Bio-FET
A field-effect transistor-based biosensor, also known as a biosensor field-effect transistor (Bio-FET[1] or BioFET), field-effect biosensor (FEB),[2] or biosensor MOSFET,[3] is a field-effect transistor (based on the MOSFET structure)[3] that is gated by changes in the surface potential induced by the binding of molecules. When charged molecules, such as biomolecules, bind to the FET gate, which is usually a dielectric material, they can change the charge distribution of the underlying semiconductor material resulting in a change in conductance of the FET channel.[4][5] A Bio-FET consists of two main compartments: one is the biological recognition element and the other is the field-effect transistor.[1][6] The BioFET structure is largely based on the ion-sensitive field-effect transistor (ISFET), a type of metal-oxide-semiconductor field-effect transistor (MOSFET) where the metal gate is replaced by an ion-sensitive membrane, electrolyte solution and reference electrode.[7]
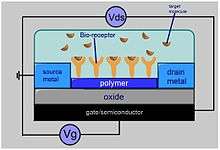
Mechanism of operation
Bio-FETs couple a transistor device with a bio-sensitive layer that can specifically detect bio-molecules such as nucleic acids and proteins. A Bio-FET system consists of a semiconducting field-effect transistor that acts as a transducer separated by an insulator layer (e.g. SiO2) from the biological recognition element (e.g. receptors or probe molecules) which are selective to the target molecule called analyte.[8] Once the analyte binds to the recognition element, the charge distribution at the surface changes with a corresponding change in the electrostatic surface potential of the semiconductor. This change in the surface potential of the semiconductor acts like a gate voltage would in a traditional MOSFET, i.e. changing the amount of current that can flow between the source and drain electrodes.[9] This change in current (or conductance) can be measured, thus the binding of the analyte can be detected. The precise relationship between the current and analyte concentration depends upon the region of transistor operation.[10]
Fabrication of Bio-FET
The fabrication of Bio-FET system consists of several steps as follows:
- Finding a substrate suitable for serving as a FET site, and forming a FET on the substrate,
- Exposing an active site of the FET from the substrate,
- Providing a sensing film layer on active site of FET,
- Providing a receptor on the sensing film layer in order to be used for ion detection,
- Removing a semiconductor layer, and thinning a dielectric layer,
- Etching the remaining portion of the dielectric layer to expose an active site of the FET,
- Removing the photoresist, and depositing a sensing film layer followed by formation of a photoresist pattern on the sensing film,
- Etching the unprotected portion of the sensing film layer, and removing the photoresist[11]
Advantages
The principle of operation of Bio-FET devices based on detecting changes in electrostatic potential due to binding of analyte. This the same mechanism of operation as glass electrode sensors which also detect changes in surface potential but were developed as early as the 1920s. Due to the small magnitude of the changes in surface potential upon binding of biomolecules or changing pH, glass electrodes require a high impedance amplifier which increases the size and cost of the device. In contrast, the advantage of Bio-FET devices is that they operate as an intrinsic amplifier, converting small changes in surface potential to large changes in current (through the transistor component) without the need for additional circuitry. This means BioFETs have the capability to be much smaller and more affordable than glass electrode-based biosensors. If the transistor is operated in the subthreshold region, then an exponential increase in current is expected for a unit change in surface potential.
Bio-FETs can be used for detection in fields such as medical diagnostics,[12][11] biological research, environmental protection and food analysis. Conventional measurements like optical, spectrometric, electrochemical, and SPR measurements can also be used to analyze biological molecules. Nevertheless, these conventional methods are relatively time-consuming and expensive, involving multi-stage processes and also not compatible to real-time monitoring,[13] in contrast to Bio-FETs. Bio-FETs are low weight, low cost of mass production, small size and compatible with commercial planar processes for large-scale circuitry. They can be easily integrated into digital microfluidic devices for Lab-on-a-chip. For example, a microfluidic device can control sample droplet transport whilst enabling detection of bio-molecules, signal processing, and the data transmission, using an all-in-one chip.[14] Bio-FET also does not require any labeling step,[13] and simply utilise a specific molecular (e.g. antibody, ssDNA[15]) on the sensor surface to provide selectivity. Some Bio-FETs display fascinating electronic and optical properties. An example FET would is a glucose-sensitive based on the modification of the gate surface of ISFET with SiO2 nanoparticles and the enzyme glucose oxidase (GOD); this device showed obviously enhanced sensitivity and extended lifetime compared with that without SiO2 nanoparticles.[16]
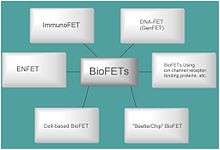
Optimization
The choice of reference electrode (liquid gate) or back-gate voltage determines the carrier concentration within the field effect transistor, and therefore its region of operation, therefore the response of the device can be optimised by tuning the gate voltage. If the transistor is operated in the subthreshold region then an exponential increase in current is expected for a unit change in surface potential. The response is often reported as the change in current on analyte binding divided by the initial current (), and this value is always maximal in the subthreshold region of operation due to this exponential amplification.[10][17][18][19] For most devices, optimum signal-to-noise, defined as change in current divided by the baseline noise, () is also obtained when operating in the subthreshold region,[10][20] however as the noise sources vary between devices, this is device dependent.[21]
One optimization of Bio-FET may be to put a hydrophobic passivation surface on the source and the drain to reduce non-specific biomolecular binding to regions which are not the sensing-surface.[22][23] Many other optimisation strategies have been reviewed in the literature.[10][24][25]
History
The MOSFET (metal-oxide-semiconductor field-effect transistor, or MOS transistor) was invented by Mohamed M. Atalla and Dawon Kahng in 1959, and demonstrated in 1960.[26] Two years later, Leland C. Clark and Champ Lyons invented the first biosensor in 1962.[27][28] Biosensor MOSFETs (BioFETs) were later developed, and they have since been widely used to measure physical, chemical, biological and environmental parameters.[3]
The first BioFET was the ion-sensitive field-effect transistor (ISFET), invented by Piet Bergveld for electrochemical and biological applications in 1970.[29][30] Other early BioFETs include the adsorption FET (ADFET) patented by P.F. Cox in 1974, and a hydrogen-sensitive MOSFET demonstrated by I. Lundstrom, M.S. Shivaraman, C.S. Svenson and L. Lundkvist in 1975.[3] The ISFET is a special type of MOSFET with a gate at a certain distance,[3] and where the metal gate is replaced by an ion-sensitive membrane, electrolyte solution and reference electrode.[31] The ISFET is widely used in biomedical applications, such as the detection of DNA hybridization, biomarker detection from blood, antibody detection, glucose measurement, pH sensing, and genetic technology.[31]
By the mid-1980s, other BioFETs had been developed, including the gas sensor FET (GASFET), pressure sensor FET (PRESSFET), chemical field-effect transistor (ChemFET), reference ISFET (REFET), enzyme-modified FET (ENFET) and immunologically modified FET (IMFET).[3] By the early 2000s, BioFETs such as the DNA field-effect transistor (DNAFET), gene-modified FET (GenFET) and cell-potential BioFET (CPFET) had been developed.[31]
See also
References
- Maddalena, Francesco; Kuiper, Marjon J.; Poolman, Bert; Brouwer, Frank; Hummelen, Jan C.; de Leeuw, Dago M.; De Boer, Bert; Blom, Paul W. M. (2010). "Organic field-effect transistor-based biosensors functionalized with protein receptors" (PDF). Journal of Applied Physics. 108 (12): 124501. doi:10.1063/1.3518681. ISSN 0021-8979.
- Goldsmith, Brett R.; Locascio, Lauren; Gao, Yingning; Lerner, Mitchell; Walker, Amy; Lerner, Jeremy; Kyaw, Jayla; Shue, Angela; Afsahi, Savannah; Pan, Deng; Nokes, Jolie; Barron, Francie (2019). "Digital Biosensing by Foundry-Fabricated Graphene Sensors". Scientific Reports. 9 (1): 434. doi:10.1038/s41598-019-38700-w. ISSN 2045-2322. PMC 6342992. PMID 30670783.
- Bergveld, Piet (October 1985). "The impact of MOSFET-based sensors" (PDF). Sensors and Actuators. 8 (2): 109–127. doi:10.1016/0250-6874(85)87009-8. ISSN 0250-6874.
- Brand, U.; Brandes, L.; Koch, V.; Kullik, T.; Reinhardt, B.; Rüther, F.; Scheper, T.; Schügerl, K.; Wang, S.; Wu, X.; Ferretti, R.; Prasad, S.; Wilhelm, D. (1991). "Monitoring and control of biotechnological production processes by Bio-FET-FIA-sensors". Applied Microbiology and Biotechnology. 36 (2): 167–172. doi:10.1007/BF00164414. ISSN 0175-7598. PMID 1368106.
- Lin, M. C.; Chu, C. J.; Tsai, L. C.; Lin, H. Y.; Wu, C. S.; Wu, Y. P.; Wu, Y. N.; Shieh, D. B.; Su, Y. W. (2007). "Control and Detection of Organosilane Polarization on Nanowire Field-Effect Transistors". Nano Letters. 7 (12): 3656–3661. CiteSeerX 10.1.1.575.5601. doi:10.1021/nl0719170.
- Lee, Joonhyung; Dak, Piyush; Lee, Yeonsung; Park, Heekyeong; Choi, Woong; Alam, Muhammad A.; Kim, Sunkook (2014). "Two-dimensional Layered MoS2 Biosensors Enable Highly Sensitive Detection of Biomolecules". Scientific Reports. 4 (1): 7352. doi:10.1038/srep07352. ISSN 2045-2322. PMC 4268637. PMID 25516382.
- Schöning, Michael J.; Poghossian, Arshak (2002). "Recent advances in biologically sensitive field-effect transistors (BioFETs)" (PDF). The Analyst. 127 (9): 1137–1151. doi:10.1039/B204444G. ISSN 0003-2654. PMID 12375833.
- Alena Bulyha, Clemens Heitzinger and Norbert J Mauser: Bio-Sensors: Modelling and Simulation of Biologically Sensitive Field-Effect-Transistors, ERCIM News, 04,2011.
- Matsumoto, A; Miyahara, Y (21 November 2013). "Current and emerging challenges of field effect transistor based bio-sensing". Nanoscale. 5 (22): 10702–10718. doi:10.1039/c3nr02703a. PMID 24064964.
- Lowe, Benjamin M.; Sun, Kai; Zeimpekis, Ioannis; Skylaris, Chris-Kriton; Green, Nicolas G. (2017). "Field-effect sensors – from pH sensing to biosensing: sensitivity enhancement using streptavidin–biotin as a model system". The Analyst. 142 (22): 4173–4200. doi:10.1039/c7an00455a. ISSN 0003-2654. PMID 29072718.
- Yuji Miyahara, Toshiya Sakata, Akira Matsumoto: Microbio genetic analysis based on Field Effect Transistors, Principles of Bacterial Detection: Biosensors, Recognition Receptors and Microsystems.
- Poghossian, A.; Cherstvy, A.; Ingebrandt, S.; Offenhäusser, A.; Schöning, M.J. (2005). "Possibilities and limitations of label-free detection of DNA hybridization with field-effect-based devices". Sensors and Actuators B: Chemical. 111-112: 470–480. doi:10.1016/j.snb.2005.03.083. ISSN 0925-4005.
- K.Y.Park, M.S.Kim, K.M.Park, and S.Y.Choi: Fabrication of BioFET sensor for simultaneous detection of protein and DNA, Electrochem.org.
- Choi K, Kim JY, Ahn JH, Choi JM, Im M, Choi YK: Integration of field-effect transistor-based biosensors with a digital microfluidic device for a lab-on-a-chip application, Lab Chip., 2012 Apr
- Chu, Chia-Jung; Yeh, Chia-Sen; Liao, Chun-Kai; Tsai, Li-Chu; Huang, Chun-Ming; Lin, Hung-Yi; Shyue, Jing-Jong; Chen, Yit-Tsong; Chen, Chii-Dong (2013). "Improving Nanowire Sensing Capability by Electrical Field Alignment of Surface Probing Molecules". Nano Letters. 13 (6): 2564–2569. doi:10.1021/nl400645j. PMID 23634905.
- Jing-Juan Xu, Xi-Liang Luo and Hong-Yuan Chen: ANALYTICAL ASPECTS OF FET-BASED BIOSENSORS, Frontiers in Bioscience, 10, 420--430, January 1, 2005
- Sarkar, Deblina; Liu, Wei; Xie, Xuejun; Anselmo, Aaron C.; Mitragotri, Samir; Banerjee, Kaustav (2014). "MoS2Field-Effect Transistor for Next-Generation Label-Free Biosensors". ACS Nano. 8 (4): 3992–4003. doi:10.1021/nn5009148. ISSN 1936-0851. PMID 24588742.
- Wen, Xuejin; Gupta, Samit; Nicholson, Theodore R.; Lee, Stephen C.; Lu, Wu (2011). "AlGaN/GaN HFET biosensors working at subthreshold regime for sensitivity enhancement". Physica Status Solidi C. 8 (7–8): 2489–2491. doi:10.1002/pssc.201001174. ISSN 1862-6351.
- Sun, K; Zeimpekis, I; Hu, C; Ditshego, N M J; Thomas, O; de Planque, M R R; Chong, H M H; Morgan, H; Ashburn, P (2016). "Effect of subthreshold slope on the sensitivity of nanoribbon sensors" (PDF). Nanotechnology. 27 (28): 285501. doi:10.1088/0957-4484/27/28/285501. ISSN 0957-4484. PMID 27255984.
- Gao, Xuan P. A.; Zheng, Gengfeng; Lieber, Charles M. (2010). "Subthreshold Regime has the Optimal Sensitivity for Nanowire FET Biosensors". Nano Letters. 10 (2): 547–552. doi:10.1021/nl9034219. ISSN 1530-6984. PMC 2820132. PMID 19908823.
- Rajan, Nitin K.; Routenberg, David A.; Reed, Mark A. (2011). "Optimal signal-to-noise ratio for silicon nanowire biochemical sensors". Applied Physics Letters. 98 (26): 264107–264107–3. doi:10.1063/1.3608155. ISSN 0003-6951. PMC 3144966. PMID 21799538.
- Kim JY, Choi K, Moon DI, Ahn JH, Park TJ, Lee SY, Choi YK: Surface engineering for enhancement of sensitivity in an underlap-FET biosensor by control of wettability, Biosens Bioelectron., 2013
- A. Finn, J.Alderman, J. Schweizer : TOWARDS AN OPTIMIZATION OF FET-BASED BIO-SENSORS, European Cells and Materials, Vol. 4. Suppl. 2, 2002 (pages 21-23)
- Schöning, Michael J.; Poghossian, Arshak (2002). "Recent advances in biologically sensitive field-effect transistors (BioFETs)" (PDF). The Analyst. 127 (9): 1137–1151. doi:10.1039/b204444g. ISSN 0003-2654. PMID 12375833.
- Schöning, Michael J.; Poghossian, Arshak (2006). "Bio FEDs (Field-Effect Devices): State-of-the-Art and New Directions". Electroanalysis. 18 (19–20): 1893–1900. doi:10.1002/elan.200603609. ISSN 1040-0397.
- "1960: Metal Oxide Semiconductor (MOS) Transistor Demonstrated". The Silicon Engine: A Timeline of Semiconductors in Computers. Computer History Museum. Retrieved August 31, 2019.
- Park, Jeho; Nguyen, Hoang Hiep; Woubit, Abdela; Kim, Moonil (2014). "Applications of Field-Effect Transistor (FET)–Type Biosensors". Applied Science and Convergence Technology. 23 (2): 61–71. doi:10.5757/ASCT.2014.23.2.61. ISSN 2288-6559. S2CID 55557610.
- Clark, Leland C.; Lyons, Champ (1962). "Electrode Systems for Continuous Monitoring in Cardiovascular Surgery". Annals of the New York Academy of Sciences. 102 (1): 29–45. doi:10.1111/j.1749-6632.1962.tb13623.x. ISSN 1749-6632. PMID 14021529.
- Chris Toumazou; Pantelis Georgiou (December 2011). "40 years of ISFET technology:From neuronal sensing to DNA sequencing". Electronics Letters. Retrieved 13 May 2016.
- Bergveld, P. (January 1970). "Development of an Ion-Sensitive Solid-State Device for Neurophysiological Measurements". IEEE Transactions on Biomedical Engineering. BME-17 (1): 70–71. doi:10.1109/TBME.1970.4502688. PMID 5441220.
- Schöning, Michael J.; Poghossian, Arshak (10 September 2002). "Recent advances in biologically sensitive field-effect transistors (BioFETs)" (PDF). Analyst. 127 (9): 1137–1151. doi:10.1039/B204444G. ISSN 1364-5528. PMID 12375833.