Last universal common ancestor
The last universal common ancestor or last universal cellular ancestor (LUCA), also called the last universal ancestor (LUA), or concestor, is the most recent population of organisms from which all organisms now living on Earth have a common descent, the most recent common ancestor of all current life on Earth.[1] (A related concept is that of progenote.[3]) LUCA is not thought to be the first life on Earth, but rather the only type of organism of its time to still have living descendants.
Part of a series on |
Evolutionary biology |
---|
![]() |
Key topics |
Fields and applications
|
Social implications
|
|
While there is no specific fossil evidence of LUCA, it can be studied by comparing the genomes of all modern organisms, its descendants. By these means, a 2016 study identified a set of 355 genes most likely to have been present in LUCA.[4][5][lower-alpha 1] The genes describe a complex life form with many co-adapted features, including transcription and translation mechanisms to convert information from DNA to RNA to proteins. The study concluded that the LUCA probably lived in the high-temperature water of deep sea vents near ocean-floor magma flows.
Studies from 2000–2018 have suggested an increasingly ancient time for LUCA. In 2000, estimations suggested LUCA existed 3.5 to 3.8 billion years ago in the Paleoarchean era,[7][8] a few hundred million years before the earliest fossil evidence of life, for which there are several candidates ranging in age from 3.48 to 4.28 billion years ago.[9][10][11][12][13][14][15] A 2018 study from the University of Bristol, applying a molecular clock model, places the LUCA shortly after 4.5 billion years ago, within the Hadean.[16][17]
Charles Darwin first proposed the theory of universal common descent through an evolutionary process in his book On the Origin of Species in 1859: "Therefore I should infer from analogy that probably all the organic beings which have ever lived on this earth have descended from some one primordial form, into which life was first breathed."[18] Later biologists have separated the problem of the origin of life from that of the LUCA.
Features
By analysis of the presumed LUCA's offspring groups, the LUCA appears to have been a small, single-celled organism. It likely had a ring-shaped coil of DNA floating freely within the cell. Morphologically, it would likely not have stood out within a mixed population of small modern-day bacteria. However, Carl Woese et al., who first proposed the currently-used three domain system based on an analysis of ribosomal RNA (rRNA) sequences of bacteria, archaea, and eukaryotes, stated that in its genetic machinery, the LUCA would have been a "... simpler, more rudimentary entity than the individual ancestors that spawned the three [domains] (and their descendants)".[19]
While the gross anatomy of LUCA can only be reconstructed with much uncertainty, its biochemical mechanisms can be described in some detail, based on the properties currently shared by all independently living organisms on Earth.[20][21][22][23]
Its genetic code was likely based on DNA,[24] so that it lived after the RNA world.[lower-alpha 2] If DNA was present, it was composed exclusively of the four modern-day nucleotides: deoxyadenosine, deoxycytidine, deoxythymidine, and deoxyguanosine. The DNA was kept double-stranded by a template-dependent enzyme, DNA polymerase, which was recently proposed to belong to the family D.[27] The integrity of the DNA benefited from a group of maintenance and repair enzymes including DNA topoisomerase.[28] If the genetic code was DNA-based, it was expressed via single-stranded RNA intermediates. The RNA was produced by a DNA-dependent RNA polymerase using nucleotides similar to those of DNA, with the exception that the DNA nucleotide thymidine was replaced by uridine in RNA.[20][21][22][23] It had multiple DNA-binding proteins, such as histone-fold proteins.[29]
The genetic code was expressed into proteins. These were assembled from free amino acids by translation of a messenger RNA via a mechanism of ribosomes, transfer RNAs, and a group of related proteins. The ribosomes were composed of two subunits, a big 50S and a small 30S. Each ribosomal subunit was composed of a core of ribosomal RNA surrounded by ribosomal proteins. Both types of RNA molecules (ribosomal and transfer RNAs) played an important role in the catalytic activity of the ribosomes. Only 20 amino acids were used, only in L-isomers, to the exclusion of countless other amino acids. ATP served as an energy intermediate. Several hundred protein enzymes catalyzed chemical reactions to extract energy from fats, sugars, and amino acids, and to synthesize fats, sugars, amino acids, and nucleic acid bases through various chemical pathways.[20][21][22][23]
The cell contained a water-based cytoplasm effectively enclosed by a lipid bilayer membrane. The cell tended to exclude sodium and concentrate potassium by means of specific ion transporters (or ion pumps). The cell multiplied by duplicating all its contents followed by cellular division.[20][21][22][23] The cell used chemiosmosis to produce energy. It also reduced CO2 and oxidized H2 (methanogenesis or acetogenesis) via acetyl-thioesters.[30][31]
The LUCA probably lived in the high-temperature conditions found in deep sea vents caused by ocean water interacting with magma beneath the ocean floor.[32][4]
Hypotheses
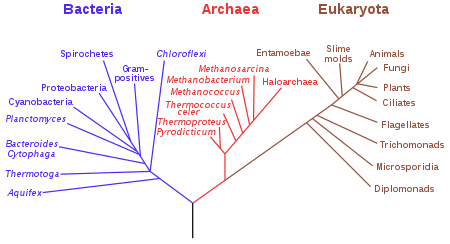
In 1859, Charles Darwin published On the Origin of Species, in which he twice stated the hypothesis that there was only one progenitor for all life forms. In the summation he states:
- "Therefore I should infer from analogy that probably all the organic beings which have ever lived on this earth have descended from some one primordial form, into which life was first breathed."[34]
The very last sentence begins with a restatement of the hypothesis:
- "There is grandeur in this view of life, with its several powers, having been originally breathed into a few forms or into one ..."[34]
When the LUCA was hypothesized, cladograms based on genetic distance between living cells indicated that Archaea split early from the rest of living things. This was inferred from the fact that the archaeans known at that time were highly resistant to environmental extremes such as high salinity, temperature or acidity, leading some scientists to suggest that the LUCA evolved in areas like the deep ocean vents, where such extremes prevail today. Archaea, however, were later discovered in less hostile environments, and are now believed to be more closely related to the Eukaryota than to the Bacteria, although many details are still unknown.[35][36]
.svg.png)
In 2010, based on "the vast array of molecular sequences now available from all domains of life,"[38] a formal test of universal common ancestry was published.[1] The formal test favored the existence of a universal common ancestor over a wide class of alternative hypotheses that included horizontal gene transfer. Basic biochemical principles make it overwhelmingly likely that all organisms do have a single common ancestor. It is extremely unlikely that organisms descended from separate incidents of cell-formation would be able to complete a horizontal gene transfer without garbling each other's genes, converting them into noncoding segments. Further, many more amino acids are chemically possible than the 22 found in protein molecules. These lines of chemical evidence, incorporated into the formal statistical test point to a single cell having been the LUCA. While the test overwhelmingly favored the existence of a single LUCA, this does not imply that the LUCA was ever alone: Instead, it was one of many early microbes[1] but the only one whose descendants survived beyond the Paleoarchean Era.[39]
With the later gene pool of the LUCA's descendants, with their common framework of the AT/GC rule and the standard twenty amino acids, horizontal gene transfer would have been feasible and could have been very common.
In an earlier hypothesis, Carl Woese (1988) had proposed that:
- no individual organism can be considered a LUCA, and
- the genetic heritage of all modern organisms derived through horizontal gene transfer among an ancient community of organisms.[40]
While the results described by Theobald (2010) and Saey (2010) demonstrate the existence of a single LUCA, Woese's argument can still be applied to Ur-organisms (initial products of abiogenesis) before the LUCA. At the beginnings of life, ancestry was not as linear as it is today because the genetic code had not evolved.[41] Before high fidelity replication, organisms could not be easily mapped on a phylogenetic tree. However, the LUCA lived after the origin of the genetic code and at least some rudimentary early form of molecular proofreading.
Location of the root
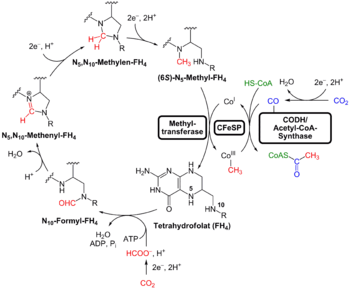
The most commonly accepted tree of life, based on several molecular studies, has its root between a monophyletic domain Bacteria and a clade formed by Archaea and Eukaryota.[42][43][44][45][46][47] However, a very small minority of studies place the root in the domain Bacteria, either in the phylum Firmicutes[48] or state that the phylum Chloroflexi is basal to a clade with Archaea and Eukaryotes and the rest of Bacteria (as proposed by Thomas Cavalier-Smith).[49]
Research by William F. Martin (2016) genetically analyzed 6.1 million protein-coding genes and 286,514 protein clusters from sequenced prokaryotic genomes of various phylogenetic trees, and identified 355 protein clusters that were probably common to the LUCA. The results "depict LUCA as anaerobic, CO2-fixing, H2-dependent with a Wood–Ljungdahl pathway (the reductive acetyl-coenzyme A pathway), N2-fixing and thermophilic. LUCA's biochemistry was replete with FeS clusters and radical reaction mechanisms." The cofactors also reveal "dependence upon transition metals, flavins, S-adenosyl methionine, coenzyme A, ferredoxin, molybdopterin, corrins and selenium. Its genetic code required nucleoside modifications and S-adenosylmethionine-dependent methylations."[4][50][51] The results are "quite specific":[5] they show that methanogenic clostridia was a basal clade in the 355 lineages examined, and that the LUCA may therefore have inhabited an anaerobic hydrothermal vent setting in a geochemically active environment rich in H2, CO2, and iron.[4]
These findings could mean that life on Earth originated in such hydrothermal vents, but it is also possible that life was restricted to such locations at some later time, perhaps by the Late Heavy Bombardment.[5] The identification of these genes as being present in LUCA has also been criticized, as they may simply represent later genes which migrated via horizontal gene transfers between archaea and bacteria.[6]
Viruses
Based on the extant distribution of viruses across the two primary domains of life, bacteria and archaea, it has been suggested that LUCA was associated with a remarkably complex virome that already included the main groups of extant viruses of bacteria and archaea and that extensive virus evolution has antedated the LUCA.[52] This ancestral virome was likely dominated by dsDNA viruses from the realms Duplodnaviria and Varidnaviria. In addition, two groups of ssDNA viruses (realm Monodnaviria), namely Microviridae and Tubulavirales, can be traced to the last bacterial common ancestor (LBCA), whereas spindle-shaped viruses, most likely infected the last archaeal common ancestor (LACA). The possibility that these virus groups were present in the LUCA virome but were subsequently lost in one of the two primary domains cannot be dismissed. By contrast, RNA viruses do not appear to have been a prominent part of the LUCA virome, even though straightforward thinking might have envisaged the LUCA virome as a domain of RNA viruses descending from the primordial RNA world. Instead, by the time the LUCA lived, RNA viruses had probably already been largely supplanted by the more efficient DNA virosphere.[52]
See also
- Abiogenesis
- Bacterial phyla
- Common descent
- Origin of the first cell
- Protocell
- Panspermia
- Timeline of evolution
Footnotes
- However, some of those genes could have developed later, then spread universally by horizontal gene transfer ("gene sharing") between archaea and bacteria.[6]
- However, other studies propose that LUCA may have been defined wholly through RNA,[25] consisted of a RNA-DNA hybrid genome, or possessed a retrovirus-like genetic cycle with DNA serving as a stable genetic repository.[26]
References
- Theobald, D.L. (May 2010). "A formal test of the theory of universal common ancestry". Nature. 465 (7295): 219–222. Bibcode:2010Natur.465..219T. doi:10.1038/nature09014. PMID 20463738. S2CID 4422345.
- Gogarten, Johann Peter; Deamer, David (25 November 2016). "Is LUCA a thermophilic progenote?". Nature Microbiology. 1 (12): 16229. doi:10.1038/nmicrobiol.2016.229. PMID 27886195. S2CID 205428194.
- There is a common misconception that definitions of LUCA and progenote are the same; however, progenote is defined as an organism "still in the process of evolving the relationship between genotype and phenotype". It is only a hypothesis that LUCA is was a progenote.[2]
- Weiss, M.C.; Sousa, F. L.; Mrnjavac, N.; Neukirchen, S.; Roettger, M.; Nelson-Sathi, S.; Martin, W.F. (2016). "The physiology and habitat of the last universal common ancestor". Nat Microbiol. 1 (9): 16116. doi:10.1038/nmicrobiol.2016.116. PMID 27562259. S2CID 2997255.
- Wade, Nicholas (25 July 2016). "Meet Luca, the ancestor of all living things". The New York Times. Archived from the original on 28 July 2016. Retrieved 8 October 2018.
- Gogarten, Johann Peter; Deamer, David (2016). "Is LUCA a thermophilic progenote?". Nature Microbiology. 1 (12): 16229. doi:10.1038/nmicrobiol.2016.229. PMID 27886195. S2CID 205428194.
- Doolittle, W.F. (February 2000). "Uprooting the tree of life". Scientific American. 282 (2): 90–95. Bibcode:2000SciAm.282b..90D. doi:10.1038/scientificamerican0200-90. PMID 10710791.
- Glansdorff, N.; Xu, Y.; Labedan, B. (2008). "The last universal common ancestor: emergence, constitution and genetic legacy of an elusive forerunner". Biology Direct. 3: 29. doi:10.1186/1745-6150-3-29. PMC 2478661. PMID 18613974.
- Borenstein, Seth (13 November 2013). "Oldest fossil found: Meet your microbial mom". Associated Press. Archived from the original on 29 June 2015. Retrieved 15 November 2013.
- Noffke, N.; Christian, D.; Wacey, D.; Hazen, R.M. (December 2013). "Microbially induced sedimentary structures recording an ancient ecosystem in the ca. 3.48 billion-year-old Dresser Formation, Pilbara, Western Australia". Astrobiology. 13 (12): 1103–1124. Bibcode:2013AsBio..13.1103N. doi:10.1089/ast.2013.1030. PMC 3870916. PMID 24205812.
- Ohtomo, Yoko; Kakegawa, Takeshi; Ishida, Akizumi; Nagase, Toshiro; Rosing, Minik T. (2013). "Evidence for biogenic graphite in early Archaean Isua metasedimentary rocks". Nature Geoscience. 7 (1): 25–28. Bibcode:2014NatGe...7...25O. doi:10.1038/ngeo2025.
- Hassenkam, T.; Andersson, M.P.; Dalby, K.N.; Mackenzie, D.M.A.; Rosing, M.T. (2017). "Elements of Eoarchean life trapped in mineral inclusions". Nature. 548 (7665): 78–81. Bibcode:2017Natur.548...78H. doi:10.1038/nature23261. PMID 28738409. S2CID 205257931.
- Borenstein, Seth (19 October 2015). "Hints of life on what was thought to be desolate early Earth". AP News. Associated Press. Archived from the original on 14 December 2018. Retrieved 9 October 2018.
- Bell, Elizabeth A.; Boehnke, Patrick; Harrison, T. Mark; Mao, Wendy L. (24 November 2015). "Potentially biogenic carbon preserved in a 4.1 billion-year-old zircon". Proceedings of the National Academy of Sciences of the United States of America. 112 (47): 14518–14521. Bibcode:2015PNAS..11214518B. doi:10.1073/pnas.1517557112. ISSN 1091-6490. PMC 4664351. PMID 26483481.
- Dodd, Matthew S.; Papineau, Dominic; Grenne, Tor; slack, John F.; Rittner, Martin; Pirajno, Franco; O'Neil, Jonathan; Little, Crispin T.S. (2 March 2017). "Evidence for early life in Earth's oldest hydrothermal vent precipitates" (PDF). Nature. 543 (7643): 60–64. Bibcode:2017Natur.543...60D. doi:10.1038/nature21377. PMID 28252057. S2CID 2420384. Archived (PDF) from the original on 23 July 2018. Retrieved 25 June 2019.
- Betts, Holly C.; Puttick, Mark N.; Clark, James W.; Williams, Tom A.; Donoghue, Philip C.J.; Pisani, Davide (20 August 2018). "Integrated genomic and fossil evidence illuminates life's early evolution and eukaryote origin" (PDF). Nature Ecology & Evolution. 2 (10): 1556–1562. doi:10.1038/s41559-018-0644-x. PMC 6152910. PMID 30127539. Archived from the original (PDF) on 30 August 2019. Retrieved 11 June 2019.
- "A timescale for the origin and evolution of all of life on Earth". University of Bristol. 20 August 2018. Archived from the original on 18 March 2019. Retrieved 11 June 2019 – via phys.org.
- Darwin, Charles (1859). The Origin of Species by Means of Natural Selection. John Murray. p. 490.
- Woese, C. R.; Kandler, O.; Wheelis, M. L. (1990). "Towards a natural system of organisms: proposal for the domains Archaea, Bacteria, and Eucarya". Proceedings of the National Academy of Sciences. 87 (12): 4576–79. Bibcode:1990PNAS...87.4576W. doi:10.1073/pnas.87.12.4576. ISSN 0027-8424. PMC 54159. PMID 2112744.
- Wächtershäuser, Günter (1998). "Towards a Reconstruction of Ancestral Genomes by Gene Cluster Alignment". Systematic and Applied Microbiology. 21 (4): 473–74, IN1, 475–77. doi:10.1016/S0723-2020(98)80058-1.
- Gregory, Michael. "What is Life?". Clinton College. Archived from the original on 2007-12-13.
- Pace, N.R. (January 2001). "The universal nature of biochemistry". Proceedings of the National Academy of Sciences of the United States of America. 98 (3): 805–808. Bibcode:2001PNAS...98..805P. doi:10.1073/pnas.98.3.805. PMC 33372. PMID 11158550.
- Wächtershäuser, G. (January 2003). "From pre-cells to Eukarya – a tale of two lipids". Molecular Microbiology. 47 (1): 13–22. doi:10.1046/j.1365-2958.2003.03267.x. PMID 12492850.
- Garwood, Russell J. (2012). "Patterns In Palaeontology: The first 3 billion years of evolution". Palaeontology Online. 2 (11): 1–14. Archived from the original on June 26, 2015. Retrieved June 25, 2015.
- Marshall, Michael. "Life began with a planetary mega-organism". New Scientist. Archived from the original on 25 July 2016. Retrieved 31 July 2016.
- Koonin, Eugene V.; Martin, William (1 December 2005). "On the origin of genomes and cells within inorganic compartments". Trends in Genetics. 21 (12): 647–654. doi:10.1016/j.tig.2005.09.006. ISSN 0168-9525. PMC 7172762. PMID 16223546.
- Koonin, EV; Krupovic, M; Ishino, S; Ishino, Y (2020). "The replication machinery of LUCA: common origin of DNA replication and transcription". BMC Biology. 18 (1): 61. doi:10.1186/s12915-020-00800-9. PMC 7281927. PMID 32517760.
- Ahmad, Muzammil; Xu, Dongyi; Wang, Weidong (23 May 2017). "Type IA topoisomerases can be "magicians" for both DNA and RNA in all domains of life". RNA Biology. 14 (7): 854–864. doi:10.1080/15476286.2017.1330741. ISSN 1547-6286. PMC 5546716. PMID 28534707.
- Lupas, Andrei N.; Alva, Vikram (2018). "Histones predate the split between bacteria and archaea". Bioinformatics. 35 (14): 2349–2353. doi:10.1093/bioinformatics/bty1000. PMID 30520969.
- Martin, W.; Russell, M. J. (October 2007). "On the origin of biochemistry at an alkaline hydrothermal vent". Philosophical Transactions of the Royal Society of London. Series B, Biological Sciences. 362 (1486): 1887–925. doi:10.1098/rstb.2006.1881. PMC 2442388. PMID 17255002.
- Lane, N.; Allen, J. F.; Martin, W. (April 2010). "How did LUCA make a living? Chemiosmosis in the origin of life". BioEssays. 32 (4): 271–80. doi:10.1002/bies.200900131. PMID 20108228.
- Wade, Nicholas (25 July 2016). "Meet Luca, the Ancestor of All Living Things". The New York Times. ISSN 0362-4331. Archived from the original on 28 July 2016. Retrieved 2 March 2017.
- Woese, C.R.; Kandler, O.; Wheelis, M.L. (June 1990). "Towards a natural system of organisms: proposal for the domains Archaea, Bacteria, and Eucarya". Proceedings of the National Academy of Sciences of the United States of America. 87 (12): 4576–79. Bibcode:1990PNAS...87.4576W. doi:10.1073/pnas.87.12.4576. PMC 54159. PMID 2112744.
- Darwin, Charles (1859). On the Origin of Species. London, UK: John Murray. pp. 484, 490.
- Xie, Q.; Wang, Y.; Lin, J.; Qin, Y.; Wang, Y.; Bu, W. (2012). "Potential key bases of ribosomal RNA to kingdom-specific spectra of antibiotic susceptibility and the possible archaeal origin of eukaryotes". PLOS ONE. 7 (1): e29468. Bibcode:2012PLoSO...729468X. doi:10.1371/journal.pone.0029468. PMC 3256160. PMID 22247777.
- Yutin, N.; Makarova K.S.; Mekhedov S.L.; Wolf, Y.I.; Koonin, E.V. (August 2008). "The deep archaeal roots of eukaryotes". Molecular Biology and Evolution. 25 (8): 1619–30. doi:10.1093/molbev/msn108. PMC 2464739. PMID 18463089.
- Smets, Barth F.; Barkay, Tamar (September 2005). "Horizontal gene transfer: perspectives at a crossroads of scientific disciplines". Nature Reviews Microbiology. 3 (9): 675–678. doi:10.1038/nrmicro1253. PMID 16145755. S2CID 2265315.
- Steel, M.; Penny, D. (May 2010). "Origins of life: Common ancestry put to the test". Nature. 465 (7295): 168–69. Bibcode:2010Natur.465..168S. doi:10.1038/465168a. PMID 20463725. S2CID 205055573.
- Egel, Richard (March 2012). "Primal Eukaryogenesis: On the Communal Nature of Precellular States, Ancestral to Modern Life". Life. 2 (1): 170–212. doi:10.3390/life2010170. PMC 4187143. PMID 25382122.
- Woese, C. (June 1998). "The universal ancestor". Proceedings of the National Academy of Sciences of the United States of America. 95 (12): 6854–6859. Bibcode:1998PNAS...95.6854W. doi:10.1073/pnas.95.12.6854. PMC 22660. PMID 9618502.
- Maynard Smith, John; Szathmáry, Eörs (1995). The Major Transitions in Evolution. Oxford, England: Oxford University Press. ISBN 978-0-19-850294-4. Archived from the original on 13 March 2017. Retrieved 27 January 2016.
- Brown, J.R.; Doolittle, W.F. (1995). "Root of the Universal Tree of Life Based on Ancient Aminoacyl-tRNA Synthetase Gene Duplications". Proc Natl Acad Sci USA. 92 (7): 2441–2445. Bibcode:1995PNAS...92.2441B. doi:10.1073/pnas.92.7.2441. PMC 42233. PMID 7708661.
- Gogarten, J. P.; Kibak, H.; Dittrich, P.; Taiz, L.; Bowman, E.J.; Bowman, B.J.; Manolson, M. F.; et al. (1989). "Evolution of the Vacuolar H+-ATPase: Implications for the Origin of Eukaryotes". Proc Natl Acad Sci USA. 86 (17): 6661–6665. Bibcode:1989PNAS...86.6661G. doi:10.1073/pnas.86.17.6661. PMC 297905. PMID 2528146.
- Gogarten, J.P.; Taiz, L. (1992). "Evolution of Proton Pumping ATPases: Rooting the Tree of Life". Photosynthesis Research. 33 (2): 137–146. doi:10.1007/BF00039176. PMID 24408574. S2CID 20013957.
- Gribaldo, S.; Cammarano, P. (1998). "The Root of the Universal Tree of Life Inferred from Anciently Duplicated Genes Encoding Components of the Protein-Targeting Machinery". Journal of Molecular Evolution. 47 (5): 508–16. Bibcode:1998JMolE..47..508G. doi:10.1007/pl00006407. PMID 9797401.
- Iwabe, Naoyuki; Kuma, Kei-Ichi; Hasegawa, Masami; Osawa, Syozo; Miyata Source, Takashi; Hasegawat, Masami; Osawat, Syozo; Miyata, Takashi (1989). "Evolutionary Relationship of Archaebacteria, Eubacteria, and Eukaryotes Inferred from Phylogenetic Trees of Duplicated Genes". Proc Natl Acad Sci USA. 86 (23): 9355–9359. Bibcode:1989PNAS...86.9355I. doi:10.1073/pnas.86.23.9355. PMC 298494. PMID 2531898.
- Boone, David R.; Castenholz, Richard W.; Garrity, George M., eds. (2001). The Archaea and the Deeply Branching and Phototrophic Bacteria. Bergey's Manual of Systematic Bacteriology. Springer. doi:10.1007/978-0-387-21609-6. ISBN 978-0-387-21609-6. S2CID 41426624. Archived from the original on 2014-12-25. Retrieved 2017-09-02.
- Valas, R.E.; Bourne, P.E. (2011). "The origin of a derived superkingdom: how a gram-positive bacterium crossed the desert to become an archaeon". Biology Direct. 6: 16. doi:10.1186/1745-6150-6-16. PMC 3056875. PMID 21356104.
- Cavalier-Smith, T. (2006). "Rooting the tree of life by transition analyses". Biology Direct. 1: 19. doi:10.1186/1745-6150-1-19. PMC 1586193. PMID 16834776.
- Lane, Nick (2015). The Vital Question: Energy, evolution, and the origins of complex life. W.W. Norton. ISBN 978-0-393-08881-6. Archived from the original on 20 August 2017 – via Google Books.
- Sutherland, Joseph F. "On the origin of the Bacteria and the Archaea". Archived from the original on 2017-09-10. Retrieved 16 August 2014.
- Krupovic, M; Dolja, VV; Koonin, EV (2020). "The LUCA and its complex virome". Nature Reviews Microbiology. doi:10.1038/s41579-020-0408-x. PMID 32665595.
External links
Media related to Last universal ancestor at Wikimedia Commons