Sustainable energy
Sustainable energy is the practice of using energy in a way that "meets the needs of the present without compromising the ability of future generations to meet their own needs."[1][2]
Part of a series about |
Sustainable energy |
---|
![]() |
Overview |
Energy conservation |
Renewable energy |
Sustainable transport |
|
Meeting the world's needs for electricity, heating, cooling, and power for transport in a sustainable way is widely considered to be one of the greatest challenges facing humanity in the 21st century. Worldwide, nearly a billion people lack access to electricity, and around 3 billion people rely on smoky fuels such as wood, charcoal or animal dung in order to cook. These and fossil fuels are a major contributor to air pollution, which causes an estimated 7 million deaths per year. Production and consumption of energy emits over 70% of human-caused greenhouse gas emissions.
Proposed pathways for limiting global warming to 1.5 °C describe rapid implementation of low-emission methods of producing electricity and a shift towards more use of electricity in sectors such as transport. The pathways also include measures to reduce energy consumption; and use of carbon-neutral fuels, such as hydrogen produced by renewable electricity or with carbon capture and storage.[3] Achieving these goals will require government policies including carbon pricing, energy-specific policies, and phase-out of fossil fuel subsidies.
When referring to methods of producing energy, the term "sustainable energy" is often used interchangeably with the term "renewable energy". In general, renewable energy sources such as solar, wind, and hydroelectric energy are widely considered to be sustainable. However, particular renewable energy projects, such as the clearing of forests for the production of biofuels, can lead to similar or even worse environmental damage when compared to using fossil fuel energy. Nuclear power is a zero emission source and while its sustainability is debated,[4][5] the European Union has chosen it to be the part of a low-carbon energy backbone by 2050.[6]
Moderate amounts of wind and solar energy, which are intermittent energy sources, can be integrated into the electrical grid without additional infrastructure such as grid energy storage. These sources generated 7.5% of worldwide electricity in 2018,[7] a share that has grown rapidly. As of 2019, costs of wind, solar, and batteries are projected to continue falling.
Definitions
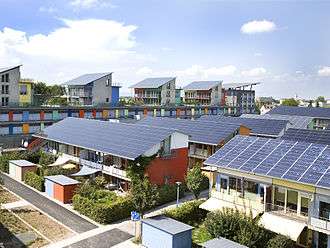
The concept of sustainable development was described by the World Commission on Environment and Development in its 1987 book Our Common Future.[1] Its definition of "sustainability", now used widely, was, "Sustainable development should meet the needs of the present without compromising the ability of future generations to meet their own needs."[1]
In its book, the Commission described four key elements of sustainability with respect to energy: the ability to increase the supply of energy to meet growing human needs, energy efficiency and conservation, public health and safety, and "protection of the biosphere and prevention of more localized forms of pollution."[8] Various definitions of sustainable energy have been offered since then which are also based on the three pillars of sustainable development, namely environment, economy, and society.
- Environmental criteria include greenhouse gas emissions, impact on biodiversity, and the production of hazardous waste and toxic emissions.
- Economic criteria include the cost of energy, whether energy is delivered to users with high reliability, and effects on jobs associated with energy production.
- Socio-cultural criteria include the prevention of wars over the energy supply (energy security) and long-term availability of energy.
The organizing principle for sustainability is sustainable development, which includes the four interconnected domains: ecology, economics, politics and culture.[9]
Current status
Providing sustainable energy is widely viewed as one of the greatest challenges facing humanity in the 21st century, both in terms of meeting the needs of the present and in terms of effects on future generations.[10][11] Bill Gates said in 2011:
If you gave me the choice between picking the next 10 presidents or ensuring that energy is environmentally friendly and a quarter as costly, I'd pick the energy thing.[12]
Worldwide, 940 million (13% of the world) people do not have access to electricity, and 3 billion people rely on dirty fuels for cooking[13]. Air pollution, caused largely by the burning of fuel, kills an estimated 7 million people each year.[14] The United Nations Sustainable Development Goals call for "access to affordable, reliable, sustainable and modern energy for all" by 2030.[15]
Energy production and consumption are major contributors to climate change, being responsible for 72% of annual human-caused greenhouse gas emissions as of 2014. Generation of electricity and heat contributes 31% of human-caused greenhouse gas emissions, use of energy in transportation contributes 15%, and use of energy in manufacturing and construction contributes 12%. An additional 5% is released through processes associated with fossil fuel production, and 8% through various other forms of fuel combustion.[16][17] As of 2015, 80% of the world's primary energy is produced from fossil fuels.[18]
In developing countries, over 2.5 billion people rely on traditional cookstoves[19] and open fires to burn biomass or coal for heating and cooking. This practice causes harmful local air pollution and increases the danger from fires, resulting in an estimated 4.3 million deaths annually.[20] Additionally, serious local environmental damage, including desertification, can be caused by excessive harvesting of wood and other combustible material.[21] Promoting usage of cleaner fuels and more efficient technologies for cooking is, therefore, one of the top priorities of the United Nations Sustainable Energy for All initiative. As of 2019, efforts to design clean cookstoves that are inexpensive, powered by sustainable energy sources, and acceptable to users have been mostly disappointing.[20]
Proposed pathways for climate change mitigation
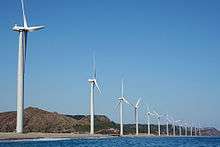
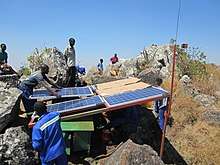
Cost–benefit analysis work has been done by a disparate array of specialists and agencies to determine the best path to decarbonizing the energy supply of the world.[22][23] The IPCC's 2018 Special Report on Global Warming of 1.5 °C says that for limiting warming to 1.5 °C and avoiding the worst effects of climate change, "global net human-caused emissions of CO
2 would need to fall by about 45% from 2010 levels by 2030, reaching net zero around 2050." As part of this report, the IPCC's working group on climate change mitigation reviewed a variety of previously-published papers that describe pathways (i.e. scenarios and portfolios of mitigation options) to stabilize the climate system through changes in energy, land use, agriculture, and other areas.
The pathways that are consistent with limiting warning to approximately 1.5 °C describe a rapid transition towards producing electricity through lower-emission methods, and increasing use of electricity instead of other fuels in sectors such as transportation.[24] These pathways have the following characteristics (unless otherwise stated, the following values are the median across all pathways):
- Renewable energy: The proportion of primary energy supplied by renewables increases from 15% in 2020 to 60% in 2050.[25] The proportion of primary energy supplied by biomass increases from 10% to 27%,[26] with effective controls on whether land use is changed in the growing of biomass.[27] The proportion from wind and solar increases from 1.8% to 21%.[26]
- Nuclear energy: The proportion of primary energy supplied by nuclear power increases from 2.1% in 2020 to 4% in 2050. Most pathways describe an increase in use of nuclear power, but some describe a decrease. The reason for the wide range of possibilities is that deployment of nuclear energy "can be constrained by societal preferences."[28]
- Coal and oil: Between 2020 and 2050, the proportion of primary energy from coal declines from 26% to 5%, and the proportion from oil declines from 35% to 13%.[26]
- Natural gas: In most pathways, the proportion of primary energy supplied by natural gas decreases, but in some pathways, it increases. Using the median values across all pathways, the proportion of primary energy from natural gas declines from 23% in 2020 to 13% in 2050. [26]
- Carbon capture and storage: Pathways describe more use of carbon capture and storage for bioenergy and fossil fuel energy.[28]
- Electrification: In 2020, around 20% of final energy use is provided by electricity. By 2050, this proportion more than doubles in most pathways.[29]
- Energy conservation: Pathways describe methods to increase energy efficiency and reduce energy demand in all sectors (industry, buildings, and transport). With these measures, pathways show energy usage to remain around the same between 2010 and 2030, and increase slightly by 2050.[30]
Renewable energy sources
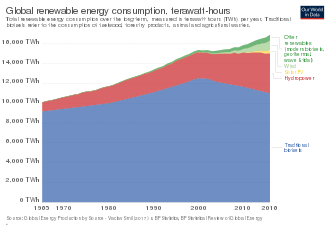
When referring to sources of energy, the terms "sustainable energy" and "renewable energy" are often used interchangeably, however particular renewable energy projects sometimes raise significant sustainability concerns. Renewable energy technologies are essential contributors to sustainable energy as they generally contribute to world energy security, and reduce dependence on fossil fuel resources thus mitigating greenhouse gas emissions.[31]
Solar power
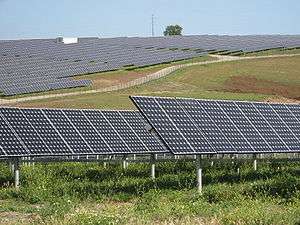
In 2018, solar power provided around 3% of global electricity.[7] Solar electricity production uses photovoltaic (PV) cells to convert light into electrical current. Photovoltaic modules can be integrated into buildings or used in photovoltaic power stations connected to the electrical grid. They are especially useful for providing electricity to remote areas. Although generally warranted for 25 years it is claimed that an average solar panel will last 40 years[32] and almost all of it can be recycled.[33]
Currently, photovoltaic (PV) panels only have the ability to convert around 24% of the sunlight that hits them into electricity.[34] At this rate, solar energy still holds many challenges for widespread implementation, but steady progress has been made in reducing manufacturing cost and increasing photovoltaic efficiency. In 2008, researchers at Massachusetts Institute of Technology (MIT) developed a method to store solar energy by using it to produce hydrogen fuel from water.[35] Such research is targeted at addressing the obstacle that solar development faces of storing energy for use during nighttime hours when the sun is not shining.
Large national and regional research projects on artificial photosynthesis are designing nanotechnology-based systems that use solar energy to split water into hydrogen fuel[36] and a proposal has been made for a Global Artificial Photosynthesis project.[37]
Research is ongoing in space-based solar power, a concept in which solar panels are launched into outer space and the energy they capture is transmitted back to Earth as microwaves. A test facility for the technology is being built in China.[38]
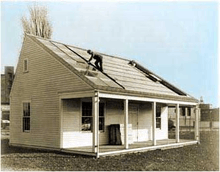
Solar heating
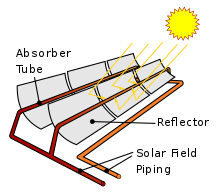
Solar heating systems generally consist of solar thermal collectors, a fluid system to move the heat from the collector to its point of usage, and a reservoir or tank for heat storage and subsequent use. The systems may be used to heat domestic hot water, swimming pool water, or for space heating.[39] The heat can also be used for industrial applications or as an energy input for other uses such as cooling equipment.[40] In many climates, a solar heating system can provide a very high percentage (20 to 80%) of domestic hot water energy. Heat can be stored through thermal energy storage technologies. For instance, the summer heat can be stored for winter heating. Similar principles are used to store winter cold for summer air conditioning.
Wind power
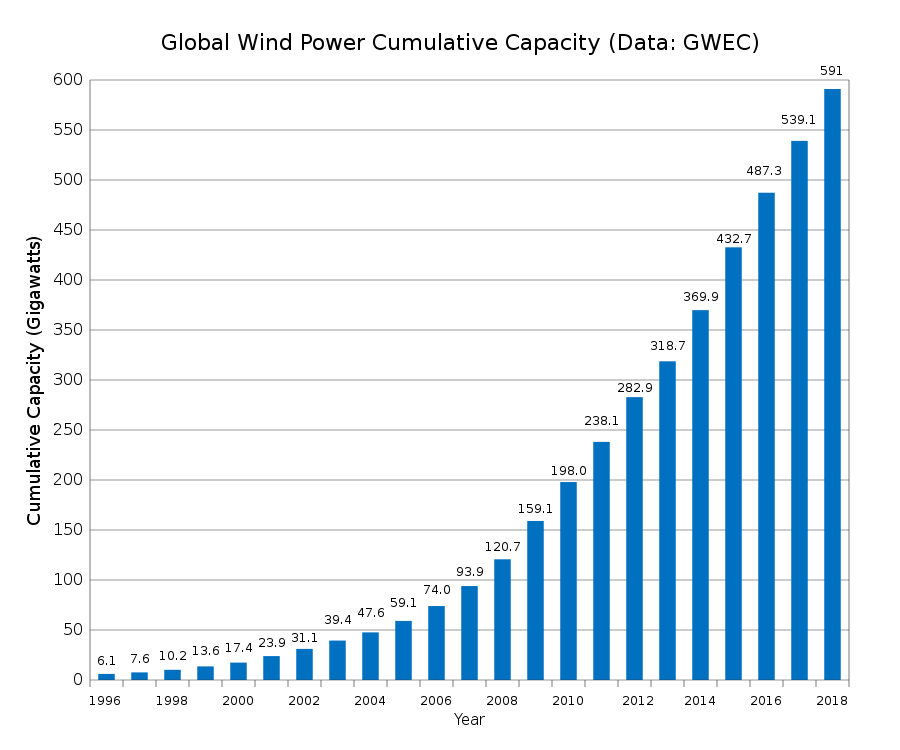
In 2018, wind power provided approximately 6% of the global electricity supply.[7] However, it may be difficult to site wind turbines in some areas for aesthetic or environmental reasons.[31] A large wind farm may consist of several hundred individual wind turbines, and cover an extended area of hundreds of square miles, but the land between the turbines may be used for agricultural or other purposes. A wind farm may also be located offshore.
After about 20 years wind turbine blades need replacing with larger blades, and research continues on how best to recycle them and how to manufacture blades which are easier to recycle.[42]
Hydropower
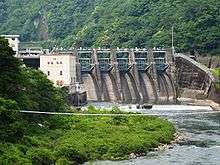
Among sources of renewable energy, hydroelectric plants have the advantages of being long-lived—many existing plants have operated for more than 100 years. Also, hydroelectric plants are clean, have few emissions and can compensate for variations in wind and solar power.[43] Criticisms directed at large-scale hydroelectric plants include: dislocation of people living where the reservoirs are planned, and release of greenhouse gases during construction and flooding of the reservoir.[44]
However, it has been found that high emissions are associated only with shallow reservoirs in warm (tropical) locales, and recent innovations in hydropower turbine technology are enabling efficient development of low-impact run-of-the-river hydroelectricity projects.[45] Generally speaking, hydroelectric plants produce much lower life-cycle emissions than other types of generation.
In 2015, hydropower supplied 16% of the world's electricity, down from a high of nearly 20% in the mid-to late 20th century.[46] It produced 60% of electricity in Canada and nearly 80% in Brazil.[46] As of 2017, new hydropower construction has stopped or slowed down since 1980 in most countries except China.[46]
Biomass
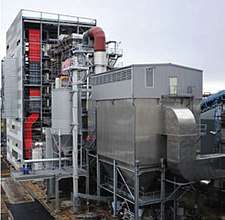
Biomass is biological material derived from living, or recently living organisms. As an energy source, biomass can either be burned to produce heat and to generate electricity or converted to modern biofuels such as biodiesel and ethanol.
Biomass is extremely versatile and one of the most-used sources of renewable energy. It is available in many countries, which makes it attractive for reducing dependence on imported fossil fuels. If the production of biomass is well-managed, carbon emissions can be significantly offset by the absorption of carbon dioxide by the plants during their lifespans. However this "carbon debt" may be paid back too late, or (especially in the United States) not properly accounted for.[47] If the biomass source is agricultural or municipal waste, burning it or converting it into biogas also provides a way to dispose of this waste.[48] Bioenergy production can be combined with carbon capture and storage to create a zero-carbon or negative-carbon system, but it is doubtful this can be scaled up quickly enough.[49]
If biomass is harvested from crops, such as tree plantations, the cultivation of these crops can displace natural ecosystems, degrade soils, and consume water resources and synthetic fertilizers.[48][50] In some cases, these impacts can actually result in higher overall carbon emissions compared to using petroleum-based fuels.[50][51]
Biofuels
Biofuels are fuels, such as ethanol, manufactured from various types of biomass, such as corn or sugar beet. Biofuels are usually liquid and used to power transport, often blended with liquid fossil fuels such as gasoline, diesel or kerosene. As of 2020 which are sustainable biofuel is being debated.
Cellulosic ethanol has many benefits over traditional corn based-ethanol. It does not take away or directly conflict with the food supply because it is produced from wood, grasses, or non-edible parts of plants.[52] Moreover, some studies have shown cellulosic ethanol to be potentially more cost effective and economically sustainable than corn-based ethanol.[53] As of 2018, efforts to commercialize production of cellulosic ethanol have been mostly disappointing, but new commercial efforts are continuing.[54][55]
Use of farmland for growing fuel can result in less land being available for growing food. Since photosynthesis is inherently inefficient, and crops also require significant amounts of energy to harvest, dry, and transport, the amount of energy produced per unit of land area is very small, in the range of 0.25 W/m2 to 1.2 W/m2.[56] In the United States, corn-based ethanol has replaced less than 10% of motor gasoline use since 2011, but has consumed around 40% of the annual corn harvest in the country.[50] In Malaysia and Indonesia, the clearing of forests to produce palm oil for biodiesel has led to serious social and environmental effects, as these forests are critical carbon sinks and habitats for endangered species.[57] In 2015, annual global production of liquid biofuels was equivalent to 1.8% of the energy extracted from crude oil.[46] It has been suggested that, due to the limited quantities that can be sustainably produced, it should all be aviation biofuel: because unlike other forms of transport long-distance aviation cannot be powered by batteries, hydrogen, ammonia or fuel cells.[58]
Geothermal
Geothermal energy is produced by tapping into the thermal energy created and stored within the earth. It arises from the radioactive decay of an isotope of potassium and other elements found in the Earth's crust.[59] Geothermal energy can be obtained by drilling into the ground, very similar to oil exploration, and then it is carried by a heat-transfer fluid (e.g. water, brine or steam).[59] Geothermal systems that are mainly dominated by water have the potential to provide greater benefits to the system and will generate more power.[60] Within these liquid-dominated systems, there are possible concerns of subsidence and contamination of ground-water resources. Therefore, protection of ground-water resources is necessary in these systems. This means that careful reservoir production and engineering is necessary in liquid-dominated geothermal reservoir systems.[60] Geothermal energy is considered sustainable because that thermal energy is constantly replenished.[61]
Geothermal energy can be harnessed to for electricity generation and for heating. Technologies in use include dry steam power stations, flash steam power stations and binary cycle power stations. As of 2010, geothermal electricity generation is used in 24 countries,[62] while geothermal heating is in use in 70 countries.[63] International markets grew at an average annual rate of 5 percent over the three years to 2015.[64]
Geothermal power is considered to be a sustainable, renewable source of energy because the heat extraction is small compared with the Earth's heat content.[65] The greenhouse gas emissions of geothermal electric stations are on average 45 grams of carbon dioxide per kilowatt-hour of electricity, or less than 5 percent of that of conventional coal-fired plants.[63]
Marine energy
Marine energy is mainly tidal power and wave power. As of 2020, a few small tidal power plants are operating in France and China,[66] and engineers continue to try and make wave power equipment more robust against storms.[67]
Non-renewable energy sources
Nuclear power
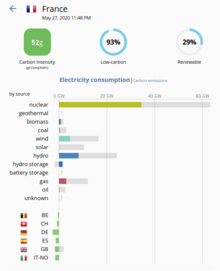
Sustainability of nuclear power based on nuclear fission is debated as the technology widely used today consumes mined uranium ore which is a non-renewable resource. However, since the amount of nuclear energy per unit of fuel is million times larger than chemical energy of any other fuel, the amounts of fuel used in nuclear fission are proportionally smaller. Due to relatively small fuel usage, high surface power density, high spent fuel recycling rate (up to 96%) and vast reserves, nuclear power can be considered as practically sustainable in scale of hundreds of years.[68]
Nuclear power plants have been used since the 1950s to produce a zero emission, steady supply of electricity, without creating local air pollution. In 2012, nuclear power plants in 30 countries generated 11% of global electricity.[69] The IPCC considers nuclear power to be a low-carbon energy source, with lifecycle greenhouse gas emissions (including the mining and processing of uranium), similar to the emissions from renewable energy sources.[70] As of 2020 nuclear power provides 50% of European Union low-carbon electricity and 26% of total energy production in Europe.[71]
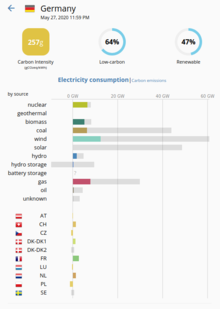
2 emissions in Germany as of 27 May 2020 with overall CO
2intensity of 257 gCO2eq/kWh. Source: electricitymap.org
The anti-nuclear movement has led to a decrease in the contribution of nuclear energy to the global electricity supply since 1993.[72] Public support for nuclear energy is often low as a result of safety concerns,[73] however for each unit of energy produced, nuclear energy is far safer than fossil fuel energy.[74] Traditional environmental groups such as Greenpeace and the Sierra Club are opposed to all use of nuclear power.[75] Individuals who have described nuclear power as a green energy source include Stewart Brand,[75] George Monbiot,[76] Bill Gates,[77] James Lovelock[78] and director of Greenpeace UK (2001-2007) Stephen Tindale.[79]
In 2018 nuclear power was chosen by European Union as part of the low-carbon European power system.[6]
Future designs
Newer nuclear reactor designs are capable of extracting energy from nuclear waste until it is no longer (or dramatically less) dangerous, and have design features that greatly minimize the possibility of a nuclear accident. These designs (for instance the molten salt reactor) have yet to be commercialized. Some other reactors, such as the Integral Fast Reactor, can "burn" nuclear waste through a process known as nuclear transmutation. Nuclear power plants can be more or less eliminated from their problem of nuclear waste through the use of nuclear reprocessing and newer plants such as fast breeder plants.
Thorium is a fissionable material used in thorium-based nuclear power. The thorium fuel cycle claims several potential advantages over a uranium fuel cycle, including greater abundance, superior physical and nuclear properties, better resistance to nuclear weapons proliferation[80][81][82] and reduced plutonium and actinide production.[82] Therefore, it is sometimes referred as sustainable.[83] Safety assessment of already operating nuclear power plants to extend their lifetimes, perhaps up to 80 years,[84] continues.[85] Regardless of past accidents, nuclear power remains the safest energy source available per unit of energy as compared to other sources.[86]
Fusion
A prospective energy source is nuclear fusion (as opposed to nuclear fission used today). It is the reaction that exists in stars, including the Sun. Fusion reactors currently in construction (ITER) are expected to be inherently safe due to lack of chain reaction and do not produce long-lived nuclear waste.[87] The fuel for nuclear fusion reactors are very widely available deuterium, lithium and tritium.[88]
Carbon capture and storage
In theory, the greenhouse gas emissions of fossil fuel and biomass power plants can be significantly reduced through carbon capture and storage, although this process is expensive. According to the Intergovernmental Panel on Climate Change, the lowest cost path to meeting the 2 °C target includes massive deployment of one specific type of negative emissions technology called bioenergy with carbon capture and sequestration, or BECCS.[89] However, achieving this target through BECCS requires more resources than are currently available worldwide. For example, to capture 10 billion tons of CO2 per year (GtCO2/y) would require biomass from 40 percent of the world's current cropland.[90]
Managing intermittent energy sources
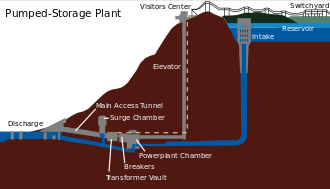
Solar and wind are variable renewable energy (VRE) sources that supply electricity intermittently depending on the weather and the time of day. Overall intermittency can be reduced by combining these sources.[91][92]
Most electric grids were constructed for non-intermittent energy sources such as coal-fired power plants. According to the International Energy Agency: "Power system flexibility is required across a range of timescales, and different flexibility hardware solutions and operational practices solutions offer timescale-specific capabilities".[93] Half the world's electricity will need to be wind and solar by 2030, to limit the global rise in temperature to well below 2 °C by 2050.[94] As larger amounts of solar and wind energy are integrated into the grid, it becomes necessary to make changes to the overall system to ensure that the supply of electricity is matched to demand.These changes can include the following:
- Using hydroelectricity, natural gas plants, or other fossil fuel or nuclear plants to produce backup power
- Using grid energy storage to store excess solar and wind energy and release it as needed. The most commonly used storage method is pumped-storage hydroelectricity, which is feasible only at locations that are next to a large hill or a deep underground mine. Batteries are being deployed widely. Other storage technologies such as power-to-gas have been used in limited situations.[95]
- Trading electricity with other locations in a regional grid or through long-distance transmission lines
- Reducing demand for electricity at certain times through energy demand management and use of smart grids.
- Energy market, or more specifically electricity market, changes so that power supply flexibility is better paid[96][97]
As of 2019, the cost and logistics of energy storage for large population centers is a significant challenge, although the cost of battery systems has plunged dramatically.[98] For instance, a 2019 study found that for solar and wind energy to replace all fossil fuel generation for a week of extreme cold in the eastern and midwest United States, energy storage capacity would have to increase from the 11 GW in place at that time to between 230 GW and 280 GW, depending on how much nuclear power is retired.[98]
Pumped-storage as well as load following coal, fossil gas and nuclear power plants are the most widespread techniques for balancing intermittent energy sources as of 2020.
As of 2020 in the European Union this has become the de facto standard in countries which decided to shut down their zero-emission nuclear power plants such as Germany.[99][100][101][102] This practice has led to a debate between Germany, which made significant investments in fossil gas imports but failed its CO
2 emission reduction goals,[103] and France, whose energy mix is largely composed of nuclear power and whose average CO2 emissions from energy sector are 5x smaller than Germany.[104][105]
Energy efficiency
Moving towards energy sustainability will require changes not only in the way energy is supplied, but in the way it is used, and reducing the amount of energy required to deliver various goods or services is essential. Opportunities for improvement on the demand side of the energy equation are as rich and diverse as those on the supply side, and often offer significant economic benefits.[106]
Efficiency slows down energy demand growth so that rising clean energy supplies can make deep cuts in fossil fuel use. A recent historical analysis has demonstrated that the rate of energy efficiency improvements has generally been outpaced by the rate of growth in energy demand, which is due to continuing economic and population growth. As a result, despite energy efficiency gains, total energy use and related carbon emissions have continued to increase. Thus, given the thermodynamic and practical limits of energy efficiency improvements, slowing the growth in energy demand is essential.[107] However, unless clean energy supplies come online rapidly, slowing demand growth will only begin to reduce total emissions; reducing the carbon content of energy sources is also needed. Any serious vision of a sustainable energy economy thus requires commitments to both renewables and efficiency.[108]
Trends
As of 2019 fossil fuels still supply over 80% of world energy consumption and, although energy consumption per person is expected to peak in the 2020s, the use of sustainable energy is not increasing fast enough to meet the 2 degree goal of the Paris Agreement.[109]
As of 2019 forecasts for the 400 GW of nuclear power capacity, which in 2018 supplied 10% of the world's electricity, vary from an 8% decrease to a 25% increase by 2030.[110]
In 2020, the International Energy Agency warned that the economic turmoil caused by the coronavirus outbreak may prevent or delay companies from investing in green energy. [111][112][113] The outbreak could potentially spell a slowdown in the world’s clean energy transition if no action is undertaken.[114]
Fuel switching from coal to natural gas
On average for a given unit of energy produced, the greenhouse gas emissions of natural gas are around half the emissions of coal when used to generate electricity, and around two-thirds the emissions of coal when used to produce heat: however reducing methane leaks is imperative.[115] Natural gas also produces significantly less air pollution than coal. Building gas-fired power plants and gas pipelines is therefore promoted as a way to reduce emissions and phase out coal use, however this practice is controversial. Opponents argue that developing natural gas infrastructure will create decades of carbon lock-in and stranded assets, and that renewables create far less emissions at comparable costs.[116] The life-cycle greenhouse-gas emissions of natural gas are around 40 times the emissions of wind and nuclear energy.
Electrification
Despite continued electrification fossil fuels may still be providing over two thirds of world energy by 2040.[109]
Government energy policies
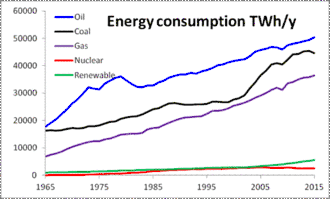
According to the IPCC, both explicit carbon pricing and complementary energy-specific policies are necessary mechanisms to limit global warming to 1.5 °C.[118]
Energy-specific programs and regulations have historically been the mainstay of efforts to reduce fossil fuel emissions.[119] Successful cases include the building of nuclear reactors in France in the 1970s and 1980s, and fuel efficiency standards in the United States which conserved billions of barrels of oil.[119] Other examples of energy-specific policies include energy-efficiency requirements in building codes, banning new coal-fired electricity plants, performance standards for electrical appliances, and support for electric vehicle use.[120][118] Nevertheless fossil fuel subsidies remain a key barrier to a transition to a clean energy system.[121]
Carbon taxes are an effective way to encourage movement towards a low-carbon economy, while providing a source of revenue that can be used to lower other taxes[122] or to help lower-income households afford higher energy costs.[123] Carbon taxes have encountered strong political pushback in some jurisdictions, whereas energy-specific policies tend to be politically safer.[119] According to the OECD climate change cannot be curbed without carbon taxes on energy, but 70% of energy-related CO2 emissions were not taxed at all in 2018.[124] Some studies estimate that combining a carbon tax with energy-specific policies would be more cost-effective than a carbon tax alone.[118]
Sustainable energy research
There are numerous organizations within the academic, federal, and commercial sectors conducting large scale advanced research in the field of sustainable energy. Scientific production towards sustainable energy systems is rising exponentially, growing from about 500 English journal papers only about renewable energy in 1992 to almost 9,000 papers in 2011.[125]
Hydrogen
Hydrogen is a zero-emission fuel that can be produced by using electrolysis to split water molecules into hydrogen and oxygen. Hydrogen can play a role in a sustainable energy system if the electricity used to produce it is generated from sustainable sources, such as wind or solar. Hydrogen can be produced when there is a surplus of intermittent renewable electricity, then stored and used to generate heat or to re-generate electricity. Hydrogen can be distributed by ship[126] or through pipelines. Up to 20% can be mixed into natural gas pipelines without changing pipelines or appliances,[127] but as hydrogen is so light this would only save 7% of emissions.[128] As of 2020 trials are underway on how to convert a natural gas grid to 100% hydrogen,[129] in order to reduce or eliminate emissions from residential and industrial natural gas heating.[130] It can be used to power vehicles that have hydrogen fuel cells.[131] As it has a low energy to volume content, it is easier to use in hydrogen-powered ships or heavy road vehicles[132] than in cars and airplanes.
As of 2018, very little of the world's supply of hydrogen is created from sustainable sources. Nearly all hydrogen is created by steam methane reforming (SMR), which results in high greenhouse gas emissions but is currently cheaper than creating hydrogen via electrolysis. Although some carbon from SMR could be captured the process can be made more sustainable by using autothermal reforming with carbon capture and storage technologies to remove most of the carbon dioxide that is emitted.[130]
See also
- Environmental impact of the energy industry
- Fossil fuel divestment
- Life-cycle greenhouse-gas emissions of energy sources
- Sustainable Energy for All initiative
References
- Kutscher, Milford & Kreith 2018.
- Renewable Energy & Efficiency Partnership (August 2004). "Glossary of terms in sustainable energy regulation" (PDF). Retrieved 19 December 2008.
- Committee on Climate Change (November 2018). "Hydrogen in a low-carbon economy" (PDF). Retrieved 31 December 2019.
- "Debate: is nuclear clean enough for the EU's green funding?". Power Technology. 16 January 2020.
- McCurry, Justin (12 September 2019). "Japan should scrap nuclear reactors after Fukushima, says new environment minister". The Guardian. ISSN 0261-3077. Retrieved 5 March 2020.
- "6_EN_ACT_part1_v11.docx". eur-lex.europa.eu. Retrieved 27 May 2020.
- "Wind & Solar Share in Electricity Production Data | Enerdata". Power Technology.
- World Commission on Environment and Development (1987). "Chapter 7: Energy: Choices for Environment and Development". Our Common Future: Report of the World Commission on Environment and Development. Oxford New York: Oxford University Press. ISBN 978-0-19-282080-8. OCLC 15489268.
- James, Paul; Magee, Liam; Scerri, Andy; Steger, Manfred B. (2015). Urban Sustainability in Theory and Practice. London: Routledge.; Liam Magee; Andy Scerri; Paul James; Jaes A. Thom; Lin Padgham; Sarah Hickmott; Hepu Deng; Felicity Cahill (2013). "Reframing social sustainability reporting: Towards an engaged approach". Environment, Development and Sustainability. Springer.
- Evans, Robert L. (2007). Fueling our future : an introduction to sustainable energy. Cambridge: Cambridge University Press. pp. 3. ISBN 9780521865630. OCLC 144595567.
- "The Global Energy Challenge". World Bank Blogs. Retrieved 27 September 2019.
- "Q&A: Bill Gates on the World's Energy Crisis". WIRED. 19 (7). 20 June 2011. Retrieved 2 October 2019.
- Hannah Ritchie (2019). "Access to Energy". OurWorldInData.org. Retrieved 5 July 2020.
- "7 million premature deaths annually linked to air pollution". WHO. 25 March 2014. Retrieved 30 September 2019.
- "Goal 7—Ensure Access to Affordable, Reliable, Sustainable and Modern Energy for All". UN Chronicle. 8 April 2015. Retrieved 27 September 2019.
- "Global Historical Emissions". Climate Watch. Retrieved 28 September 2019.
- World Resources Institute (June 2015). "CAIT Country Greenhouse Gas Emissions: Sources and Methods" (PDF). Retrieved 28 September 2019.
- "Fossil fuel energy consumption (% of total)". World Bank Open Data (in Indonesian). Retrieved 27 September 2019.
- "Access to clean cooking – SDG7: Data and Projections – Analysis". IEA. Retrieved 28 December 2019.
- "These cheap, clean stoves were supposed to save millions of lives. What happened?". Washington Post. 29 October 2015. Retrieved 1 March 2019.
- Tester 2012, p. 504.
- Loftus, Peter J.; Cohen, Armond M.; Long, Jane C. S.; Jenkins, Jesse D. (2015). "A critical review of global decarbonization scenarios: what do they tell us about feasibility?" (PDF). Wiley Interdisciplinary Reviews: Climate Change. 6: 93–112. doi:10.1002/wcc.324.
- SR15 Summary for policymakers.
- SR15, C.2.4.2.2.
- SR15, C.2.4.2.1, Table 2.6 low-OS.
- SR15, 2.4.2.1, Table 2.6 low-OS.
- SR15, p. 111.
- SR15, 2.4.2.1.
- SR15, 2.4.2.2.
- SR15, 2.4.3.
- International Energy Agency (2007). Renewables in global energy supply: An IEA facts sheet, OECD, 34 pages. Archived 12 October 2009 at the Wayback Machine
- admin. "How Long Do Solar Panels Last and Replacement Guide". Those Solar Guys. Retrieved 31 December 2019.
- "Waste take-back, treatment & legal compliance | PV CYCLE Association". pvcycle.org. Retrieved 31 December 2019.
- "NREL Photovoltaic Efficiency Chart". NREL. Retrieved 19 April 2017.
- "'Major discovery' from MIT primed to unleash solar revolution". MIT News. Retrieved 17 April 2012.
- Collings AF and Critchley C. Artificial Photosynthesis- from Basic Biology to Industrial Application. WWiley-VCH. Weinheim (2005) p xi
- Faunce, Thomas A.; Lubitz, Wolfgang; Rutherford, A. W. (Bill); MacFarlane, Douglas; Moore, Gary F.; Yang, Peidong; Nocera, Daniel G.; Moore, Tom A.; Gregory, Duncan H.; Fukuzumi, Shunichi; Yoon, Kyung Byung; Armstrong, Fraser A.; Wasielewski, Michael R.; Styring, Stenbjorn (2013). "Energy and environment policy case for a global project on artificial photosynthesis". Energy & Environmental Science. 6 (3): 695. doi:10.1039/C3EE00063J.
- "Solar farms in space could be renewable energy's next frontier". NBC News. Retrieved 4 June 2019.
- Solar water heating energy.gov
- "Solar assisted air-conditioning of buildings". Archived from the original on 5 November 2012. Retrieved 5 November 2012.
- "Global Wind Report Annual Market Update". Gwec.net. Retrieved 21 August 2013.
- "Critical question: How to recycle 12 000 wind turbines? • Recycling International". Recycling International. 12 July 2019. Retrieved 31 December 2019.
- Opperman, Jeff. "Moving To The System Scale Can Improve Hydropower". Forbes. Retrieved 4 February 2020.
- Almeida, Rafael M.; Shi, Qinru; Gomes-Selman, Jonathan M.; Wu, Xiaojian; Xue, Yexiang; Angarita, Hector; Barros, Nathan; Forsberg, Bruce R.; García-Villacorta, Roosevelt; Hamilton, Stephen K.; Melack, John M. (19 September 2019). "Reducing greenhouse gas emissions of Amazon hydropower with strategic dam planning". Nature Communications. 10 (1): 4281. Bibcode:2019NatCo..10.4281A. doi:10.1038/s41467-019-12179-5. ISSN 2041-1723. PMC 6753097. PMID 31537792.
- Ferris, David (3 November 2011). "The Power of the Dammed: How Small Hydro Could Rescue America's Dumb Dams". Retrieved 4 January 2012.
- Smil 2017b, p. 286.
- Elbein, Saul (4 March 2019). "Europe's renewable energy policy is built on burning American trees". Vox. Retrieved 1 January 2020.
- Tester 2012, p. 512.
- Ambrose, Jillian (10 December 2019). "Drax owner plans to be world's first carbon-negative business". The Guardian. ISSN 0261-3077. Retrieved 1 January 2020.
- Smil 2017a, p. 162.
- Edenhofer 2014, p. 616.
- M.R. Schmer; K.P. Vogel; R.B. Mitchell; R.K. Perrin (2008). "Net energy of cellulosic ethanol from switchgrass". Proceedings of the National Academy of Sciences of the United States of America. 105 (2): 464–469. Bibcode:2008PNAS..105..464S. doi:10.1073/pnas.0704767105. PMC 2206559. PMID 18180449.
- Charles E. Wyman (2007). "What is (and is not) vital to advancing cellulosic ethanol". Trends in Biotechnology. 25 (4): 153–157. doi:10.1016/j.tibtech.2007.02.009. PMID 17320227.
- Rapier, Robert. "Cellulosic Ethanol Falling Far Short Of The Hype". Forbes. Retrieved 6 June 2019.
- "Clariant bets big on cellulosic ethanol". Chemical & Engineering News. Retrieved 6 June 2019.
- Smil 2017a, p. 161.
- Lustgarten, Abrahm (20 November 2018). "Palm Oil Was Supposed to Help Save the Planet. Instead It Unleashed a Catastrophe". The New York Times. ISSN 0362-4331. Retrieved 15 May 2019.
- "Biomass in a low-carbon economy". Committee on Climate Change. Retrieved 28 December 2019.
- László, Erika (1981). "Geothermal Energy: An Old Ally". Ambio. 10 (5): 248–249. JSTOR 4312703.
- Dorfman, Myron H. (July 1976). "Water Required to Develop Geothermal Energy". Journal (American Water Works Association). 68 (7): 370–375. doi:10.1002/j.1551-8833.1976.tb02435.x. JSTOR 41268497.
- L. Ryback (2007). "Geothermal Sustainability". GHC Bulletin: 2–6.
- Geothermal Energy Association. Geothermal Energy: International Market Update May 2010, p. 4-6.
- Moomaw, W., P. Burgherr, G. Heath, M. Lenzen, J. Nyboer, A. Verbruggen, 2011: Annex II: Methodology. In IPCC: Special Report on Renewable Energy Sources and Climate Change Mitigation (ref. page 10)
- "The International Geothermal Market At a Glance – May 2015" (PDF). GEA—Geothermal Energy Association. May 2015.
- Rybach, Ladislaus (September 2007), "Geothermal Sustainability" (PDF), Geo-Heat Centre Quarterly Bulletin, Klamath Falls, Oregon: Oregon Institute of Technology, 28 (3), pp. 2–7, ISSN 0276-1084, retrieved 9 May 2009
- Smil 2017b, p. 288.
- Peters, Adele (16 April 2019). "Could wave power be the next boom in renewable energy?". Fast Company. Retrieved 6 February 2020.
- "Ch 24 Page 162: Sustainable Energy - without the hot air | David MacKay". withouthotair.com. Retrieved 26 June 2020.
- Bruckner 2014, p. 530.
- "IPCC Working Group III – Mitigation of Climate Change, Annex III: Technology - specific cost and performance parameters" (PDF). IPCC. 2014. p. 7. Retrieved 14 December 2018.
- "Electricity Generation". FORATOM. Retrieved 27 May 2020.
- Bruckner 2014, p. 517.
- Armaroli, Nicola; Balzani, Vincenzo (2011). "Towards an electricity-powered world. In". Energy and Environmental Science. 4 (9): 3193–3222. doi:10.1039/c1ee01249e.
- "It goes completely against what most believe, but out of all major energy sources, nuclear is the safest". Our World in Data. Retrieved 29 August 2019.
- Pinker, Steven (2018). Enlightenment now : the case for reason, science, humanism, and progress. New York, New York. p. 881. ISBN 9780525427575. OCLC 993692045.
- Monbiot, George (20 February 2009). "George Monbiot: A kneejerk rejection of nuclear power is not an option | Environment". London: theguardian.com. Retrieved 21 August 2013.
- "Has Bill Gates come up with a safe, clean way to harness nuclear power?". The Independent. Retrieved 9 January 2013.
- Lovelock, James (2006). The Revenge of Gaia. Reprinted Penguin, 2007. ISBN 978-0-14-102990-0
- Brand, Stewart (2010). Whole Earth Discipline.
- Kang, J.; Von Hippel, F. N. (2001). "U‐232 and the proliferation‐resistance of U‐233 in spent fuel". Science & Global Security. 9 (1): 1. Bibcode:2001S&GS....9....1K. doi:10.1080/08929880108426485. "Archived copy" (PDF). Archived from the original (PDF) on 3 December 2014. Retrieved 2 March 2015.CS1 maint: archived copy as title (link)
- Nuclear Materials FAQ
- Robert Hargraves; Ralph Moir (January 2011). "Liquid Fuel Nuclear Reactors". American Physical Society Forum on Physics & Society. Retrieved 31 May 2012.
- "Th-ING: A Sustainable Energy Source | National Security Science Magazine | Los Alamos National Laboratory". lanl.gov. 2015. Retrieved 1 March 2015.
- "Going Long Term: US Nuclear Power Plants Could Extend Operating Life to 80 Years". iaea.org. 16 January 2018. Retrieved 31 December 2019.
- Ambrose, Jillian (20 August 2019). "Nuclear regulator permits restarting of reactor 4 at Hunterston B". The Guardian. ISSN 0261-3077. Retrieved 31 December 2019.
- "What are the safest sources of energy?". Our World in Data. Retrieved 27 May 2020.
- Fernandez, Elizabeth. "The US Comes One Step Closer To Producing Commercial Fusion Power". Forbes. Retrieved 30 May 2020.
- "Ch 24 Page 172: Sustainable Energy - without the hot air | David MacKay". withouthotair.com. Retrieved 26 June 2020.
- Edenhofer, O.; Pichs-Madruga, R.; et al. (2014). Climate Change 2014: Mitigation of Climate Change. Contribution of Working Group III to the Fifth Assessment Report of the Intergovernmental Panel on Climate Change. Cambridge, U.K.: Cambridge University Press. ISBN 9781107654815.
- National Academies Of Sciences, Engineering (2019). Negative Emissions Technologies and Reliable Sequestration: A Research Agenda. Washington, D.C.: National Academies of Sciences, Engineering, and Medicine. p. 3. doi:10.17226/25259. ISBN 978-0-309-48452-7. PMID 31120708.
- Jerez, Sonia; Tobin, Isabelle; Turco, Marco; María López-Romero, Jose; Montávez, Juan Pedro; Jiménez-Guerrero, Pedro; Vautard, Robert (2018). "Resilience of the combined wind-plus-solar power production in Europe to climate change: a focus on the supply intermittence". EGUGA: 15424. Bibcode:2018EGUGA..2015424J.
- Lave, M.; Ellis, A. (2016). "Comparison of solar and wind power generation impact on net load across a utility balancing area". 2016 IEEE 43rd Photovoltaic Specialists Conference (PVSC): 1837–1842. doi:10.1109/PVSC.2016.7749939. ISBN 978-1-5090-2724-8. OSTI 1368867.
- "Introduction to System Integration of Renewables – Analysis". IEA. Retrieved 30 May 2020.
- "8 times more wind and solar power needed by 2030 to help meet Paris climate target, DNV GL finds".
- Ortiz, Diego Arguedas. "How hydrogen is transforming these tiny Scottish islands". bbc.com. Retrieved 28 December 2019.
- "World's 'largest, most ambitious' energy flexibility market trials to launch in the UK". Current. Retrieved 4 June 2020.
- "U.S. regulatory innovation to boost power system flexibility and prepare for ramp up of wind and solar – Analysis". IEA. Retrieved 4 June 2020.
- "100% Renewable Energy Needs Lots of Storage. This Polar Vortex Test Showed How Much". InsideClimate News. 20 February 2019. Retrieved 4 June 2019.
- Simon, Frédéric (29 May 2020). "Natural gas is a 'caveat' in energy transition, EU admits". www.euractiv.com. Retrieved 29 May 2020.
- "Speech by Federal Chancellor Angela Merkel at the 49th World Economic Forum Annual Meeting in Davos on 23 January 2019". Home Page. Retrieved 29 May 2020.
- "StackPath". www.uniper.energy. Retrieved 29 May 2020.
- Szalai, Pavol (13 January 2020). "Austrian Green MEP: Gas is a better transition alternative to coal than nuclear". www.euractiv.com. Retrieved 29 May 2020.
- Welle (www.dw.com), Deutsche. "Germany to fall short of 2020 climate goals: report | DW | 06.02.2019". DW.COM. Retrieved 29 May 2020.
- Barbière, Cécile (27 November 2019). "Paris, Berlin divided over nuclear's recognition as green energy". www.euractiv.com. Retrieved 29 May 2020.
- "Letter: EU must include nuclear power in its list of sustainable sources | Financial Times". www.ft.com. 15 December 2019. Retrieved 29 May 2020.
- InterAcademy Council (2007). Lighting the way: Toward a sustainable energy future p. xvii.
- Huesemann, Michael H., and Joyce A. Huesemann (2011). Technofix: Why Technology Won’t Save Us or the Environment, Chapter 5, "In Search of Solutions: Efficiency Improvements", New Society Publishers, ISBN 978-0-86571-704-6.
- American Council for an Energy-Efficient Economy (2007). The Twin Pillars of Sustainable Energy: Synergies between Energy Efficiency and Renewable Energy Technology and Policy Report E074.
- "World Energy Scenarios" (PDF). World Energy Council. 2019.
- "IAEA Releases New Projections for Nuclear Power Through 2050". International Atomic Energy Agency. 10 September 2019. Retrieved 27 January 2020.
- Newburger, Emma (13 March 2020). "Coronavirus could weaken climate change action and hit clean energy investment, researchers warn". CNBC. Retrieved 16 March 2020.
- "Text-Only NPR.org : Climate Change Push Fuels Split On Coronavirus Stimulus". NPR.
- "Put clean energy at the heart of stimulus plans to counter the coronavirus crisis—Analysis". IEA.
- "Coronavirus poses threat to climate action, says watchdog". The Guardian.
- "The Role of Gas: Key Findings". International Energy Agency. 4 October 2019. Retrieved 4 October 2019.
- "As Coal Fades in the U.S., Natural Gas Becomes the Climate Battleground". The New York Times. 26 June 2019. Retrieved 4 October 2019.
- Statistical Review of World Energy, Workbook (xlsx), London, 2016
- SR15, 2.5.2.1.
- Plumer, Brad (8 October 2018). "New U.N. Climate Report Says Put a High Price on Carbon". The New York Times. ISSN 0362-4331. Retrieved 4 October 2019.
- Lathia, Rutvik Vasudev; Dadhaniya, Sujal (February 2017). "Policy formation for Renewable Energy sources". Journal of Cleaner Production. 144: 334–336. doi:10.1016/j.jclepro.2017.01.023.
- "Fossil Fuel to Clean Energy Subsidy Swaps: How to pay for an energy revolution" (PDF). International Institute for Sustainable Development. June 2019.
- "Revenue-Neutral Carbon Tax | Canada | UNFCCC". unfccc.int. Retrieved 28 October 2019.
- Carr, Mathew (10 October 2018). "How High Does Carbon Need to Be? Somewhere From $20-$27,000". Retrieved 4 October 2019.
- "Taxes on polluting fuels are too low to encourage a shift to low-carbon alternatives - OECD". www.oecd.org. Retrieved 30 May 2020.
- Rizzi; et al. (2014). "The production of scientific knowledge on renewable energies: Worldwide trends, dynamics and challenges and implications for management. In". Renewable Energy. 62: 657–671. doi:10.1016/j.renene.2013.08.030.
- "Japan launches first liquid hydrogen carrier ship". ft.com. Retrieved 1 January 2020.
- Harrabin, Roger (2 January 2020). "Climate change hope for hydrogen fuel". Retrieved 2 January 2020.
- "Drax Electric Insights Quarterly – Q4 2019" (PDF).
- "H21". Retrieved 31 December 2019.
- "Transitioning to hydrogen: Assessing the engineering risks and uncertainties". www.theiet.org. Retrieved 11 April 2020.
- Lathia, Rutvik Vasudev; Dobariya, Kevin S.; Patel, Ankit (January 2017). "Hydrogen Fuel Cells for Road Vehicles". Journal of Cleaner Production. 141: 462. doi:10.1016/j.jclepro.2016.09.150.
- "Hydrogen Fuel Cell trucks can decarbonise heavy transport". Energy Post. 17 October 2019. Retrieved 1 January 2020.
Bibliography
- Bruckner, T.; et al. (2014). "Chapter 7: Energy Systems" (PDF). Intergovernmental Panel on Climate Change Fifth Assessment Report 2014. pp. 511–597.
- Edenhofer, Ottmar (2014). Climate Change 2014: Mitigation of Climate Change : Working Group III contribution to the Fifth Assessment Report of the Intergovernmental Panel on Climate Change. New York, NY: Cambridge University Press. ISBN 978-1-107-05821-7. OCLC 892580682.CS1 maint: ref=harv (link)
- IPCC, 2018: Global Warming of 1.5°C. An IPCC Special Report on the impacts of global warming of 1.5°C above pre-industrial levels and related global greenhouse gas emission pathways, in the context of strengthening the global response to the threat of climate change, sustainable development, and efforts to eradicate poverty [V. Masson-Delmotte, P. Zhai, H. O. Pörtner, D. Roberts, J. Skea, P.R. Shukla, A. Pirani, W. Moufouma-Okia, C. Péan, R. Pidcock, S. Connors, J. B. R. Matthews, Y. Chen, X. Zhou, M. I. Gomis, E. Lonnoy, T. Maycock, M. Tignor, T. Waterfield (eds.)].
- Report website, chapters I–V
- Summary for policymakers, 32 pp.
- Headline statements, 2 pp.
- Technical summary, 22 pp.
- FAQ, 24 pp.
- Glossary, 24 pp.
- Kutscher, C.F.; Milford, J.B.; Kreith, F. (2018). Principles of Sustainable Energy Systems, Third Edition. Mechanical and Aerospace Engineering Series. CRC Press. ISBN 978-0-429-93916-7. Retrieved 10 February 2019.CS1 maint: ref=harv (link)
- Smil, Vaclav (2017a). Energy Transitions: Global and National Perspectives. Santa Barbara, California: Praeger, an imprint of ABC-CLIO, LLC. ISBN 978-1-4408-5324-1. OCLC 955778608.CS1 maint: ref=harv (link)
- Smil, Vaclav (2017b). Energy and Civilization : A History. Cambridge, Massachusetts: The MIT Press. ISBN 978-0-262-03577-4. OCLC 959698256.CS1 maint: ref=harv (link)
- Tester, Jefferson (2012). Sustainable Energy : Choosing Among Options. Cambridge, MA: MIT Press. ISBN 978-0-262-01747-3. OCLC 892554374.CS1 maint: ref=harv (link)