatic Adaptation and Growth Rates". PLOS ONE. 8 (10): e76741. Bibcode:2013PLoSO...876741H. doi:10.1371/journal.pone.0076741. PMC 3797777. PMID 24146919.
- T. Lynn Harrell Jr.; Alberto Pérez‐Huerta; Celina A. Suarez (2016). "Endothermic mosasaurs? Possible thermoregulation of Late Cretaceous mosasaurs (Reptilia, Squamata) indicated by stable oxygen isotopes in fossil bioapatite in comparison with coeval marine fish and pelagic seabirds". Palaeontology. 59 (3): 351–363. doi:10.1111/pala.12240.
- Vanessa C. Bowman; Jane E. Francis; James B. Riding (2013). "Late Cretaceous winter sea ice in Antarctica?" (PDF). Geology. 41 (12): 1227–1230. Bibcode:2013Geo....41.1227B. doi:10.1130/G34891.1.
- James E. Martin (2006). "Biostratigraphy of the Mosasauridae (Reptilia) from the Cretaceous of Antarctica". Geological Society, London, Special Publications. 258 (1): 101–108. Bibcode:2006GSLSP.258..101M. doi:10.1144/gsl.sp.2006.258.01.07.
- Martin S. Fernandez; Zulma Gasparini (2012). "Campanian and Maastrichtian mosasaurs from Antarctic Peninsula and Patagonia, Argentina". Bulletin de la Société Géologique de France. 183 (2): 93–102. doi:10.2113/gssgfbull.183.2.93.
- G. L. Bell Jr.; J. E. Martin (1995). "Direct evidence of aggressive intraspecific competition in Mosasaurus conodon (Mosasauridae:Squamata)". Journal of Vertebrate Paleontology. 15 (suppl. to 3): 18A. doi:10.1080/02724634.1995.10011277.
- Carolyn Gramling (2016-10-26). "Ancient sea monster battle revealed in unusual fossil". Science. doi:10.1126/science.aal0310.
- Theagarten Lingham-Soliar (2004). "Palaeopathology and injury in the extinct mosasaurs (Lepidosauromorpha, Squamata) and implications for modern reptiles". Lethaia. 37 (3): 255–262. doi:10.1080/00241160410006519.
- Anne S. Schulp; Geert H.I.M. Walenkamp; Paul A.M. Hofman; Yvonne Stuip; Bruce M. Rothschild (2006). "Chronic bone infection in the jaw of Mosasaurus hoffmanni (Squamata)" (PDF). 6 (2006): 41–52. Cite journal requires
|journal=
(help) - John A. Robbins (2010). Investigating Holocene climate change on the northern Channel Islands and Cretaceous mosasaur ecology using stable isotopes (PhD). Southern Methodist University. ISBN 978-1-124-43286-1.
- Erle G. Kauffman (2004). "Mosasaur Predation on Upper Cretaceous Nautiloids and Ammonites from the United States Pacific Coast". PALAIOS. 19 (1): 96–100. Bibcode:2004Palai..19...96K. doi:10.1669/0883-1351(2004)019<0096:MPOUCN>2.0.CO;2.
- Daniel J. Field; Aaron LeBlanc; Adrienne Gau; Adam D. Behlke (2015). "Pelagic neonatal fossils support viviparity and precocial life history of Cretaceous mosasaurs". Palaeontology. 58 (3): 401–407. doi:10.1111/pala.12165.
- G. L. Bell Jr.; M. A. Sheldon; J. P. Lamb; J. E. Martin (1996). "The first direct evidence of live birth in Mosasauridae (Squamata): Exceptional preservation in Cretaceous Pierre Shale of South Dakota". Journal of Vertebrate Paleontology. 16 (suppl. to 3): 21A. doi:10.1080/02724634.1996.10011371.
- Alexandra Houssaye; Paul Tafforeau (2012). "What vertebral microanatomy reveals about the ecology of juvenile mosasaurs (Reptilia, Squamata)". Journal of Vertebrate Paleontology. 32 (5): 1042–1048. doi:10.1080/02724634.2012.680999. S2CID 84662320.
- James E. Martin (2002). "Juvenile marine reptiles from the Late Cretaceous of the Antarctic peninsula and their relationships to other such occurrences in central South Dakota and Belgium" (PDF). Proceedings of the South Dakota Academy of Science. 81 (2002): 53–57.
- B. M. Rothschild; L. D. Martin (2005). "Mosasaur ascending: the phytogeny of bends". Netherlands Journal of Geosciences. 84 (Special Issue 3): 341–344. doi:10.1017/S0016774600021120.
- Agnete Weinreich Carlsen (2017). "Frequency of decompression illness among recent and extinct mammals and "reptiles": a review". The Science of Nature. 104 (7–8): 56. Bibcode:2017SciNa.104...56C. doi:10.1007/s00114-017-1477-1. PMID 28656350. S2CID 23194069.
- Nathalie Bardet (2012). "Maastrichtian marine reptiles of the Mediterranean Tethys: a palaeobiogeographical approach". Bulletin de la Société Géologique de France. 183 (6): 573–596. doi:10.2113/gssgfbull.183.6.573.
- Elizabeth L. Nicholls and Anthony P. Russel (1990). "Paleobiogeography of the Cretaceous Western Interior Seaway of North America: the vertebrate evidence". Palaeogeography, Palaeoclimatology, Palaeoecology. 79 (1–2): 149–169. Bibcode:1990PPP....79..149N. doi:10.1016/0031-0182(90)90110-S.
- David B. Kemp; Stuart A. Robinson; J. Alistair Crame; Jane E. Francis; Jon Ineson; Rowan J. Whittle; Vanessa Bowman; Charlotte O'Brien (2014). "A cool temperate climate on the Antarctic Peninsula through the latest Cretaceous to early Paleogene". Geology. 42 (7): 583–586. Bibcode:2014Geo....42..583K. doi:10.1130/g35512.1.
- Jose-Carmelo Corral; Ana Berreteaga; Henri Cappetta (2016). "Upper Maastrichtian shallow marine environments and neoselachian assemblages in North Iberian palaeomargin (Castilian Ramp, Spain)". Cretaceous Research. 57: 639–661. doi:10.1016/j.cretres.2015.08.001.
- Matt Friedman (2012). "Ray-finned fishes (Osteichthyes, Actinopterygii) from the type Maastrichtian, the Netherlands and Belgium". Scripta Geologica (8): 113–142.
- Steven M. Stanley (1999). Earth System History. New York: W.H. Freeman and Company. pp. 487–489. ISBN 978-0-7167-2882-5.
- S. He; T. K. Kyser; W. G. E. Caldwell (2005). "Paleoenvironment of the Western Interior Seaway inferred from δ18O and δ13C values of molluscs from the Cretaceous Bearpaw marine cyclothem". Palaeogeography, Palaeoclimatology, Palaeoecology. 217 (2005): 67–85. Bibcode:2005PPP...217...67H. doi:10.1016/j.palaeo.2004.11.016.
- Cynthia G. Fisher; Michael A. Arthur (2002). "Water mass characteristics in the Cenomanian US Western Interior seaway as indicated by stable isotopes of calcareous organisms". Palaeogeography, Palaeoclimatology, Palaeoecology. 188 (2002): 89–213. Bibcode:2002PPP...188..189F. doi:10.1016/S0031-0182(02)00552-7.
- Caitlin R. Kiernan (2002). "Stratigraphic distribution and habitat segregation of mosasaurs in the Upper Cretaceous of western and central Alabama, with a historical review of Alabama mosasaur discoveries". Journal of Vertebrate Paleontology. 22 (1): 91–103. doi:10.1671/0272-4634(2002)022[0091:sdahso]2.0.co;2.
- Andrew D. Gentry; James F. Parham; Dana J. Ehret; Jun A. Ebersole (2018). "A new species of Peritresius Leidy, 1856 (Testudines: Pan-Cheloniidae) from the Late Cretaceous (Campanian) of Alabama, USA, and the occurrence of the genus within the Mississippi Embayment of North America". PLOS ONE. 13 (4): e0195651. Bibcode:2018PLoSO..1395651G. doi:10.1371/journal.pone.0195651. PMC 5906092. PMID 29668704.
- Jun Ebersole; Takehito Ikejiri; Harry Lyon Blewitt; Sandy Ebersole (2013). "An Overview of Late Cretaceous Vertebrates from Alabama". Bulliten of the Alabama Museum of Natural History. 31 (1): 46–70.
- Paulina Jiménez-Huidobro; Michael W. Caldwell (2019). "A New Hypothesis of the Phylogenetic Relationships of the Tylosaurinae (Squamata: Mosasauroidea)". Frontiers in Earth Science. 7 (47): 47. Bibcode:2019FrEaS...7...47J. doi:10.3389/feart.2019.00047.
- Todd Cook, Eric Brown, Patricia E. Ralrick, and Takuya Konish. (2017). "A late Campanian euselachian assemblage from the Bearpaw Formation of Alberta, Canada: some notable range extensions". Canadian Journal of Earth Sciences. 54 (9): 973–980. Bibcode:2017CaJES..54..973C. doi:10.1139/cjes-2016-0233. hdl:1807/77762.CS1 maint: multiple names: authors list (link)
- Tim T. Tokaryk; C. R. Harington (1992). "Baptornis sp. (Aves: Hesperornithiformes) from the Judith River Formation (Campanian) of Saskatchewan, Canada". Journal of Paleontology. 66 (6): 1010–1012. doi:10.1017/S002233600002093X.
- Tamaki Sato (2003). "Terminonatator ponteixensis, a new elasmosaur (Reptilia; Sauropterygia) from the Upper Cretaceous of Saskatchewan". Journal of Vertebrate Paleontology. 23 (1): 89–103. doi:10.1671/0272-4634(2003)23[89:tpanes]2.0.co;2.
- Tamaki Sato (2005). "A new polycotylid plesiosaur (Reptilia: Sauropterygia) from the Upper Cretaceous Bearpaw Formation in Saskatchewan, Canada". Journal of Paleontology. 79 (5): 969–980. doi:10.1666/0022-3360(2005)079[0969:anpprs]2.0.co;2.
- Hoganson, J. W.; Woodward, B. (2004). "Skeleton of the Rare Giant Sea Turtle, Archelon, Recovered from the Cretaceous DeGrey Member of the Pierre Shale near Cooperstown, Griggs County, North Dakota" (PDF). North Dakota Geological Society Newsletter. 32 (1): 1–4.
- David J. Cicimurri; Gorden L. Bell, Jr.; Philip W. Stoffer (1999). "Vertebrate Paleontology of the Pierre Shale and Fox Hills Formations (Late Campanian-Late Maastrichtian) of Badlands National Park, South Dakota" (PDF). National Park Service Paleontological Research. 4: 1–7.
- Thomas S. Tobin; Peter D. Ward; Eric J. Steig; Eduardo B. Olivero; Isaac A. Hilburn; Ross N. Mitchell; Matthew R. Diamond; Timothy D. Raub; Joseph L. Kirschvink (2012). "Extinction patterns, δ18 O trends, and magnetostratigraphy from a southern high-latitude Cretaceous–Paleogene section: Links with Deccan volcanism". Palaeogeography, Palaeoclimatology, Palaeoecology. 350-352 (2012): 180–188. Bibcode:2012PPP...350..180T. doi:10.1016/j.palaeo.2012.06.029.
- Rodrigo A. Oteroa; Sergio Soto-Acuña; David Rubilar-Rogers; Carolina S. Gutstein (2017). "Kaikaifilu hervei gen. et sp. nov., a new large mosasaur (Squamata, Mosasauridae) from the upper Maastrichtian of Antarctica". Cretaceous Research. 70: 209–225. doi:10.1016/j.cretres.2016.11.002.
- José P. O’Gorman; Karen M. Panzeri; Marta S. Fernández; Sergio Santillana; Juan J. Moly; Marcelo Reguero (2018). "A new elasmosaurid from the upper Maastrichtian López de Bertodano Formation: new data on weddellonectian diversity". Alcheringa: An Australasian Journal of Palaeontology. 42 (4): 575–586. doi:10.1080/03115518.2017.1339233. S2CID 134265841.
- Alberto L. Cione; Sergio Santillana; Soledad Gouiric-Cavalli; Carolina Acosta Hospitaleche; Javier N. Gelfo; Guillermo M. Lopez; Marcelo Reguero (2018). "Before and after the K/Pg extinction in West Antarctica: New marine fish records from Marambio (Seymour) Island". Cretaceous Research. 85: 250–265. doi:10.1016/j.cretres.2018.01.004.
- T.L. Harrell Jr.; A. Pérez-Huerta (2014). "Habitat preference of mosasaurs indicated by rare earth element (REE) content of fossils from the Upper Cretaceous marine deposits of Alabama, New Jersey, and South Dakota (USA)". Netherlands Journal of Geosciences. 94 (1): 145–154. doi:10.1017/njg.2014.29.
- Johan Lindgren (2005). "The first record of Hainosaurus (Reptilia: Mosasauridae) from Sweden". Journal of Paleontology. 79 (6): 1157–1165. doi:10.1111/j.1502-3931.1998.tb00520.x.
- Theagarten Lingham-Soliar (1998). "Unusual death of a Cretaceous giant". Lethaia. 31 (4): 308–310. doi:10.1111/j.1502-3931.1998.tb00520.x.
- John W. Jagt; Dirk Cornelissen; Eric W. Mulder; Anne S. Schulp; Jacques Severinjns; Louis Verding (2008). "The youngest in situ record to date of Mosasaurus hoffmannii (Squamata, Mosasauridae) from the Maastrichtian type area, The Netherlands". Proceedings of the Second Mosasaur Meeting: 73–80.
- William B. Gallagher; Carl E. Campbell; John W. M. Jagt; Eric W. A. Mulder (2005). "Mosasaur (Reptilia, Squamata) material from the Cretaceous-Tertiary boundary interval in Missouri". Journal of Vertebrate Paleontology. 25 (22): 473–475. doi:10.1671/0272-4634(2005)025[0473:mrsmft]2.0.co;2.
- "GEOL 104 Lecture 38: The Cretaceous-Tertiary Extinction III: Not With a Bang, But a Whimper". University of Maryland Department of Geology. 2006. Archived from the original on 2012-03-13.
External links
![]() |
Wikimedia Commons has media related to Mosasaurus. |