Nonmetal
In chemistry, a nonmetal (or non-metal) is a chemical element that mostly lacks the characteristics of a metal. Physically, a nonmetal tends to have a relatively low melting point, boiling point, and density. A nonmetal is typically brittle when solid and usually has poor thermal conductivity and electrical conductivity. Chemically, nonmetals tend to have relatively high ionization energy, electron affinity, and electronegativity. They gain or share electrons when they react with other elements and chemical compounds. Seventeen elements are generally classified as nonmetals: most are gases (hydrogen, helium, nitrogen, oxygen, fluorine, neon, chlorine, argon, krypton, xenon and radon); one is a liquid (bromine); and a few are solids (carbon, phosphorus, sulfur, selenium, and iodine). Metalloids such as boron, silicon, and germanium are sometimes counted as nonmetals.
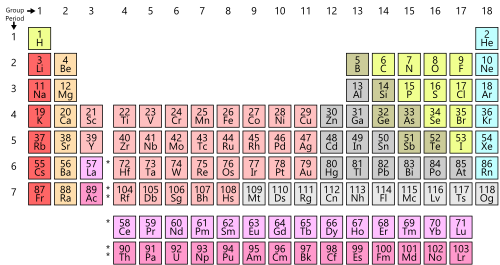
Metalloids are included in the legend as they generally behave chemically like nonmetals and are sometimes counted as such. | ||
Part of a series on the |
Periodic table |
---|
Periodic table forms |
By periodic table structure
|
|
By other characteristics
|
|
|
Data pages for elements
|
|
The nonmetals are divided into two categories reflecting their relative propensity to form chemical compounds: reactive nonmetals and noble gases. The reactive nonmetals vary in their nonmetallic character. The less electronegative of them, such as carbon and sulfur, mostly have weak to moderately strong nonmetallic properties and tend to form covalent compounds with metals. The more electronegative of the reactive nonmetals, such as oxygen and fluorine, are characterised by stronger nonmetallic properties and a tendency to form predominantly ionic compounds with metals. The noble gases are distinguished by their great reluctance to form compounds with other elements.
The distinction between categories is not absolute. Boundary overlaps, including with the metalloids, occur as outlying elements in each category show or begin to show less-distinct, hybrid-like, or atypical properties.
Although five times more elements are metals than nonmetals, two of the nonmetals—hydrogen and helium—make up over 99 percent of the observable universe.[1] Another nonmetal, oxygen, makes up almost half of the Earth's crust, oceans, and atmosphere.[2] Living organisms are composed almost entirely of nonmetals: hydrogen, oxygen, carbon, and nitrogen.[3] Nonmetals form many more compounds than metals.[4]
Definition and applicable elements
There is no rigorous definition of a nonmetal. Broadly, any element lacking a preponderance of metallic properties can be regarded as a nonmetal.
The elements generally classified as nonmetals include one element in group 1 (hydrogen); one in group 14 (carbon); two in group 15 (nitrogen and phosphorus); three in group 16 (oxygen, sulfur and selenium); most of group 17 (fluorine, chlorine, bromine and iodine); and all of group 18 (with the possible exception of oganesson).
As there is no widely agreed definition of a nonmetal, elements in the periodic table vicinity of where the metals meet the nonmetals are inconsistently classified by different authors. Elements sometimes also classified as nonmetals are the metalloids boron (B), silicon (Si), germanium (Ge), arsenic (As), antimony (Sb), tellurium (Te), and astatine (At).[5] The nonmetal selenium (Se) is sometimes instead classified as a metalloid, particularly in environmental chemistry.[6]
Properties
JJ Zuckerman and FC Nachod
In Steudel's Chemistry of the non-metals (1977, preface)
Nonmetals show more variability in their properties than do metals.[7] These properties are largely determined by the interatomic bonding strengths and molecular structures of the nonmetals involved, both of which are subject to variation as the number of valence electrons in each nonmetal varies. Metals, in contrast, have more homogenous structures and their properties are more easily reconciled.[8]
Physically, they largely exist as diatomic or monatomic gases, with the remainder having more substantial (open-packed) forms, unlike metals, which are nearly all solid and close-packed. If solid, they have a submetallic appearance (with the exception of sulfur) and are mostly brittle, as opposed to metals, which are lustrous, and generally ductile or malleable; they usually have lower densities than metals; are mostly poorer conductors of heat and electricity; and tend to have significantly lower melting points and boiling points than those of metals.
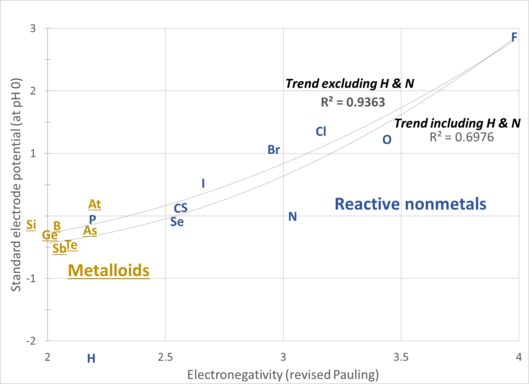
- Scatter plot of electronegativity values and standard electrode potentials of chemically active nonmetallic elements, showing a rough correlation between the two properties. The higher the standard electrode potential, the greater is the capacity to act as an oxidizing agent.[9] The chart shows that oxygen and the nonmetallic halogens are the strongest oxidising agents and that, for the most part, the elements commonly recognised as metalloids are the weakest. The electrode potentials are for the reduction of the elements to monatomic anions (X→X−; X = F, Cl, Br, I, or H) or to their protonated forms (for example, O2→H2O; N2→NH3.[10]
- Hydrogen and nitrogen have anomalous standard electrode potentials due to their reluctance to form anions.
- A broad progression in nonmetallic character is seen, with the metalloids in the lower left, and oxygen and the nonmetallic halogens in the upper right.
- Trend lines are shown with and without the anomalous hydrogen and nitrogen values. The R2 values show how close each trend line fits its data points. Values range from 0.0 (indicating no fit) and 1.0 (a very good fit).
Chemically the nonmetals mostly have high ionisation energies, high electron affinities (nitrogen and the noble gases have negative electron affinities) and high electronegativity values[n 1] noting that, in general, the higher an element's ionisation energy, electron affinity, and electronegativity, the more nonmetallic that element is.[11] Nonmetals (including – to a limited extent – xenon and probably radon) usually exist as anions or oxyanions in aqueous solution; they generally form ionic or covalent compounds when combined with metals (unlike metals, which mostly form alloys with other metals); and have acidic oxides whereas the common oxides of nearly all metals are basic.
Complicating the chemistry of the nonmetals is the first row anomaly seen particularly in hydrogen, (boron), carbon, nitrogen, oxygen and fluorine; and the alternation effect seen in (arsenic), selenium and bromine.[12] The first row anomaly largely arises from the electron configurations of the elements concerned.
Hydrogen is noted for the different ways it forms bonds. It most commonly forms covalent bonds.[13] It can lose its single valence electron in aqueous solution, leaving behind a bare proton with tremendous polarising power. This subsequently attaches itself to the lone electron pair of an oxygen atom in a water molecule, thereby forming the basis of acid-base chemistry.[14] Under certain conditions a hydrogen atom in a molecule can form a second, weaker, bond with an atom or group of atoms in another molecule. Such bonding, "helps give snowflakes their hexagonal symmetry, binds DNA into a double helix; shapes the three-dimensional forms of proteins; and even raises water's boiling point high enough to make a decent cup of tea."[15]
From (boron) to neon, since the 2p subshell has no inner analogue and experiences no electron repulsion effects it consequently has a relatively small radius, unlike the 3p, 4p and 5p subshells of heavier elements[16] (a similar effect is seen in the 1s elements, hydrogen and helium). Ionisation energies and electronegativities among these elements are consequently higher than would otherwise be expected, having regard to periodic trends. The small atomic radii of carbon, nitrogen, and oxygen facilitates the formation of triple or double bonds.[17] The larger atomic radii, which enable higher coordination numbers, and lower electronegativities, which better tolerate higher positive charges, of the heavier group 15–18 nonmetals means they are able to exhibit valences other than the lowest for their group (that is, 3, 2, 1, or 0) for example in PCl5, SF6, IF7, and XeF2.[18] Period four elements immediately after the first row of the transition metals, such as selenium and bromine, have unusually small atomic radii because the 3d electrons are not effective at shielding the increased nuclear charge, and smaller atomic size correlates with higher electronegativity.[19]
Categories
Immediately to the left of most nonmetals on the periodic table are metalloids such as boron, silicon, and germanium, which generally behave chemically like nonmetals,[20] and are included here for comparative purposes. In this sense they can be regarded as the most metallic of nonmetallic elements.
Based on shared attributes, the nonmetals can be divided into the two categories of reactive nonmetal, and noble gas. The metalloids and the two nonmetal categories then span a progression in chemical nature from weakly nonmetallic, to moderately nonmetallic, to strongly nonmetallic (oxygen and the four nonmetallic halogens), to almost inert. Analogous categories occur among the metals in the form of the weakly metallic (the post-transition metals), the moderately metallic (most of the transition metals), the strongly metallic (the alkali metal and alkaline earth metals, and the lanthanides and actinides), and the relatively inert (the noble transition metals).
As with categorisation schemes generally, there is some variation and overlapping of properties within and across each category. One or more of the metalloids are sometimes classified as nonmetals.[5] Among the reactive nonmetals, carbon, phosphorus, selenium, and iodine—which border the metalloids—show some metallic character, as does hydrogen. Among the noble gases, radon is the most metallic and begins to show some cationic behaviour, which is unusual for a nonmetal.[21]
Metalloid
The seven metalloids are boron (B), silicon (Si), germanium (Ge), arsenic (As), antimony (Sb), tellurium (Te), and astatine (At). On a standard periodic table, they occupy a diagonal area in the p-block extending from boron at the upper left to astatine at lower right, along the dividing line between metals and nonmetals shown on some periodic tables. They are called metalloids mainly in light of their physical resemblance to metals.
While they each have a metallic appearance, they are brittle and only fair conductors of electricity. Boron, silicon, germanium, tellurium are semiconductors. Arsenic and antimony have the electronic band structures of semimetals although both have less stable semiconducting allotropes. Astatine has been predicted to have a metallic crystalline structure.
Electronegativity values of metalloids and nonmetals[n 2] | |||||||||
---|---|---|---|---|---|---|---|---|---|
1 | 2 | 13 | 14 | 15 | 16 | 17 | 18 | ||
Noble gases |
|||||||||
1 | H 2.2 |
Reactive nonmetals |
He (5.5) |
||||||
2 | B 2.04 | C 2.55 | N 3.04 | O 3.44 | F 3.98 | Ne (4.84) |
|||
3 | Si 1.9 | P 2.19 | S 2.58 | Cl 3.16 | Ar (3.2) |
||||
4 | Ge 2.01 | As 2.18 | Se 2.55 | Br 2.96 | Kr (2.94) |
||||
5 | Sb 2.05 | Te 2.1 | I 2.66 | Xe (2.4) |
|||||
6 | Metalloids | Rn (2.06) |
|||||||
Electronegativity (EN) gives some indication of nonmetallic character. The metalloids have uniformly moderate values (1.8–2.2). Among the reactive nonmetals, hydrogen (2.2) and phosphorus (2.19) have moderate values but they each have higher ionisation energies than the metalloids, and are very rarely classed as such. Oxygen and the nonmetallic halogens have uniformly high EN values; nitrogen has a high EN but a marginally negative electron affinity that makes it a reluctant anion former.[n 3] The noble gases have some of the highest ENs but their complete valence shells and sizeably negative electron affinities render them chemically inert to a large degree. |
Chemically the metalloids generally behave like (weak) nonmetals. They have moderate ionisation energies, low to high electron affinities, moderate electronegativity values, are poor to moderately strong oxidising agents, and demonstrate a tendency to form alloys with metals.
Reactive nonmetal
The reactive nonmetals have a diverse range of individual physical and chemical properties. In periodic table terms they largely occupy a position between the weakly nonmetallic metalloids to the left and the noble gases to the right.
Physically, five are solids, one is a liquid (bromine), and five are gases. Of the solids, graphite carbon, selenium, and iodine are metallic-looking, whereas S8 sulfur has a pale-yellow appearance. Ordinary white phosphorus has a yellowish-white appearance but the black allotrope, which is the most stable form of phosphorus, has a metallic-looking appearance. Bromine is a reddish-brown liquid. Of the gases, fluorine and chlorine are coloured pale yellow, and yellowish green. Electrically, most are insulators whereas graphite is a semimetal and black phosphorus, selenium, and iodine are semiconductors.
Chemically, they tend to have moderate to high ionisation energies, electron affinities, and electronegativity values, and be relatively strong oxidising agents. Collectively, the highest values of these properties are found among oxygen and the nonmetallic halogens. Manifestations of this status include oxygen's major association with the ubiquitous processes of corrosion and combustion, and the intrinsically corrosive nature of the nonmetallic halogens. All five of these nonmetals exhibit a tendency to form predominately ionic compounds with metals whereas the remaining nonmetals tend to form predominately covalent compounds with metals.
Noble gas
Six nonmetals are categorised as noble gases: helium (He), neon (Ne), argon (Ar), krypton (Kr), xenon (Xe), and the radioactive radon (Rn). In periodic table terms they occupy the outermost right column. They are called noble gases in light of their characteristically very low chemical reactivity.
They have very similar properties, all being colorless, odorless, and nonflammable. With their closed valence shells the noble gases have feeble interatomic forces of attraction resulting in very low melting and boiling points.[22] That is why they are all gases under standard conditions, even those with atomic masses larger than many normally solid elements.[23]
Chemically, the noble gases have relatively high ionization energies, negative electron affinities, and relatively high electronegativities. Compounds of the noble gases number less than half a thousand, with most of these occurring via oxygen or fluorine combining with either krypton, xenon or radon.
The status of the period 7 congener of the noble gases, oganesson (Og), is not known—it may or may not be a noble gas. It was originally predicted to be a noble gas[24] but may instead be a fairly reactive solid with an anomalously low first ionisation potential, and a positive electron affinity, due to relativistic effects.[25] On the other hand, if relativistic effects peak in period 7 at element 112, copernicium, oganesson may turn out to be a noble gas after all,[26] albeit more reactive than either xenon or radon. While oganesson could be expected to be the most metallic of the group 18 elements, credible predictions on its status as either a metal or a nonmetal (or a metalloid) appear to be absent.
Alternative categories
Nonmetal categorisation and alternative schemes | |||||
---|---|---|---|---|---|
Reactive nonmetal | Noble gas | ||||
H, C, N, P, O, S, Se, F, Cl, Br, I | He, Ne, Ar, Kr, Xe, Rn | ||||
(1) | Other nonmetal | Halogen | Noble gas | ||
H, C, N, P, O, S, (Se) | F, Cl, Br, I, At | He, Ne, Ar, Kr, Xe, Rn | |||
(2) | Solid | Liquid | Gaseous | ||
C, P, S, Se, I, At | Br | H, N, O, F, Cl, He, Ne, Ar, Kr, Xe, Rn | |||
(3) | Electronegative nonmetal |
Very electronegative nonmetal |
Noble gas | ||
H, C, P, S, Se, I | N, O, F, Cl, Br | He, Ne, Ar, Kr, Xe, Rn | |||
(4) | Polyatomic element |
Diatomic element | Monatomic element (noble gas) | ||
C, P, S, Se | H, N, O, F, Cl, Br, I | He, Ne, Ar, Kr, Xe, Rn | |||
(5) | Hydrogen | Nonmetal | Halogen | Noble gas | |
H | C, N, P, O, S, Se | F, Cl, Br, I, At | He, Ne, Ar, Kr, Xe, Rn | ||
(6) | Hydrogen | Carbon and other nonmetals | Halogen | Noble gas | |
H | C, N, P, O, S, Se | F, Cl, Br, I, At | He, Ne, Ar, Kr, Xe, Rn | ||
(7) | Metalloid | Intermediate nonmetal |
Corrosive nonmetal |
Noble gas | |
B, Si, Ge, As, Sb, Te | H, C, N, P, S, Se | O, F, Cl, Br, I, At | He, Ne, Ar, Kr, Xe, Rn | ||
(8) | Hydrogen | Metalloid | Nonmetal | Halogen | Noble gas |
H | B, Si, Ge, As, Sb, Te, Po | C, N, P, O, S, Se | F, Cl, Br, I, At | He, Ne, Ar, Kr, Xe, Rn | |
(9) | Hydrogen | Semiconductor | Other nonmetal | Halogen | Noble gas |
H | B, Si, Ge, As, Sb, Te | C, N, P, O, S, Se | F, Cl, Br, I, At | He, Ne, Ar, Kr, Xe, Rn |
The nonmetals are sometimes instead divided according to either (1) the relative homogeneity of the halogens; (2) physical form; (3) electronegativity; (4) molecular structure; (5) the peculiar nature of hydrogen, and the relative homogeneity of the halogens; (6) their analogous categories among the metals; or (7) the uniqueness of hydrogen and the treatment of the metalloids as nonmetallic analogues of the post-transition metals.
In scheme (1), the halogens are in a category of their own; astatine is classed as a nonmetal, rather than a metalloid; and the remaining nonmetals are referred to as other nonmetals.[27] If selenium is counted as a metalloid rather than another nonmetal, the resulting set of less active nonmetals (H, C, N, P, O, S) are sometimes instead referred to or categorised as organogens,[28] CHONPS elements[29] or biogens.[30] Collectively these six nonmetals comprise the bulk of life on Earth;[31] a rough estimate of the composition of the biosphere is C1450H3000O1450N15P1S1.[32]
In scheme (2), the nonmetals can simply be divided based on their physical forms at room temperature and pressure. The fluid nonmetals (bromine and the gaseous nonmetals) have the highest ionisation energy and electronegativity values among the elements, with the exception of hydrogen which tends to be anomalous in whichever category it is placed in. The solid nonmetals are collectively the most metallic of the nonmetallic elements, apart from the metalloids.
In scheme (3), the nonmetals are divided based on a loose correlation between electronegativity and oxidizing power.[33] Very electronegative nonmetals have electronegativity values over 2.8; electronegative nonmetals have values of 1.9 to 2.8.
In scheme (4), the nonmetals are distinguished based on the molecular structures of their most thermodynamically stable forms in ambient conditions.[34] Polyatomic nonmetals form structures or molecules in which each atom has two or three nearest neighbours (Cx, P4, S8, Sex); diatomic nonmetals form molecules in which each atom has one nearest neighbour (H2, N2, O2, F2, Cl2, Br2, I2); and the monatomic noble gases exist as isolated atoms (He, Ne, Ar, Kr, Xe, Rn) with no fixed nearest neighbour. This gradual reduction in the number of nearest neighbours corresponds (approximately) to a reduction in metallic character. A similar progression is seem among the metals. Metallic bonding tends to involve close-packed centrosymmetric structures with a high number of nearest neighbours. Post-transition metals and metalloids, sandwiched between the true metals and the nonmetals, tend to have more complex structures with an intermediate number of nearest neighbours.
In scheme (5), hydrogen is placed by itself on account of it being "so different from all other elements".[35] The remaining nonmetals are divided into nonmetals, halogens, and noble gases, with the unnamed category being distinguished by including nonmetals with relatively strong interatomic bonding, and the metalloids being effectively treated as a third super-category alongside metals and nonmetals.
He, Ne, Ar, Kr, Xe, Rn | |||||
Active metals Groups 1–3, Ln, An, (Al) | Corrosive nonmetals O, F, Cl, Br, I | ||||
Transition metals Most of them | Intermediate nonmetals H, C, N, P, S, Se | ||||
Frontier metals (Al) Ag, Sn, Bi etc. | Metalloids B, Si, Ge, As, Sb, Te | ||||
Ru, Rh, Pd, Os, Ir, Pt, Au |
Scheme (6) is a variant of scheme 5 in which the C, N, O, P, S, Se are categorised as carbon and other nonmetals (no emphasis).[36]
In scheme (7) the nonmetals are divided into four classes that complement a four-fold division of the metals, with the noble metals treated as a subset of the transition metals. The metalloids are treated as chemically weak nonmetals, in a manner analogous to their chemically weak frontier metal counterparts.[37]
In scheme (8), hydrogen is again placed by itself on account of its uniqueness. The remaining nonmetals are divided into metalloids, nonmetals, (referred to as "quintessential nonmetals"), halogens, and noble gases. Since the metalloids abut the post-transition or "poor" metals, they might be renamed the "poor non-metals".[38]
Scheme (9) is a variant of scheme 8 in which the metalloids are labeled as semiconductors (with Po regarded as a post-transition metal) and C, N, O, P, S, Se categorised as other nonmetals.[39]
Comparison of properties
Characteristic and other properties of metalloids, reactive nonmetals, and noble gases are summarized in the following table. Metalloids have been included in light of their generally nonmetallic chemistry. Physical properties are listed in loose order of ease of determination; chemical properties run from general to specific, and then to descriptive.
Physical property | Metalloid | Reactive nonmetal | Noble gas |
---|---|---|---|
Form | solid | solid: C, P, S, Se, I liquid: Br gaseous: H, N, O, F, Cl |
gaseous |
Appearance | metallic | metallic, coloured, or translucent | translucent |
Elasticity | brittle | brittle if solid | soft and easily crushed when frozen |
Atomic structure | close-packed* or polyatomic | polyatomic: C, P, S, Se diatomic: H, N, O, F, Cl, Br, I |
monatomic |
Bulk coordination number | 12*, 6, 4, 3, or 2 | 3, 2, or 1 | 0 |
Allotropes | most form | known for C, P, O, S, Se | none form |
Electrical conductivity | moderate | poor to moderate | poor |
Volatility | low: B, Si, Ge, Sb, Te moderate: As, At? |
low: C moderate: P, S, Se, Br, I high: H, N, O, F, Cl |
high |
Electronic structure | metallic* to semiconductor | semimetallic, semiconductor, or insulator | insulator |
Outer s and p electrons | 3–7 | 1, 4–7 | 2, 8 |
Crystal structure | rhombohedral: B, As, Sb cubic: Si, Ge, At? hexagonal: Te |
cubic: P, O, F hexagonal: H, C, N, Se orthorhombic: S, Cl, Br, I |
cubic: Ne, Ar, Kr, Xe, Rn hexagonal: He |
Chemical property | Metalloid | Reactive nonmetal | Noble gas |
General chemical behaviour | nonmetallic to incipient metallic | • inert to nonmetallic • Rn shows some cationic behaviour[40] | |
Ionization energy | low | moderate to high | high to very high |
Electron affinity | low to high | moderate to high (exception: N is negative) | negative |
Electronegativity | moderate: Si < Ge ≈ B ≈ Sb < Te <As ≈ At |
moderate to high: P < Se ≈ C < S < I < Br < N < Cl < O < F |
moderate to very high |
Non-zero oxidation states | • negative oxidation states known for all, but for H this is an unstable state • positive oxidation states known for all but F, and only exceptionally for O • from −5 for B to +7 for Cl, Br, I, and At |
• only positive oxidation states known, and only for heavier noble gases • from +2 for Kr, Xe, and Rn to +8 for Xe | |
Oxidising power | low (exception: At is moderate) |
low to high | n/a |
Catenation | marked tendency | marked tendency: C, P, S, Se less tendency: H, N, O, F, Cl, Br, I |
least inclination |
Compounds with metals | tend to form alloys or inter-metallic compounds | mainly covalent: H†, C, N, P, S, Se mainly ionic: O, F, Cl, Br, I |
none form simple compounds |
Oxides | • polymeric in structure[41] • B, Si, Ge, As, Sb, Te[42] are glass formers • tend to be amphoteric or weakly acidic[43][44] |
• C, P, S, Se, and I are known in at least one polymeric form • P, S, Se are glass formers; CO2 forms a glass at 40 GPa • acidic, or neutral (H2O, CO, NO, N2O) |
• XeO2 is polymeric;[45] other noble gas oxides are molecular • no glass formers • stable xenon oxides (XeO3, XeO4) are acidic |
Sulfates | most form | some form | not known |
* Bulk astatine has been predicted to have a metallic face-centred cubic structure
† Hydrogen can also form alloy-like hydrides
Properties of nonmetals (and metalloids) by group
- Abbreviations used in this section are: AR Allred-Rochow; CN coordination number; and MH Moh's hardness
Group 1
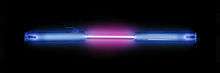
Hydrogen is a colourless, odourless, and comparatively unreactive diatomic gas with a density of 8.988 × 10−5 g/cm3 and is about 14 times lighter than air. It condenses to a colourless liquid −252.879 °C and freezes into an ice- or snow-like solid at −259.16 °C. The solid form has a hexagonal crystalline structure and is soft and easily crushed. Hydrogen is an insulator in all of its forms. It has a high ionisation energy (1312.0 kJ/mol), moderate electron affinity (73 kJ/mol), and moderate electronegativity (2.2). Hydrogen is a poor oxidising agent (H2 + 2e− → 2H– = –2.25 V at pH 0). Its chemistry, most of which is based around its tendency to acquire the electron configuration of the noble gas helium, is largely covalent in nature, noting it can form ionic hydrides with highly electropositive metals, and alloy-like hydrides with some transition metals. The common oxide of hydrogen (H2O) is a neutral oxide.[n 4]
Group 13
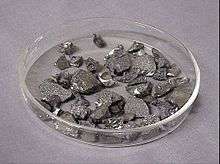
Boron is a lustrous, barely reactive solid with a density 2.34 g/cm3 (cf. aluminium 2.70), and is hard (MH 9.3) and brittle. It melts at 2076 °C (cf. steel ~1370 °C) and boils at 3927 °C. Boron has a complex rhombohedral crystalline structure (CN 5+). It is a semiconductor with a band gap of about 1.56 eV. Boron has a moderate ionisation energy (800.6 kJ/mol), low electron affinity (27 kJ/mol), and moderate electronegativity (2.04). Being a metalloid, most of its chemistry is nonmetallic in nature. Boron is a poor oxidizing agent (B12 + 3e → BH3 = –0.15 V at pH 0). While it bonds covalently in nearly all of its compounds, it can form intermetallic compounds and alloys with transition metals of the composition MnB, if n > 2. The common oxide of boron (B2O3) is weakly acidic.
Group 14
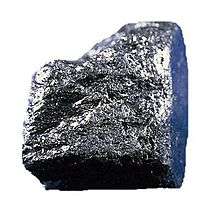
Carbon (as graphite, its most thermodynamically stable form) is a lustrous and comparatively unreactive solid with a density of 2.267 g/cm3, and is soft (MH 0.5) and brittle. It sublimes to vapour at 3642 C°. Carbon has a hexagonal crystalline structure (CN 3). It is a semimetal in the direction of its planes, with an electrical conductivity exceeding that of some metals, and behaves as a semiconductor in the direction perpendicular to its planes. It has a high ionisation energy (1086.5 kJ/mol), moderate electron affinity (122 kJ/mol), and high electronegativity (2.55). Carbon is a poor oxidising agent (C + 4e− → CH4 = 0.13 V at pH 0). Its chemistry is largely covalent in nature, noting it can form salt-like carbides with highly electropositive metals. The common oxide of carbon (CO2) is a medium-strength acidic oxide.
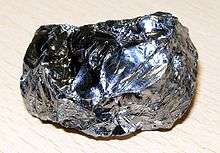
Silicon is a metallic-looking relatively unreactive solid with a density of 2.3290 g/cm3, and is hard (MH 6.5) and brittle. It melts at 1414 °C (cf. steel ~1370 °C) and boils at 3265 °C. Silicon has a diamond cubic structure (CN 4). It is a semiconductor with a band gap of about 1.11 eV. Silicon has a moderate ionisation energy (786.5 kJ/mol), moderate electron affinity (134 kJ/mol), and moderate electronegativity (1.9). It is a poor oxidising agent (Si + 4e → Si4 = –0.147 at pH 0). As a metalloid the chemistry of silicon is largely covalent in nature, noting it can form alloys with metals such as iron and copper. The common oxide of silicon (SiO2) is weakly acidic.
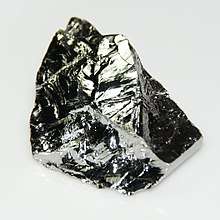
Germanium is a shiny, mostly unreactive grey-white solid with a density of 5.323 g/cm3 (about two-thirds that of iron), and is hard (MH 6.0) and brittle. It melts at 938.25 °C (cf. silver 961.78 °C) and boils at 2833 °C. Germanium has a diamond cubic structure (CN 4). It is a semiconductor with a band gap of about 0.67 eV. Germanium has a moderate ionisation energy (762 kJ/mol), moderate electron affinity (119 kJ/mol), and moderate electronegativity (2.01). It is a poor oxidising agent (Ge + 4e → GeH4 = –0.294 at pH 0). As a metalloid the chemistry of germanium is largely covalent in nature, noting it can form alloys with metals such as aluminium and gold. Most alloys of germanium with metals lack metallic or semimetallic conductivity. The common oxide of germanium (GeO2) is amphoteric.
Group 15
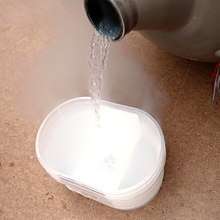
Nitrogen is a colourless, odourless, and relatively inert diatomic gas with a density of 1.251 × 10−3 g/cm3 (marginally heavier than air). It condenses to a colourless liquid at −195.795 °C and freezes into an ice- or snow-like solid at −210.00 °C. The solid form (density 0.85 g/cm3; cf. lithium 0.534) has a hexagonal crystalline structure and is soft and easily crushed. Nitrogen is an insulator in all of its forms. It has a high ionisation energy (1402.3 kJ/mol), low electron affinity (–6.75 kJ/mol), and high electronegativity (3.04). The latter property manifests in the capacity of nitrogen to form usually strong hydrogen bonds, and its preference for forming complexes with metals having low electronegativities, small cationic radii, and often high charges (+3 or more). Nitrogen is a poor oxidising agent (N2 + 6e− → 2NH3 = −0.057 V at pH 0). Only when it is in a positive oxidation state, that is, in combination with oxygen or fluorine, are its compounds good oxidising agents, for example, 2NO3− → N2 = 1.25 V. Its chemistry is largely covalent in nature; anion formation is energetically unfavourable owing to strong inter electron repulsions associated with having three unpaired electrons in its outer valence shell, hence its negative electron affinity. The common oxide of nitrogen (NO) is weakly acidic. Many compounds of nitrogen are less stable than diatomic nitrogen, so nitrogen atoms in compounds seek to recombine if possible and release energy and nitrogen gas in the process, which can be leveraged for explosive purposes.
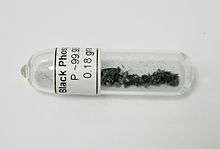
Phosphorus in its most thermodynamically stable black form, is a lustrous and comparatively unreactive solid with a density of 2.69 g/cm3, and is soft (MH 2.0) and has a flaky comportment. It sublimes at 620 °C. Black phosphorus has an orthorhombic crystalline structure (CN 3). It is a semiconductor with a band gap of 0.3 eV. It has a high ionisation energy (1086.5 kJ/mol), moderate electron affinity (72 kJ/mol), and moderate electronegativity (2.19). In comparison to nitrogen, phosphorus usually forms weak hydrogen bonds, and prefers to form complexes with metals having high electronegativities, large cationic radii, and often low charges (usually +1 or +2. Phosphorus is a poor oxidising agent (P4 + 3e− → PH3– = −0.046 V at pH 0 for the white form, −0.088 V for the red). Its chemistry is largely covalent in nature, noting it can form salt-like phosphides with highly electropositive metals. Compared to nitrogen, electrons have more space on phosphorus, which lowers their mutual repulsion and results in anion formation requiring less energy. The common oxide of phosphorus (P2O5) is a medium-strength acidic oxide.
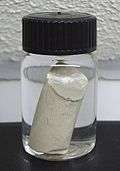
When assessing periodicity in the properties of the elements it needs to be borne in mind that the quoted properties of phosphorus tend to be those of its least stable white form rather than, as is the case with all other elements, the most stable form. White phosphorus is the most common, industrially important, and easily reproducible allotrope. For those reasons it is the standard state of the element. Paradoxically, it is also thermodynamically the least stable, as well as the most volatile and reactive form. It gradually changes to red phosphorus. This transformation is accelerated by light and heat, and samples of white phosphorus almost always contain some red phosphorus and, accordingly, appear yellow. For this reason, white phosphorus that is aged or otherwise impure is also called yellow phosphorus. When exposed to oxygen, white phosphorus glows in the dark with a very faint tinge of green and blue. It is highly flammable and pyrophoric (self-igniting) upon contact with air. White phosphorus has a density of 1.823 g/cm3, is soft (MH 0.5) as wax, pliable and can be cut with a knife. It melts at 44.15 °C and, if heated rapidly, boils at 280.5 °C; it otherwise remains solid and transforms to violet phosphorus at 550 °C. It has a body-centred cubic structure, analogous to that of manganese, with unit cell comprising 58 P4 molecules. It is an insulator with a band gap of about 3.7 eV.
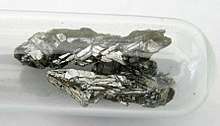
Arsenic is a grey, metallic looking solid which is stable in dry air but develops a golden bronze patina in moist air, which blackens on further exposure. It has a density of 5.727 g/cm3, and is brittle and moderately hard (MH 3.5; more than aluminium; less than iron). Arsenic sublimes at 615 °C. It has a rhombohedral polyatomic crystalline structure (CN 3). Arsenic is a semimetal, with an electrical conductivity of around 3.9 × 104 S•cm−1 and a band overlap of 0.5 eV. It has a moderate ionisation energy (947 kJ/mol), moderate electron affinity (79 kJ/mol), and moderate electronegativity (2.18). Arsenic is a poor oxidising agent (As + 3e → AsH3 = –0.22 at pH 0). As a metalloid, its chemistry is largely covalent in nature, noting it can form brittle alloys with metals, and has an extensive organometallic chemistry. Most alloys of arsenic with metals lack metallic or semimetallic conductivity. The common oxide of arsenic (As2O3) is acidic but weakly amphoteric.
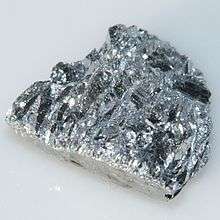
Antimony is a silver-white solid with a blue tint and a brilliant lustre. It is stable in air and moisture at room temperature. Antimony has a density of 6.697 g/cm3, and is moderately hard (MH 3.0; about the same as copper). It has a rhombohedral crystalline structure (CN 3). Antimony melts at 630.63 °C and boils at 1635 °C. It is a semimetal, with an electrical conductivity of around 3.1 × 104 S•cm−1 and a band overlap of 0.16 eV. Antimony has a moderate ionisation energy (834 kJ/mol), moderate electron affinity (101 kJ/mol), and moderate electronegativity (2.05). It is a poor oxidising agent (Sb + 3e → SbH3 = –0.51 at pH 0). As a metalloid, its chemistry is largely covalent in nature, noting it can form alloys with one or more metals such as aluminium, iron, nickel, copper, zinc, tin, lead and bismuth, and has an extensive organometallic chemistry. Most alloys of antimony with metals have metallic or semimetallic conductivity. The common oxide of antimony (Sb2O3) is amphoteric.
Group 16
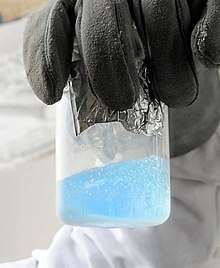
MD Joesten, L Hogg, and ME Castellion
In The world of chemistry (2007, p. 217)
Oxygen is a colourless, odourless, and unpredictably reactive diatomic gas with a gaseous density of 1.429 × 10−3 g/cm3 (marginally heavier than air). It is generally unreactive at room temperature. Thus, sodium metal will "retain its metallic lustre for days in the presence of absolutely dry air and can even be melted (m.p. 97.82 °C) in the presence of dry oxygen without igniting".[47] On the other hand, oxygen can react with many inorganic and organic compounds either spontaneously or under the right conditions,[48] (such as a flame or a spark) [or ultra-violet light?]. It condenses to pale blue liquid −182.962 °C and freezes into a light blue solid at −218.79 °C. The solid form (density 0.0763 g/cm3) has a cubic crystalline structure and is soft and easily crushed. Oxygen is an insulator in all of its forms. It has a high ionisation energy (1313.9 kJ/mol), high electron affinity (141 kJ/mol), and high electronegativity (3.44). Oxygen is a strong oxidising agent (O2 + 4e → 2H2O = 1.23 V at pH 0). Metal oxides are largely ionic in nature.[49]
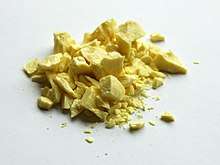
Sulfur is a bright-yellow moderately reactive[50] solid. It has a density of 2.07 g/cm3 and is soft (MH 2.0) and brittle. It melts to a light yellow liquid 95.3 °C and boils at 444.6 °C. Sulfur has an abundance on earth one-tenth that of oxygen. It has an orthorhombic polyatomic (CN 2) crystalline structure, and is brittle. Sulfur is an insulator with a band gap of 2.6 eV, and a photoconductor meaning its electrical conductivity increases a million-fold when illuminated. Sulfur has a moderate ionisation energy (999.6 kJ/mol), moderate electron affinity (200 kJ/mol), and high electronegativity (2.58). It is a poor oxidising agent (S8 + 2e− → H2S = 0.14 V at pH 0). The chemistry of sulfur is largely covalent in nature, noting it can form ionic sulfides with highly electropositive metals. The common oxide of sulfur (SO3) is strongly acidic.
.jpg)
Selenium is a metallic-looking, moderately reactive[50] solid with a density of 4.81 g/cm3 and is soft (MH 2.0) and brittle. It melts at 221 °C to a black liquid and boils at 685 °C to a dark yellow vapour. Selenium has a hexagonal polyatomic (CN 2) crystalline structure. It is a semiconductor with a band gap of 1.7 eV, and a photoconductor meaning its electrical conductivity increases a million-fold when illuminated. Selenium has a moderate ionisation energy (941.0 kJ/mol), high electron affinity (195 kJ/mol), and high electronegativity (2.55). It is a poor oxidising agent (Se + 2e− → H2Se = −0.082 V at pH 0). The chemistry of selenium is largely covalent in nature, noting it can form ionic selenides with highly electropositive metals. The common oxide of selenium (SeO3) is strongly acidic.
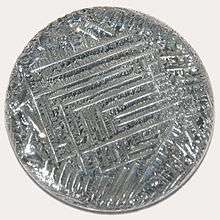
Tellurium is a silvery-white, moderately reactive,[50] shiny solid, that has a density of 6.24 g/cm3 and is soft (MH 2.25) and brittle. It is the softest of the commonly recognised metalloids. Tellurium reacts with boiling water, or when freshly precipitated even at 50 °C, to give the dioxide and hydrogen: Te + 2 H2O → TeO2 + 2 H2. It has a melting point of 450 °C and a boiling point of 988 °C. Tellurium has a polyatomic (CN 2) hexagonal crystalline structure. It is a semiconductor with a band gap of 0.32 to 0.38 eV. Tellurium has a moderate ionisation energy (869.3 kJ/mol), high electron affinity (190 kJ/mol), and moderate electronegativity (2.1). It is a poor oxidising agent (Te + 2e− → H2Te = −0.45 V at pH 0). The chemistry of tellurium is largely covalent in nature, noting it has an extensive organometallic chemistry and that many tellurides can be regarded as metallic alloys. The common oxide of tellurium (TeO2) is amphoteric.
Group 17
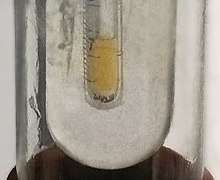
Fluorine is an extremely toxic and reactive pale yellow diatomic gas that, with a gaseous density of 1.696 × 10−3 g/cm3, is about 40% heavier than air. Its extreme reactivity is such that it was not isolated (via electrolysis) until 1886 and was not isolated chemically until 1986. Its occurrence in an uncombined state in nature was first reported in 2012, but is contentious. Fluorine condenses to a pale yellow liquid at −188.11 °C and freezes into a colourless solid[47] at −219.67 °C. The solid form (density 1.7 g/cm−3) has a cubic crystalline structure and is soft and easily crushed. Fluorine is an insulator in all of its forms. It has a high ionisation energy (1681 kJ/mol), high electron affinity (328 kJ/mol), and high electronegativity (3.98). Fluorine is a powerful oxidising agent (F2 + 2e → 2HF = 2.87 V at pH 0); "even water, in the form of steam, will catch fire in an atmosphere of fluorine".[51] Metal fluorides are generally ionic in nature.
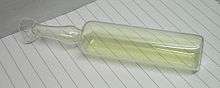
Chlorine is an irritating green-yellow diatomic gas that is extremely reactive, and has a gaseous density of 3.2 × 10−3 g/cm3 (about 2.5 times heavier than air). It condenses at −34.04 °C to an amber-coloured liquid and freezes at −101.5 °C into a yellow crystalline solid. The solid form (density 1.9 g/cm−3) has an orthorhombic crystalline structure and is soft and easily crushed. Chlorine is an insulator in all of its forms. It has a high ionisation energy (1251.2 kJ/mol), high electron affinity (349 kJ/mol; higher than fluorine), and high electronegativity (3.16). Chlorine is a strong oxidising agent (Cl2 + 2e → 2HCl = 1.36 V at pH 0). Metal chlorides are largely ionic in nature. The common oxide of chlorine (Cl2O7) is strongly acidic.
.png)
Bromine is a deep brown diatomic liquid that is quite reactive, and has a liquid density of 3.1028 g/cm3. It boils at 58.8 °C and solidifies at −7.3 °C to an orange crystalline solid (density 4.05 g/cm−3). It is the only element, apart from mercury, known to be a liquid at room temperature. The solid form, like chlorine, has an orthorhombic crystalline structure and is soft and easily crushed. Bromine is an insulator in all of its forms. It has a high ionisation energy (1139.9 kJ/mol), high electron affinity (324 kJ/mol), and high electronegativity (2.96). Bromine is a strong oxidising agent (Br2 + 2e → 2HBr = 1.07 V at pH 0). Metal bromides are largely ionic in nature. The unstable common oxide of bromine (Br2O5) is strongly acidic.
Iodine, the rarest of the nonmetallic halogens, is a metallic looking solid that is moderately reactive, and has a density of 4.933 g/cm3. It melts at 113.7 °C to a brown liquid and boils at 184.3 °C to a violet-coloured vapour. It has an orthorhombic crystalline structure with a flaky habit. Iodine is semiconductor in the direction of its planes, with a band gap of about 1.3 eV and a conductivity of 1.7 × 10−8 S•cm−1 at room temperature. This is higher than selenium but lower than boron, the least electrically conducting of the recognised metalloids. Iodine is an insulator in the direction perpendicular to its planes. It has a high ionisation energy (1008.4 kJ/mol), high electron affinity (295 kJ/mol), and high electronegativity (2.66). Iodine is a moderately strong oxidising agent (I2 + 2e → 2I− = 0.53 V at pH 0). Metal iodides are predominantly ionic in nature. The only stable oxide of iodine (I2O5) is strongly acidic.
Group 18
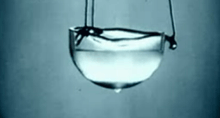
Helium has a density of 1.785 × 10−4 g/cm3 (cf. air 1.225 × 10−3 g/cm3), liquifies at −268.928 °C, and cannot be solidified at normal pressure. It has the lowest boiling point of all of the elements. Liquid helium exhibits super-fluidity, superconductivity, and near-zero viscosity; its thermal conductivity is greater than that of any other known substance (more than 1,000 times that of copper). Helium can only be solidified at −272.20 °C under a pressure of 2.5 MPa. It has a very high ionisation energy (2372.3 kJ/mol), low electron affinity (estimated at −50 kJ/mol), and very high electronegativity (5.5 AR). No normal compounds of helium have so far been synthesised.
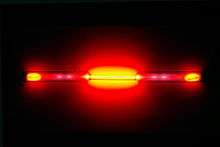
Neon has a density of 9.002 × 10−4 g/cm3, liquifies at −245.95 °C, and solidifies at −248.45 °C. It has the narrowest liquid range of any element and, in liquid form, has over 40 times the refrigerating capacity of liquid helium and three times that of liquid hydrogen. Neon has a very high ionisation energy (2080.7 kJ/mol), low electron affinity (estimated at −120 kJ/mol), and very high electronegativity (4.84 AR). It is the least reactive of the noble gases; no normal compounds of neon have so far been synthesised.
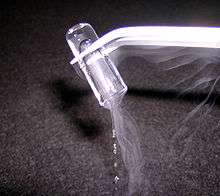
Argon has a density of 1.784 × 10−3 g/cm3, liquifies at −185.848 °C, and solidifies at −189.34 °C. Although non-toxic, it is 38% denser than air and therefore considered a dangerous asphyxiant in closed areas. It is difficult to detect because (like all the noble gases) it is colourless, odourless, and tasteless. Argon has a high ionisation energy (1520.6 kJ/mol), low electron affinity (estimated at −96 kJ/mol), and high electronegativity (3.2 AR). One interstitial compound of argon, Ar1C60 is a stable solid at room temperature.
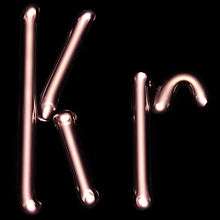
Krypton has a density of 3.749 × 10−3 g/cm3, liquifies at −153.415 °C, and solidifies at −157.37 °C. It has a high ionisation energy (1350.8 kJ/mol), low electron affinity (estimated at −60 kJ/mol), and high electronegativity (2.94 AR). Krypton can be reacted with fluorine to form the difluoride, KrF2. The reaction of KrF
2 with B(OTeF
5)
3 produces an unstable compound, Kr(OTeF
5)
2, that contains a krypton-oxygen bond.
Xenon has a density of 5.894 × 10−3 g/cm3, liquifies at −161.4 °C, and solidifies at −165.051 °C. It is non-toxic, and belongs to a select group of substances that penetrate the blood–brain barrier, causing mild to full surgical anesthesia when inhaled in high concentrations with oxygen. Xenon has a high ionisation energy (1170.4 kJ/mol), low electron affinity (estimated at −80 kJ/mol), and high electronegativity (2.4 AR). It forms a relatively large number of compounds, mostly containing fluorine or oxygen. An unusual ion containing xenon is the tetraxenonogold(II) cation, AuXe2+
4, which contains Xe–Au bonds. This ion occurs in the compound AuXe
4(Sb
2F
11)
2, and is remarkable in having direct chemical bonds between two notoriously unreactive atoms, xenon and gold, with xenon acting as a transition metal ligand. The compound Xe
2Sb
2F
11 contains a Xe–Xe bond, the longest element-element bond known (308.71 pm = 3.0871 Å). The most common oxide of xenon (XeO3) is strongly acidic.
Radon, which is radioactive, has a density of 9.73 × 10−3 g/cm3, liquifies at −61.7 °C, and solidifies at −71 °C. It has a high ionisation energy (1037 kJ/mol), low electron affinity (estimated at −70 kJ/mol), and moderate electronegativity (2.06 AR). The only confirmed compounds of radon, which is the rarest of the naturally occurring noble gases, are the difluoride RnF2, and trioxide, RnO3. It has been reported that radon is capable of forming a simple Rn2+ cation in halogen fluoride solution, which is highly unusual behaviour for a nonmetal, and a noble gas at that. Radon trioxide (RnO3) is expected to be acidic.
Oganesson, the heaviest element on the periodic table, has only recently been synthesized. Owing to its short half-life, its chemical properties have not yet been investigated. Due to the significant relativistic destabilisation of the 7p3/2 orbitals, it is expected to be significantly reactive and behave more similarly to the group 14 elements, as it effectively has four valence electrons outside a pseudo-noble gas core. Its boiling point is expected to be about 80±30 °C, so that it is probably neither noble nor a gas; as a liquid it is expected to have a density of about 5 g/cm3. It is expected to have a barely positive electron affinity (estimated as 5 kJ/mol) and a moderate ionisation energy of about 860 kJ/mol, which is rather low for a nonmetal and close to those of the metalloids tellurium and astatine. The oganesson fluorides OgF2 and OgF4 are expected to show significant ionic character, suggesting that oganesson may have at least incipient metallic properties. The oxides of oganesson, OgO and OgO2, are predicted to be amphoteric.
Cross-cutting relationships
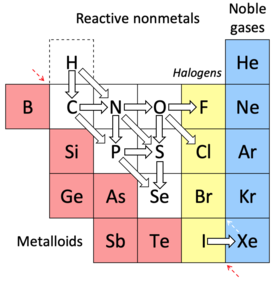
Some pairs of nonmetals show additional relationships, beyond those associated with group membership.
H and C. Hydrogen in group 1, and carbon in group 14, show some out-of-group similarities.[52] These include proximity in ionization energies, electron affinities and electronegativity values; half-filled valence shells; and correlations between the chemistry of H–H and C–H bonds.
H and N. Both are relatively unreactive colourless diatomic gases, with comparably high ionization energies (1312.0 and 1402.3 kJ/mol), each having half-valence subshells, 1s and 2p respectively. Like the reactive azide N3− anion, inter-electron repulsions in the H− hydride anion (with its single nuclear charge) make ionic hydrides highly reactive. Unusually for nonmetals, the two elements are known in cationic forms. In water the H+ "cation" exists as an H13O6+ ion, with a delocalised proton in a central OHO group.[53] Nitrogen forms an N5+ pentazenium cation; bulk quantities of the salt N5+SbF6− can be prepared. Coincidentally, the NH4+ ammonium cation behaves in many respects as an alkali metal anion.[54]
C and P. Carbon and phosphorus represent an example of a less-well known diagonal relationship, especially in organic chemistry. "Spectacular" evidence of this relationship was provided in 1987 with the synthesis of a ferrocene-like molecule in which six of the carbon atoms were replaced by phosphorus atoms.[54] Further illustrating the theme is the "extraordinary" similarity between low coordinate phosphorus compounds and unsaturated carbon compounds, and related research into organophosphorus chemistry.[55] In 2020, the first compound containing three carbon atoms and one phosphorus arranged in a tetrahedron, tri-tert-butyl phosphatetrahedrane, (PC3)(C4H9)3 was synthesised. While plain all-carbon tetrahedrane (CH)4 has never been isolated, phosphorus was selected in light of its capacity to form tetrahedral molecules, and the similarity of some of its properties to those of carbon.[56]
C and N. With nitrogen, carbon forms an extensive series of nitride compounds including those with high N:C ratios, and with structures that are simple (CN12); chain-like (C6N2 for example); graphitic (linked C6N7 units); fullerenic (C48N12) or polymeric (C3N3 units). Most of the compounds prepared to date also contain quantities of hydrogen.[57]
N and P. Like nitrogen, the chemistry of phosphorus is that of the covalent bond; the two nonmetals rarely form anions. Despite them being in the same group, and the composition of some of their compounds resembling one another, the individual chemistries of nitrogen and phosphorus are very different.[58] That said, the two elements form an extensive series of phosphorus–nitrogen compounds having chain, ring and cage structures; the P–N repeat unit in these structures bears a strong resemblance to the S–N repeat unit found in the wide range of sulfur–nitrogen compounds, discussed next.[59]
N and S. Nitrogen and sulfur have a less-well known diagonal relationship, manifested in like charge densities and electronegativities (the latter are identical if only the p electrons are counted; see Hinze and Jaffe 1962) especially when sulfur is bonded to an electron-withdrawing group. They are able to form an extensive series of seemingly interchangeable sulfur nitrides, the most famous of which, polymeric sulfur nitride, is metallic, and a superconductor below 0.26 K. The aromatic nature of the S3N22+ ion, in particular, serves as an "exemplar" of the similarity of electronic energies between the two nonmetals.[54]
N and O. Nitrogen and oxygen represent the main parts of air. They both become toxic under pressure thus, nitrogen narcosis; oxygen narcosis. They react readily with one another. Nitrogen forms several oxides, including nitrous oxide, N2O, in which nitrogen is in the +1 oxidation state; nitric oxide, NO, in which it is in the +2 state; and nitrogen dioxide, NO2, in which it is in the +4 state.
Many of the nitrogen oxides are extremely volatile; they are prime sources of pollution in the atmosphere. Nitrous oxide, also known as laughing gas, is sometimes used as an anaesthetic; when inhaled it produces mild hysteria. Nitric oxide reacts rapidly with oxygen to form brown nitrogen dioxide, an intermediate in the manufacture of nitric acid and a powerful oxidizing agent utilized in chemical processes and rocket fuels.
More generally nitrogen resembles oxygen with its high electronegativity and concomitant capability for hydrogen bonding and the ability to form coordination complexes by donating its lone pairs of electrons. There are some parallels between the chemistry of ammonia NH3 and water H2O. For example, the capacity of both compounds to be pronated to give NH4+ and H3O+ or deprotonated to give NH2− and OH−, with all of these able to be isolated in solid compounds.
O and S. Oxygen and sulfur react readily with one another, forming lower sulfur oxides (SnO, S7O2 and S6O2); sulfur monoxide (SO) and its dimer, disulfur dioxide (S2O2); sulfur dioxide (SO2); sulfur trioxide (SO3); higher sulfur oxides (SO3 and SO4 and polymeric condensates of them); and disulfur monoxide (S2O). The burning of coal and/or petroleum by industry and power plants generates sulfur dioxide (SO2) that reacts with atmospheric water and oxygen to produce sulfuric acid (H2SO4) and sulfurous acid (H2SO3). These acids are components of acid rain, lowering the pH of soil and freshwater bodies, sometimes resulting in substantial damage to the environment and chemical weathering of statues and structures. In most oxygen-containing organic molecules, the oxygen atoms can be replaced by sulfur atoms.
O and Cl. "Chlorination reactions have many similarities to oxidation reactions. They tend not to be limited to thermodynamic equilibrium and often go to complete chlorination. The reactions are often highly exothermic. Chlorine, like oxygen, forms flammable mixtures with organic compounds."[60]
O and F. Fluorine and oxygen share the ability to often bring out the highest oxidation states among the elements.
P and S (Se). Phosphorus reacts with sulfur and selenium (and oxygen) to form a large number of compounds. These compounds are characterized by structural analogies derived from the white phosphorus P4 tetrahedron.[61]
S and Se Commonalties between sulfur and selenium are abundantly obvious. For example, selenium is found in metal sulfide ores, where it partially replaces sulfur; both elements are photoconductors—their electrical conductivities increase by up to six orders of magnitude when exposed to light.[62]
I and Xe. The chemistry of iodine in its oxidation states of +1, +3, +5, and +7 is analogous to that of xenon in an immediately higher oxidation state.
Allotropes
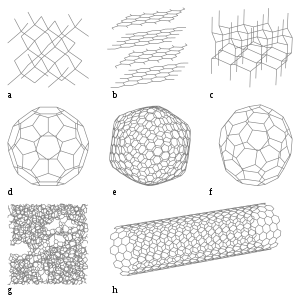
Many nonmetals have less stable allotropes, with either nonmetallic or metallic properties. Graphite, the standard state of carbon, has a lustrous appearance and is a fairly good electrical conductor. The diamond allotrope of carbon is clearly nonmetallic, however, being translucent and having a relatively poor electrical conductivity. Carbon is also known in several other allotropic forms, including semiconducting buckminsterfullerene (C60). Nitrogen can form gaseous tetranitrogen (N4), an unstable polyatomic molecule with a lifetime of about one microsecond.[63] Oxygen is a diatomic molecule in its standard state; it also exists as ozone (O3), an unstable nonmetallic allotrope with a half-life of around half an hour.[64] Phosphorus, uniquely, exists in several allotropic forms that are more stable than that of its standard state as white phosphorus (P4). The red and black allotropes are probably the best known; both are semiconductors. Phosphorus is also known as diphosphorus (P2), an unstable diatomic allotrope.[65] Sulfur has more allotropes than any other element;[66] all of these, except plastic sulfur (a metastable ductile mixture of allotropes)[67] have nonmetallic properties. Selenium has several nonmetallic allotropes, all of which are much less electrically conducting than its standard state of grey "metallic" selenium.[68] Iodine is also known in a semiconducting amorphous form.[69] Under sufficiently high pressures, just over half of the nonmetals, starting with phosphorus at 1.7 GPa,[70] have been observed to form metallic allotropes.
Most metalloids, like the less electronegative nonmetals, form allotropes. Boron is known in several crystalline and amorphous forms. The discovery of a quasispherical allotropic molecule borospherene (B40) was announced in July 2014. Silicon was most recently known only in its crystalline and amorphous forms. Silicene, a two-dimensional allotrope of silicon, with a hexagonal honeycomb structure similar to that of graphene, was observed in 2010. The synthesis of an orthorhombic allotrope Si24, was subsequently reported in 2014. At pressure of ~10–11 GPa, germanium transforms to a metallic phase with the same tetragonal structure as tin; when decompressed—and depending on the speed of pressure release—metallic germanium forms a series of allotropes that are metastable at ambient condition. Germanium also forms a graphene analogue, germanene. Arsenic and antimony form several well known allotropes (yellow, grey, and black). Tellurium is known only in its crystalline and amorphous forms; astatine is not known to have any allotropes.
Abundance and extraction
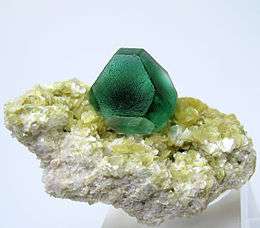
Hydrogen and helium are estimated to make up approximately 99 per cent of all ordinary matter in the universe. Less than five percent of the Universe is believed to be made of ordinary matter, represented by stars, planets and living beings. The balance is made of dark energy and dark matter, both of which are poorly understood at present.[71]
Hydrogen, carbon, nitrogen, and oxygen constitute the great bulk of the Earth's atmosphere, oceans, crust, and biosphere; the remaining nonmetals have abundances of 0.5 per cent or less. In comparison, 35 per cent of the crust is made up of the metals sodium, magnesium, aluminium, potassium and iron; together with a metalloid, silicon. All other metals and metalloids have abundances within the crust, oceans or biosphere of 0.2 per cent or less.[72]
Nonmetals, and metalloids, in their elemental forms are extracted from:[73] brine: Cl, Br, I; liquid air: N, O, Ne, Ar, Kr, Xe; minerals: B (borate minerals); C (coal; diamond; graphite); F (fluorite); Si (silica) P (phosphates); Sb (stibnite, tetrahedrite); I (in sodium iodate NaIO3 and sodium iodide NaI); natural gas: H, He, S; and from ores, as processing byproducts: Ge (zinc ores); As (copper and lead ores); Se, Te (copper ores); and Rn (uranium bearing ores). Astatine is produced in minute quantities by irradiating bismuth.
Applications in common
- For prevalent and speciality applications of individual nonmetals see the main article for each element.
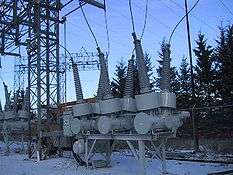
Nonmetals do not have any universal or near-universal applications. This is not the case with metals, most of which have structural uses; nor the metalloids, the typical uses of which extend to (for example) oxide glasses, alloying components, and semiconductors.
Shared applications of different subsets of the nonmetals instead encompass their presence in, or specific uses in the fields of cryogenics and refrigerants: H, He, N, O, F and Ne; fertilisers: H, N, P, S, Cl (as a micronutrient) and Se; household accoutrements: H (primary constituent of water), He (party balloons), C (in pencils, as graphite), N (beer widgets), O (as peroxide, in detergents), F (as fluoride, in toothpaste), Ne (lighting), P (matches), S (garden treatments), Cl (bleach constituent), Ar (insulated windows), Se (glass; solar cells), Br (as bromide, for purification of spa water), Kr (energy saving fluorescent lamps), I (in antiseptic solutions), Xe (in plasma TV display cells, a technology subsequently made redundant by low cost OLED displays), while Rn also sometimes occurs, but then as an unwanted, potentially hazardous indoor pollutant;[75] industrial acids: C, N, F, P, S and Cl; inert air replacements: N, Ne, S (in sulfur hexafluoride SF6), Ar, Kr and Xe; lasers and lighting: He, C (in carbon dioxide lasers, CO2), N, O (in a chemical oxygen iodine laser), F (in a hydrogen fluoride laser, HF), Ne, S (in a sulfur lamp), Ar, Kr and Xe; and medicine and pharmaceuticals: He, O, F, Cl, Br, I, Xe and Rn.
The number of compounds formed by nonmetals is vast.[76] The first nine places in a "top 20" table of elements most frequently encountered in 8,427,300 compounds, as listed in the Chemical Abstracts Service register for July 1987, were occupied by nonmetals. Hydrogen, carbon, oxygen and nitrogen were found in the majority (greater than 64 per cent) of compounds. Silicon, a metalloid, was in 10th place. The highest rated metal, with an occurrence frequency of 2.3 per cent, was iron, in 11th place.[77]
Discovery
Antiquity: C, S, (Sb)
Carbon, sulfur, and antimony were known in antiquity. The earliest known use of charcoal dates to around 3750 BCE. The Egyptians and Sumerians employed it for the reduction of copper, zinc, and tin ores in the manufacture of bronze. Diamonds were probably known from as early as 2500 BCE. The first true chemical analyses were made in the 18th century; Lavoisier recognized carbon as an element in 1789. Sulfur usage dates from before 2500 BCE; it was recognized as an element by Antoine Lavoisier in 1777. Antimony usage was concurrent with that of sulfur; the Louvre holds a 5,000 year old vase made of almost pure antimony.
13th century: (As)
Albertus Magnus (Albert the Great, 1193–1280) is believed to have been the first to isolate the element from a compound in 1250, by heating soap together with arsenic trisulfide. If so, it was the first element to be chemically discovered.
17th century: P
Phosphorus was prepared from urine, by Hennig Brand, in 1669.
18th century: H, O, N, (Te), Cl
Hydrogen: Cavendish, in 1766, was the first to distinguish hydrogen from other gases, although Paracelsus around 1500, Robert Boyle (1670), and Joseph Priestley (?) had observed its production by reacting strong acids with metals. Lavoisier named it in 1793. Oxygen: Carl Wilhelm Scheele obtained oxygen by heating mercuric oxide and nitrates in 1771, but did not publish his findings until 1777. Priestley also prepared this new "air" by 1774, but only Lavoisier recognized it as a true element; he named it in 1777. Nitrogen: Rutherford discovered nitrogen while he was studying at the University of Edinburgh. He showed that the air in which animals breathed, after removal of exhaled carbon dioxide, was no longer able to burn a candle. Scheele, Henry Cavendish, and Priestley also studied this element at about the same time; Lavoisier named it in 1775 or 1776. Tellurium: In 1783, Franz-Joseph Müller von Reichenstein, who was then serving as the Austrian chief inspector of mines in Transylvania, concluded that a new element was present in a gold ore from the mines in Zlatna, near today's city of Alba Iulia, Romania. In 1789, a Hungarian scientist, Pál Kitaibel, discovered the element independently in an ore from Deutsch-Pilsen that had been regarded as argentiferous molybdenite, but later he gave the credit to Müller. In 1798, it was named by Martin Heinrich Klaproth, who had earlier isolated it from the mineral calaverite. Chlorine: In 1774, Scheele obtained chlorine from hydrochloric acid but thought it was an oxide. Only in 1808 did Humphry Davy recognize it as an element.
Early 19th century: (B) I, Se, (Si), Br
Boron was identified by Sir Humphry Davy in 1808 but not isolated in a pure form until 1909, by the American chemist Ezekiel Weintraub. Iodine was discovered in 1811 by Courtois from the ashes of seaweed. Selenium: In 1817, when Berzelius and Johan Gottlieb Gahn were working with lead they discovered a substance that was similar to tellurium. After more investigation Berzelius concluded that it was a new element, related to sulfur and tellurium. Because tellurium had been named for the Earth, Berzelius named the new element "selenium", after the moon. Silicon: In 1823, Berzelius prepared amorphous silicon by reducing potassium fluorosilicate with molten potassium metal. Bromine: Balard and Gmelin both discovered bromine in the autumn of 1825 and published their results in the following year.
Late 19th century: He, F, (Ge), Ar, Kr, Ne, Xe
Helium: In 1868, Janssen and Lockyer independently observed a yellow line in the solar spectrum that did not match that of any other element. In 1895, in each case at around the same time, Ramsay, Cleve, and Langlet independently observed helium trapped in cleveite. Fluorine: André-Marie Ampère predicted an element analogous to chlorine obtainable from hydrofluoric acid, and between 1812 and 1886 many researchers tried to obtain it. Fluorine was eventually isolated in 1886 by Moissan. Germanium: In mid-1885, at a mine near Freiberg, Saxony, a new mineral was discovered and named argyrodite because of its silver content. The chemist Clemens Winkler analyzed this new mineral, which proved to be a combination of silver, sulfur, and a new element, germanium, which he was able to isolate in 1886. Argon: Lord Rayleigh and Ramsay discovered argon in 1894 by comparing the molecular weights of nitrogen prepared by liquefaction from air, and nitrogen prepared by chemical means. It was the first noble gas to be isolated. Krypton, neon, and xenon: In 1898, within a period of three weeks, Ramsay and Travers successively separated krypton, neon and xenon from liquid argon by exploiting differences in their boiling points.
20th century: Rn, (At)
In 1898, Friedrich Ernst Dorn discovered a radioactive gas resulting from the radioactive decay of radium; Ramsay and Robert Whytlaw-Gray subsequently isolated radon in 1910. Astatine was synthesised in 1940 by Dale R. Corson, Kenneth Ross MacKenzie, and Emilio Segrè. They bombarded bismuth-209 with alpha particles in a cyclotron to produce, after emission of two neutrons, astatine-211.
Notes
- An ionisation energy of less than 750 kJ/mol is taken to be low, 750–1000 is moderate, and > 1000 is high (> 2000 is very high); an electron affinity of less than 70 kJ/mol is taken to be low, 70–140 is moderate, and > 140 is high; an electronegativity of less than 1.8 is taken to be low; 1.8–2.2 is moderate; and > than 2.2 is high (> 4.0 is very high).
- Revised Pauling values are used for the metalloids, and reactive nonmetals; Allred-Rochow values for the noble gases
- The nonmetallic halogens (F, Cl, Br, I) readily form anions including in aqueous solution; the oxide ion O2− is unstable in aqueous solution—its affinity for H+ is so great that it abstracts a proton from a solvent H2O molecule (O2− + H2O → 2 OH−)—but is found in an extensive series of metal oxides
- The common oxide is the most stable oxide for that element
References
Data sources
Unless otherwise stated, melting points, boiling points, densities, crystalline structures, ionisation energies, electron affinities, and electronegativity values are from the CRC Handbook of Physics and Chemistry;[78] standard electrode potentials are from the 1989 compilation by Steven Bratsch.[79]
Citations
- Sukys 1999, p. 60.
- Bettelheim et al. 2016, p. 33.
- Schulze-Makuch & Irwin 2008, p. 89.
- Steurer 2007, p. 7.
- Cox 2004, p. 26
- Meyer et al. 2005, p. 284; Manahan 2001, p. 911; Szpunar et al. 2004, p. 17
- Brown & Rogers 1987, p. 40
- Kneen, Rogers & Simpson 1972, p. 262
- Greenwood & Earnshaw 2002, p. 434
- Bratsch 1989; Bard, Parsons & Jordan 1985, p. 133
- Yoder, Suydam & Snavely 1975, p. 58
- Kneen, Rogers & Simpson 1972, p. 360
- Lee 1996, p. 240
- Greenwood & Earnshaw 2002, p. 43
- Cressey 2010
- Siekierski & Burgess 2002, p. 24–25
- Siekierski & Burgess 2002, p. 23
- Cox 2004, p. 146
- Kneen, Rogers & Simpson 1972, p. 362
- Bailar et al. 1989, p. 742
- Stein 1983, p. 165
- Jolly 1966, p. 20
- Clugston & Flemming 2000, pp. 100–1, 104–5, 302
- Seaborg 1969, p. 626
- Nash 2005
- Scerri 2013, pp. 204–8
- Challoner 2014, p. 5; Government of Canada 2015; Gargaud et al. 2006, p. 447
- Ivanenko et al. 2011, p. 784
- Catling 2013, p. 12
- Crawford 1968, p. 540
- Berkowitz 2012, p. 293
- Jørgensen & Mitsch 1983, p. 59
- Wulfsberg 1987, p. 159–160
- Bettelheim et al. 2016, p. 33—34
- Field & Gray 2011, p. 12
- Dinwiddle et al. 2018, pp. 34–35
- Vernon 2020
- Dingle 2017, pp. 9, 101, 179
- Myers, Oldham & Tocci 2004, pp. 120–121
- Stein 1969; Pitzer 1975; Schrobilgen 2011
- Brasted 1974, p. 814
- Sidorov 1960
- Rochow 1966, p. 4
- Atkins 2006 et al., pp. 8, 122–23
- Ritter 2011, p. 10
- Wiberg 2001, p. 680
- Wiberg 2001, p. 403
- Greenwood & Earnshaw 2002, p. 612
- Moeller 1952, p. 208
- Cotton 2003, p. 205
- Wulfsberg 1987, p. 159
- Cronyn 2003
- Stoyanov et al.
- Rayner-Canham 2011, p. 126
- Dillon, Mathey & Nixon 1998
- Martin-Louis et al. 2020
- Miller et al.
- Wiberg 2001, p. 686
- Roy et al. 1994
- Kent 2007, p. 104
- Monteil & Vincent 1976
- Moss 1952
- Cacace, de Petris & Troiani 2002
- Koziel 2002, p. 18
- Piro et al. 2006
- Steudel & Eckert 2003, p. 1
- Greenwood & Earnshaw 2002, pp. 659–660
- Moss 1952, p. 192; Greenwood & Earnshaw 2002, p. 751
- Shanabrook, Lannin & Hisatsune 1981
- Yousuf 1998, p. 425
- Ostriker & Steinhardt 2001
- Nelson 1987, p. 732
- Emsley 2001, p. 428
- Bolin 2012, p. 2-1
- Maroni 1995
- King & Caldwell 1954, p. 17; Brady & Senese 2009, p. 69
- Nelson 1987, p. 735
- Lide 2003
- Bratsch 1989
Bibliography
- Addison WE 1964, The allotropy of the elements, Oldbourne Press, London
- Arunan E, Desiraju GR, Klein RA, Sadlej J, Scheiner S, Alkorta I, Clary DC, Crabtree RH, Dannenberg JJ, Hobza P, Kjaergaard HG, Legon AC, Mennucci B & Nesbitt DJ 2011, "Defining the hydrogen bond: An account (IUPAC Technical Report)", Pure and Applied Chemistry, vol. 83, no. 8, pp. 1619–36, doi:10.1351/PAC-REP-10-01-01
- Ashford TA 1967, The physical sciences: From atoms to stars, 2nd ed., Holt, Rinehart and Winston, New York
- Atkins P & de Paula J 2011, Physical chemistry for the life sciences, 2nd ed., Oxford University Press, Oxford, ISBN 978-1429231145
- Aylward G & Findlay T 2008, SI chemical data, 6th ed., John Wiley & Sons Australia, Milton, Queensland
- Bailar JC, Moeller T, Kleinberg J, Guss CO, Castellion ME & Metz C 1989, Chemistry, 3rd ed., Harcourt Brace Jovanovich, San Diego, ISBN 0-15-506456-8
- Ball P 2013, "The name's bond", Chemistry World, vol. 10, no. 6, p. 41
- Bard AJ, Parsons R & Jordan J 1985, Standard potentials in aqueous solution, Marcel Dekker, New York, ISBN 978-0-8247-7291-8
- Berkowitz J 2012, The stardust revolution: The new story of our origin in the stars, Prometheus Books, Amherst, New York, ISBN 978-1-61614-549-1
- Bettelheim FA, Brown WH, Campbell MK, Farrell SO 2010, Introduction to general, organic, and biochemistry, 9th ed., Brooks/Cole, Belmont California, ISBN 9780495391128
- Bettelheim FA, Brown WH, Campbell MK, Farrell SO & Torres OJ 2016, Introduction to general, organic, and biochemistry, 11th ed., Cengage Learning, Boston, ISBN 978-1-285-86975-9
- Bogoroditskii NP & Pasynkov VV 1967, Radio and electronic materials, Iliffe Books, London
- Bolin P 2000, "Gas-insulated substations, in JD McDonald (ed.), Electric power substations engineering, 3rd, ed., CRC Press, Boca Raton, FL, pp. 2–1–2-19, ISBN 9781439856383
- Borg RJ & Dienes GJ 1992, The physical chemistry of solids, Academic Press, San Diego, California, ISBN 9780121184209
- Brady JE & Senese F 2009, Chemistry: The study of matter and its changes, 5th ed., John Wiley & Sons, New York, ISBN 9780470576427
- Bratsch SG 1989, "Standard electrode potentials and temperature coefficients in water at 298.15 K," Journal of Physical Chemical Reference Data, vol. 18, no. 1, pp. 1–21, doi:10.1063/1.555839
- Brown WH & Rogers EP 1987, General, organic and biochemistry, 3rd ed., Brooks/Cole, Monterey, California, ISBN 0534068707
- Bryson PD 1989, Comprehensive review in toxicology, Aspen Publishers, Rockville, Maryland, ISBN 0871897776
- Bunge AV & Bunge CF 1979, "Electron affinity of helium (1s2s)3S", Physical Review A, vol. 19, no. 2, pp. 452–456, doi:10.1103/PhysRevA.19.452
- Cacace F, de Petris G & Troiani A 2002, "Experimental detection of tetranitrogen", Science, vol. 295, no. 5554, pp. 480–81, doi:10.1126/science.1067681
- Cairns D 2012, Essentials of pharmaceutical chemistry, 4th ed., Pharmaceutical Press, London, ISBN 9780853699798
- Cambridge Enterprise 2013, "Carbon 'candy floss' could help prevent energy blackouts", Cambridge University, viewed 28 August 2013
- Catling DC 2013, Astrobiology: A very short introduction, Oxford University Press, Oxford, ISBN 978-0-19-958645-5
- Challoner J 2014, The elements: The new guide to the building blocks of our universe, Carlton Publishing Group, ISBN 978-0-233-00436-5
- Chapman B & Jarvis A 2003, Organic chemistry, kinetics and equilibrium, rev. ed., Nelson Thornes, Cheltenham, ISBN 978-0-7487-7656-6
- Chung DD 1987, "Review of exfoliated graphite", Journal of Materials Science, vol. 22, pp. 4190–98, doi:10.1007/BF01132008
- Clugston MJ & Flemming R 2000, Advanced chemistry, Oxford University Press, Oxford, ISBN 9780199146338
- Conroy EH 1968, "Sulfur", in CA Hampel (ed.), The encyclopedia of the chemical elements, Reinhold, New York, pp. 665–680
- Cotton FA, Darlington C & Lynch LD 1976, Chemistry: An investigative approach, Houghton Mifflin, Boston ISBN 978-0-395-21671-2
- Cotton S 2006, Lanthanide and actinide chemistry, 2nd ed., John Wiley & Sons, New York, ISBN 9780470010068
- Cox T 2004, Inorganic chemistry, 2nd ed., BIOS Scientific Publishers, London, ISBN 1-85996-289-0
- Cracolice MS & Peters EI 2011, Basics of introductory chemistry: An active learning approach, 2nd ed., Brooks/Cole, Belmont California, ISBN 9780495558507
- Crawford FH 1968, Introduction to the science of physics, Harcourt, Brace & World, New York
- Cressey 2010, "Chemists re-define hydrogen bond", Nature newsblog, accessed 23 August 2017
- Cronyn MW 2003, "The proper place for hydrogen in the periodic table", Journal of Chemical Education, vol. 80, no. 8, pp. 947–951, doi:10.1021/ed080p947
- Daniel PL & Rapp RA 1976, "Halogen corrosion of metals", in MG Fontana & RW Staehle (eds), Advances in corrosion science and technology, Springer, Boston, pp. 55–172, doi:10.1007/978-1-4615-9062-0_2
- DeKock RL & Gray HB 1989, Chemical structure and bonding, 2nd ed., University Science Books, Mill Valley, California, ISBN 093570261X
- Desch CH 1914, Intermetallic Compounds, Longmans, Green and Co., New York
- Dias RP, Yoo C, Kim M & Tse JS 2011, "Insulator-metal transition of highly compressed carbon disulfide," Physical Review B, vol. 84, pp. 144104–1–6, doi:10.1103/PhysRevB.84.144104
- Dillon KB, Mathey F & Nixon JF 1998, Phosphorus: The carbon copy: From organophosphorus to phospha-organic chemistry, John Wiley & Sons, Chichester
- Dingle A 2017, The elements: An encyclopedic tour of the periodic table, Quad Books, Brighton, ISBN 978-0-85762-505-2
- Dinwiddle R, Lamb H, Franceschetti DR & Viney M (eds) 2018, How science works, Dorling Kindersley, London
- Donohue J 1982, The structures of the elements, Robert E. Krieger, Malabar, Florida, ISBN 0-89874-230-7
- Eagleson M 1994, Concise encyclopedia chemistry, Walter de Gruyter, Berlin, ISBN 3110114518
- Eastman ED, Brewer L, Bromley LA, Gilles PW, Lofgren NL 1950, "Preparation and properties of refractory cerium sulfides", Journal of the American Chemical Society, vol. 72, no. 5, pp. 2248–50, doi:10.1021/ja01161a102
- Emsley J 1971, The inorganic chemistry of the non-metals, Methuen Educational, London, ISBN 0423861204
- Emsley J 2001, Nature's building blocks: An A–Z guide to the elements, Oxford University Press, Oxford, ISBN 0198503415
- Faraday M 1853, The subject matter of a course of six lectures on the non-metallic elements, (arranged by John Scoffern), Longman, Brown, Green, and Longmans, London
- Field SQ & Gray T 2011, Theodore Gray's elements vault, Black Dog & Leventhal Publishers, New York, ISBN 978-1-57912-880-7
- Finney J 2015, Water: A Very Short Introduction, Oxford University Press, Oxford, ISBN 978-0198708728,
- Fujimori T, Morelos-Gómez A, Zhu Z, Muramatsu H, Futamura R, Urita K, Terrones M, Hayashi T, Endo M, Hong SY, Choi YC, Tománek D & Kaneko K 2013, "Conducting linear chains of sulphur inside carbon nanotubes", Nature Communications, vol. 4, article no. 2162, doi:10.1038/ncomms3162
- Gargaud M, Barbier B, Martin H & Reisse J (eds) 2006, Lectures in astrobiology, vol. 1, part 1: The early Earth and other cosmic habitats for life, Springer, Berlin, ISBN 3-540-29005-2
- Government of Canada 2015, Periodic table of the elements, accessed 30 August 2015
- Godfrin H & Lauter HJ 1995, "Experimental properties of 3He adsorbed on graphite", in WP Halperin (ed.), Progress in low temperature physics, volume 14, pp. 213–320 (216–8), Elsevier Science B.V., Amsterdam, ISBN 9780080539935
- Greenwood NN & Earnshaw A 2002, Chemistry of the elements, 2nd ed., Butterworth-Heinemann, ISBN 0750633654
- Henderson W 2000, Main group chemistry, Royal Society of Chemistry, Cambridge, ISBN 9780854046171
- Holderness A & Berry M 1979, Advanced level inorganic chemistry, 3rd ed., Heinemann Educational Books, London, ISBN 9780435654351
- Irving KE 2005, "Using chime simulations to visualize molecules", in RL Bell & J Garofalo (eds), Science units for Grades 9–12, International Society for Technology in Education, Eugene, Oregon, ISBN 9781564842176
- Ivanenko NB, Ganeev AA, Solovyev ND & Moskvin LN 2011, "Determination of trace elements in biological fluids", Journal of Analytical Chemistry, vol. 66, no. 9, pp. 784–799 (784), doi:10.1134/S1061934811090036
- Jenkins GM & Kawamura K 1976, Polymeric carbons—carbon fibre, glass and char, Cambridge University Press, Cambridge, ISBN 0521206936
- Jolly WL 1966, The chemistry of the non-metals, Prentice-Hall, Englewood Cliffs, New Jersey
- Jones WN 1969, Textbook of general chemistry, C. V. Mosby Company, St Louis, ISBN 978-0-8016-2584-8
- Jorgensen CK 2012, Oxidation numbers and oxidation states, Springer-Verlag, Berlin, ISBN 978-3-642-87760-5
- Jørgensen SE & Mitsch WJ (eds) 1983, Application of ecological modelling in environmental management, part A, Elsevier Science Publishing, Amsterdam, ISBN 0-444-42155-6
- Keith JA & Jacob T 2010, "Computational simulations on the oxygen reduction reaction in electrochemical systems", in PB Balbuena & VR Subramanian, Theory and experiment in electrocatalysis, Modern aspects of electrochemistry, vol. 50, Springer, New York, pp. 89–132, ISBN 978-1-4419-5593-7
- Kent JA 2007, Kent and Riegel's Handbook of industrial chemistry and biotechnology, 11th ed., vol. 1, Spring Science + Business Media, New York, ISBN 978-0-387-27842-1
- King RB 2004, "The metallurgist's periodic table and the Zintl-Klemm concept", in DH Rouvray & BR King (eds), The periodic table: into the 21st century, Research Studies Press, Philadelphia, pp. 189–206, ISBN 0863802923
- King GB & Caldwell WE 1954, The fundamentals of college chemistry, American Book Company, New York
- Kneen WR, Rogers MJW & Simpson P 1972, Chemistry: Facts, patterns, and principles, Addison-Wesley, London, ISBN 0201037793
- Koziel JA 2002, "Sampling and sample preparation for indoor air analysis", in J Pawliszyn (ed.), Comprehensive analytical chemistry, vol. 37, Elsevier Science B.V., Amsterdam, pp. 1–32, ISBN 0444505105
- Krikorian OH & Curtis PG 1988, "Synthesis of CeS and interactions with molten metals", High Temperatures – High Pressures, vol. 20, pp. 9–17, ISSN 0018-1544
- Labes MM, Love P & Nichols LF 1979, "Polysulfur nitride—a metallic, superconducting polymer", Chemical Review, vol. 79, no. 1, pp. 1–15, doi:10.1021/cr60317a002
- Lee JD 1996, Concise inorganic chemistry, 5th ed., Blackwell Science, Oxford, ISBN 978-0-6320-5293-6
- Lide DR (ed.) 2003, CRC handbook of chemistry and physics, 84th ed., CRC Press, Boca Raton, Florida, Section 6, Fluid properties; Vapor pressure, ISBN 0849304849
- Manahan SE 2001, Fundamentals of environmental chemistry, 2nd ed., CRC Press, Boca Raton, Florida, ISBN 156670491X
- Maroni M, Seifert B & Lindvall T (eds) 1995, "Physical pollutants", in Indoor air quality: A comprehensive reference book, Elsevier, Amsterdam, pp. 108–123, ISBN 0444816429
- Martin RM & Lander GD 1946, Systematic inorganic chemistry: From the standpoint of the periodic law, 6th ed., Blackie & Son, London
- Martin-Louis Y, Riu RL, Jones WJ, Transue PM & Cummins CC 2020, "Isolation of an elusive phosphatetrahedrane", Science Advances, vol. 6, no. 13, doi:10.1126/sciadv.aaz3168
- McCall BJ & Oka T 2003, "Enigma of H3+ in diffuse interstellar clouds", in SL Guberman (ed.), Dissociative recombination of molecular ions with electrons, Springer Science+Business Media, New York, ISBN 978-1-4613-4915-0
- McMillan PF 2006, "Solid-state chemistry: A glass of carbon dioxide", Nature, vol. 441, p. 823, doi:10.1038/441823a
- Merchant SS & Helmann JD 2012, "Elemental economy: Microbial strategies for optimizing growth in the face of nutrient limitation", in Poole RK (ed), Advances in Microbial Physiology, vol. 60, pp. 91–210, doi:10.1016/B978-0-12-398264-3.00002-4
- Meyer JS, Adams WJ, Brix KV, Luoma SM, Mount DR, Stubblefield WA & Wood CM (eds) 2005, Toxicity of dietborne metals to aquatic organisms, Proceedings from the Pellston Workshop on Toxicity of Dietborne Metals to Aquatic Organisms, 27 July–1 August 2002, Fairmont Hot Springs, British Columbia, Canada, Society of Environmental Toxicology and Chemistry, Pensacola, Florida, ISBN 1880611708
- Miller T 1987, Chemistry: a basic introduction, 4th ed., Wadsworth, Belmont, California, ISBN 0534069126
- Miller, T.S., Belen, A., Suter, T.M., Sella, A., Corà, A., McMillan, P.F.: Carbon nitrides: synthesis and characterization of a new class of functional materials. Phys. Chem. Chem. Phys. 24, 15613–15638 (2017)
- Mitchell JBA & McGowan JW 1983, "Experimental studies of electron-ion combination", Physics of ion-ion and electron-ion collisions, F Brouillard F & JW McGowan (eds), Plenum Press, ISBN 978-1-4613-3547-4
- Mitchell SC 2006, "Biology of sulfur", in SC Mitchell (ed.), Biological interactions of sulfur compounds, Taylor & Francis, London, pp. 20–41, ISBN 0203375122
- Moeller T 1952, Inorganic chemistry: An advanced textbook, John Wiley & Sons, New York
- Monteil, Y., Vincent, H.: Phosphorus compounds with the VI B group elements. Z. Naturforsch. B. J. Chem. Sci. 31b(5), 668–672 (1976)
- Moss, T.S.: Photoconductivity in the elements. Butterworths Scientific, London, pp. 180, 202 (1952). For amorphous selenium, the increase in conductivity is a thousand-fold; for "metallic" selenium the increase is from three to as much as two-hundred fold. Mikla, V.I.; Mikla, V.V.: Amorphous Chalcogenides: The Past, Present and Future. Elsevier, Boston, p. 63 (2012); Yost, D.M., Russell, H. Systematic Inorganic Chemistry of the Fifth-and-Sixth-Group Nonmetallic Elements. Prentice-Hall, New York, p. 282 (1946)
- Murray PRS & Dawson PR 1976, Structural and comparative inorganic chemistry: A modern approach for schools and colleges, Heinemann Educational Book, London, ISBN 9780435656447
- Myers RT, Oldham KB & Tocci S 2004, Holt Chemistry, teacher ed., Holt, Rinehart & Winston, Orlando, ISBN 0-03-066463-2
- Nash CS 2005, "Atomic and molecular properties of elements 112, 114, and 118", Journal of Physical Chemistry A, vol. 109, pp. 3493–500, doi:10.1021/jp050736o
- Nelson PG 1987, "Important elements", Journal of Chemical Education, vol. 68, no. 9, pp. 732–737, doi:10.1021/ed068p732
- Nelson PG 1998, "Classifying substances by electrical character: An alternative to classifying by bond type", Journal of Chemical Education, vol. 71, no. 1, pp. 24–6, doi:10.1021/ed071p24
- Novak A 1979, "Vibrational spectroscopy of hydrogen bonded systems", in TM Theophanides (ed.), Infrared and Raman spectroscopy of biological molecules, proceedings of the NATO Advanced Study Institute held at Athens, Greece, August 22–31, 1978, D. Reidel Publishing Company, Dordrecht, Holland, pp. 279–304, ISBN 9027709661
- Oka T 2006, "Interstellar H+
3", PNAS, vol. 103, no. 33, doi:10.1073_pnas.0601242103 - Ostriker JP & Steinhardt PJ 2001, "The quintessential universe", Scientific American, January, pp. 46–53
- Oxtoby DW, Gillis HP & Campion A 2008, Principles of modern chemistry, 6th ed., Thomson Brooks/Cole, Belmont, California, ISBN 0534493661
- Partington JR 1944, A text-book of inorganic chemistry, 5th ed., Macmillan & Co., London
- Patil UN, Dhumal NR & Gejji SP 2004, "Theoretical studies on the molecular electron densities and electrostatic potentials in azacubanes", Theoretica Chimica Acta, vol. 112, no. 1, pp 27–32, doi:10.1007/s00214-004-0551-2
- Patten MN 1989, Other metals and some related materials, in MN Patten (ed.), Information sources in metallic materials, Bowker-Saur, London, ISBN 0408014911
- Patterson CS, Kuper HS & Nanney TR 1967, Principles of chemistry, Appleton Century Crofts, New York
- Pearson WB 1972, The crystal chemistry and physics of metals and alloys, Wiley-Interscience, New York, ISBN 0-471-67540-7
- Pearson RG & Mawby RJ 1967, "The nature of metal–halogen bonds", in V Gutmann (ed.), Halogen chemistry, Academic Press, pp. 55–84
- Phifer C 2000, "Ceramics, glass structure and properties", in Kirk-Othmer Encyclopedia of Chemical Technology, doi:10.1002/0471238961.0712011916080906.a01
- Phillips CSG & Williams RJP 1965, Inorganic chemistry, I: Principles and non-metals, Clarendon Press, Oxford
- Piro NA, Figueroa JS, McKellar JT & Troiani CC 2006, "Triple-bond reactivity of diphosphorus molecules", Science, vol. 313, no. 5791, pp. 1276–9, doi:10.1126/science.1129630
- Pitzer K 1975, "Fluorides of radon and elements 118", Journal of the Chemical Society, Chemical Communications, no. 18, pp. 760–1, doi:10.1039/C3975000760B
- Raju GG 2005, Gaseous Electronics: Theory and Practice, CRC Press, Boca Raton, Florida, ISBN 978-0-203-02526-0
- Rao KY 2002, Structural chemistry of glasses, Elsevier, Oxford, ISBN 0080439586
- Rayner-Canham G 2011, "Isodiagonality in the periodic table", Foundations of Chemistry, vol. 13, no. 2, pp. 121–129, doi:10.1007/s10698-011-9108-y
- Rayner-Canham G & Overton T 2006, Descriptive inorganic chemistry, 4th ed., WH Freeman, New York, ISBN 0716789639
- Regnault MV 1853, Elements of chemistry, vol. 1, 2nd ed., Clark & Hesser, Philadelphia
- Ritter SK 2011, "The case of the missing xenon", Chemical & Engineering News, vol. 89, no. 9, ISSN 0009-2347
- Rochow EG 1966, The Metalloids, DC Heath and Company, Boston
- Rodgers GE 2012, Descriptive inorganic, coordination, & solid-state chemistry, 3rd ed., Brooks/Cole, Belmont, California, ISBN 9780840068460
- Roy, A.K., Burns, G.T., Grigora, S., Lie, G.C.: Poly(alkyl/aryloxothiazenes), [N = S(O)R]n: New direction in inorganic polymers. In: Wisian-Neilson, P., Alcock, H.R., Wynne, K.J. (eds.) Inorganic and Organometallic Polymers II: Advanced Materials and Intermediates, pp. 344–357. American Chemical Society, Washington DC (1994)
- Russell AM & Lee KL 2005, Structure-property relations in nonferrous metals, Wiley-Interscience, New York, ISBN 047164952X
- Scerri E 2013, A tale of seven elements, Oxford University Press, Oxford, ISBN 9780195391312
- Schaefer JC 1968, "Boron" in CA Hampel (ed.), The encyclopedia of the chemical elements, Reinhold, New York, pp. 73–81
- Scharfe ME & Schmidlin FW 1975, "Charged pigment xerography", in L Marton (ed.), Advances in Electronics and Electron Physics, vol. 38, Academic Press, New York, ISBN 0-12-014538-3, pp. 93–147
- Schrobilgen GJ 2011, "radon (Rn)", in Encyclopædia Britannica, accessed 7 Aug 2011
- Schulze-Makuch D & Irwin LN 2008, Life in the Universe: Expectations and constraints, 2nd ed., Springer-Verlag, Berlin, ISBN 9783540768166
- Seaborg GT 1969, "Prospects for further considerable extension of the periodic table", Journal of Chemical Education, vol. 46, no. 10, pp. 626–34, doi:10.1021/ed046p626
- Shanabrook BV, Lannin JS & Hisatsune IC 1981, "Inelastic light scattering in a onefold-coordinated amorphous semiconductor", Physical Review Letters, vol. 46, no. 2, 12 January, pp. 130–133
- Sherwin E & Weston GJ 1966, Chemistry of the non-metallic elements, Pergamon Press, Oxford
- Shipman JT, Wilson JD & Todd AW 2009, An introduction to physical science, 12th ed., Houghton Mifflin Company, Boston, ISBN 9780618935963
- Siebring BR & Schaff ME 1980, General chemistry, Wadsworth Publishing, Belmont, California
- Siekierski S & Burgess J 2002, Concise chemistry of the elements, Horwood Press, Chichester, ISBN 1-898563-71-3
- Silvera I & Walraven JTM 1981, "Monatomic hydrogen – a new stable gas", New Scientist, 22 January
- Smith MB 2011, Organic Chemistry: An Acid—Base Approach, CRC Press, Boca Raton, Florida, ISBN 978-1-4200-7921-0
- Stein L 1969, "Oxidized radon in halogen fluoride solutions", Journal of the American Chemical Society, vol. 19, no. 19, pp. 5396–7, doi:10.1021/ja01047a042
- Stein L 1983, "The chemistry of radon", Radiochimica Acta, vol. 32, pp. 163–71
- Steudel R 1977, Chemistry of the non-metals: With an introduction to atomic structure and chemical bonding, Walter de Gruyter, Berlin, ISBN 3110048825
- Steudel R 2003, "Liquid sulfur", in R Steudel (ed.), Elemental sulfur and sulfur-rich compounds I, Springer-Verlag, Berlin, pp. 81–116, ISBN 9783540401919
- Steudel R & Eckert B 2003, "Solid sulfur allotropes", in R Steudel (ed.), Elemental sulfur and sulfur-rich compounds I, Springer-Verlag, Berlin, pp. 1–80, ISBN 9783540401919
- Steudel R & Strauss E 1984, "Homcyclic selenium molecules and related cations", in HJ Emeleus (ed.), Advances in inorganic chemistry and radiochemistry, vol. 28, Academic Press, Orlando, Florida, pp. 135–167, ISBN 9780080578774
- Steurer W 2007, "Crystal structures of the elements" in JW Marin (ed.), Concise encyclopedia of the structure of materials, Elsevier, Oxford, pp. 127–45, ISBN 0080451276
- Stoyanov, E.S., Stoyanova, I.V., Reed, C.A.: The structure of the hydrogen ion (H+aq). J. Am. Chem. Soc. 132(5), 1484–1485 (2010)
- Stwertka A 2012, A guide to the elements, 3rd ed., Oxford University Press, Oxford, ISBN 9780199832521
- Sukys P 1999, Lifting the scientific veil: Science appreciation for the nonscientist, Rowman & Littlefield, Oxford, ISBN 0847696006
- Szpunar J, Bouyssiere B & Lobinski R 2004, "Advances in analytical methods for speciation of trace elements in the environment", in AV Hirner & H Emons (eds), Organic metal and metalloid species in the environment: Analysis, distribution processes and toxicological evaluation, Springer-Verlag, Berlin, pp. 17–40, ISBN 3540208291
- Taylor MD 1960, First principles of chemistry, Van Nostrand, Princeton, New Jersey
- Townes CH & Dailey BP 1952, "Nuclear quadrupole effects and electronic structure of molecules in the solid state", Journal of Chemical Physics, vol. 20, pp. 35–40, doi:10.1063/1.1700192
- Van Setten MJ, Uijttewaal MA, de Wijs GA & Groot RA 2007, 'Thermodynamic Stability of Boron: The Role of Defects and Zero Point Motion', Journal of the American Chemical Society, vol. 129, no. 9, pp. 2458–65, doi:10.1021/ja0631246
- Vernon RE 2020, "Organising the metals and nonmetals," Foundations of Chemistry, pp. 1−17, doi:10.1007/s10698-020-09356-6 (open access)
- Wells AF 1984, Structural inorganic chemistry, 5th ed., Clarendon Press, Oxfordshire, ISBN 0198553706
- Wiberg N 2001, Inorganic chemistry, Academic Press, San Diego, ISBN 0123526515
- Winkler MT 2009, "Non-equilibrium chalcogen concentrations in silicon: Physical structure, electronic transport, and photovoltaic potential", PhD thesis, Harvard University, Cambridge, Massachusetts
- Winkler MT, Recht D, Sher M, Said AJ, Mazur E & Aziz MJ 2011, "Insulator-to-metal transition in sulfur-doped silicon", Physical Review Letters, vol. 106, pp. 178701–4
- Wulfsberg G 1987, Principles of descriptive Inorganic chemistry, Brooks/Cole Publishing Company, Monterey, California ISBN 0-534-07494-4
- Yoder CH, Suydam FH & Snavely FA 1975, Chemistry, 2nd ed, Harcourt Brace Jovanovich, New York, ISBN 978-0-15-506470-6
- Yousuf M 1998, "Diamond anvil cells in high-pressure studies of semiconductors", in T Suski & W Paul (eds), High pressure in semiconductor physics II, Semiconductors and semimetals, vol. 55, Academic Press, San Diego, pp. 382–436, ISBN 9780080864532
- Yu PY & Cardona M 2010, Fundamentals of semiconductors: Physics and materials properties, 4th ed., Springer, Heidelberg, ISBN 9783642007101
- Zumdahl SS & DeCoste DJ 2013, Chemical principles, 7th ed., Brooks/Cole, Belmont, California, ISBN 9781111580650
Monographs
- Emsley J 1971, The inorganic chemistry of the non-metals, Methuen Educational, London, ISBN 0423861204
- Johnson RC 1966, Introductory descriptive chemistry: selected nonmetals, their properties, and behavior, WA Benjamin, New York
- Jolly WL 1966, The chemistry of the non-metals, Prentice-Hall, Englewood Cliffs, New Jersey
- Powell P & Timms PL 1974, The chemistry of the non-metals, Chapman & Hall, London, ISBN 0470695706
- Sherwin E & Weston GJ 1966, Chemistry of the non-metallic elements, Pergamon Press, Oxford
- Steudel R 1977, Chemistry of the non-metals: with an introduction to atomic structure and chemical bonding, English edition by FC Nachod & JJ Zuckerman, Berlin, Walter de Gruyter, ISBN 3110048825
External links
Media related to Nonmetals at Wikimedia Commons