Smart manufacturing
Smart manufacturing is a broad category of manufacturing that employs computer-integrated manufacturing, high levels of adaptability and rapid design changes, digital information technology, and more flexible technical workforce training.[1] Other goals sometimes include fast changes in production levels based on demand,[2] optimization of the supply chain,[2] efficient production and recyclability.[3] In this concept, as smart factory has interoperable systems, multi-scale dynamic modelling and simulation, intelligent automation, strong cyber security, and networked sensors.
History of technology |
---|
By technological eras
|
By type of technology
|
Technology timelines
|
Article indices |
The broad definition of smart manufacturing covers many different technologies. Some of the key technologies in the smart manufacturing movement include big data processing capabilities, industrial connectivity devices and services, and advanced robotics.[4]
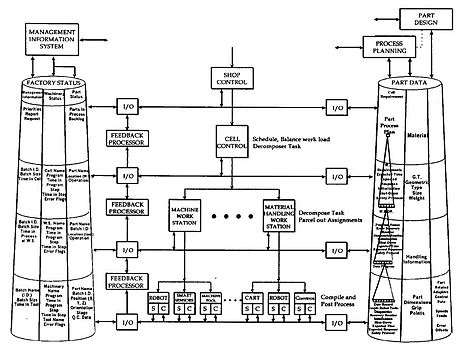
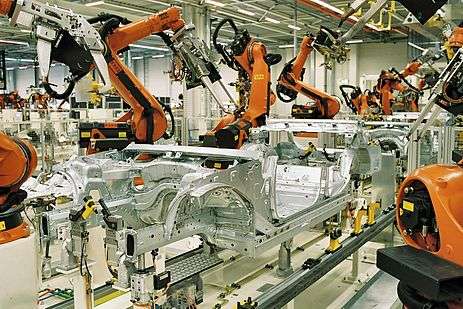
Big data processing
Smart manufacturing utilizes big data analytics, to refine complicated processes and manage supply chains.[6] Big data analytics refers to a method for gathering and understanding large data sets in terms of what are known as the three V's, velocity, variety and volume. Velocity informs the frequency of data acquisition, which can be concurrent with the application of previous data. Variety describes the different types of data that may be handled. Volume represents the amount of data.[7] Big data analytics allows an enterprise to use smart manufacturing to predict demand and the need for design changes rather than reacting to orders placed.[1]
Some products have embedded sensors, which produce large amounts of data that can be used to understand consumer behavior and improve future versions of the product.[8][9][10]
Advanced robotics
Advanced industrial robots, also known as smart machines operate autonomously and can communicate directly with manufacturing systems. In some advanced manufacturing contexts, they can work with humans for co-assembly tasks.[11] By evaluating sensory input and distinguishing between different product configurations, these machines are able to solve problems and make decisions independent of people. These robots are able to complete work beyond what they were initially programmed to do and have artificial intelligence that allows them to learn from experience.[4] These machines have the flexibility to be reconfigured and re-purposed. This gives them the ability to respond rapidly to design changes and innovation, which is a competitive advantage over more traditional manufacturing processes.[12] An area of concern surrounding advanced robotics is the safety and well-being of the human workers who interact with robotic systems. Traditionally, measures have been taken to segregate robots from the human workforce, but advances in robotic cognitive ability have opened up opportunities, such as cobots, for robots to work collaboratively with people.[13]
Industrial connectivity devices and services
Leveraging the capabilities of the Internet, manufacturers are able to increase integration and data storage. Employing cloud software allows companies access to highly configurable computing resources. This allows for servers, networks and other storage applications to be created and released at a rapid pace. Enterprise integration platforms allow the manufacturer to collect data broadcast from its machines, which can track metrics such as work flow and machine history. Open communication between manufacturing devices and networks can also be achieved through Internet connectivity. This encompasses everything from tablets to machine automation sensors and allows for machines to adjust their processes based on input from external devices.[4]
3D printing
As of 2019, 3D printing is mainly used in rapid prototyping, design iteration, and small-scale production. Improvements in speed, quality, and materials could make it useful in mass production[14][15] and mass customization.[15]
Eliminating workplace inefficiencies and hazards
Smart manufacturing can also be attributed to surveying workplace inefficiencies and assisting in worker safety. Efficiency optimization is a huge focus for adopters of "smart" systems, which is done through data research and intelligent learning automation. For instance operators can be given personal access cards with inbuilt Wi-Fi and Bluetooth, which can connect to the machines and a Cloud platform to determine which operator is working on which machine in real time.[16] An intelligent, interconnected 'smart' system can be established to set a performance target, determine if the target is obtainable, and identify inefficiencies through failed or delayed performance targets.[17] In general, automation may alleviate inefficiencies due to human error. And in general, evolving AI eliminates the inefficiencies of its predecessors.
Worker safety can be augmented by safe, innovative design and increasing integrated networks of automation. This is under the notion that Technicians are exposed less to hazardous environments as automation matures. If successful, less human supervision and user instruction for automation will devitalize workplace safety concerns.[18]
Impact of Industry 4.0
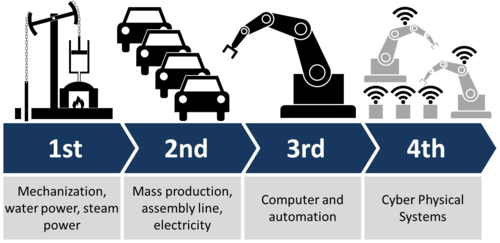
Industry 4.0 is a project in the high-tech strategy of the German government that promotes the computerization of traditional industries such as manufacturing. The goal is the intelligent factory (Smart Factory) that is characterized by adaptability, resource efficiency, and ergonomics, as well as the integration of customers and business partners in business and value processes. Its technological foundation consists of cyber-physical systems and the Internet of Things.[19]
This kind of "intelligent manufacturing" makes a great use of:
- Wireless connections, both during product assembly and long-distance interactions with them;
- Last generation sensors, distributed along the supply chain and the same products (Internet of things)
- Elaboration of a great amount of data to control all phases of construction, distribution and usage of a good.
European Roadmap "Factories of the Future" and German one "Industrie 4.0″ illustrate several of the action lines to undertake and the related benefits. Some examples are:
- Advanced manufacturing processes and rapid prototyping will make possible for each customer to order one-of-a-kind product without significant cost increase.
- Collaborative Virtual Factory (VF) platforms will drastically reduce cost and time associated to new product design and engineering of the production process, by exploiting complete simulation and virtual testing throughout the Product Lifecycle.
- Advanced Human-Machine interaction (HMI) and augmented reality (AR) devices will help increasing safety in production plants and reducing physical demand to workers (whose age has an increasing trend).
- Machine learning will be fundamental to optimize the production processes, both for reducing lead times and reducing the energy consumption.
- Cyber-physical systems and machine-to-machine (M2M) communication will allow to gather and share real-time data from the shop floor in order to reduce down and idle times by conducting extremely effective predictive maintenance.
Smart Manufacturing Paradigms
In this section, the leading visions for the future of smart manufacturing are discussed and for each one, different examples from leading industry and research organizations are presented.
Cyber Physical Systems in Smart Manufacturing
As one of the main elements of Industry 4.0, cyber-physical systems play a significant role in the future of smart manufacturing systems.
- Connection Layer
Systems, comments, machines, and humans are essential parts of manufacturing systems and their contributions highly depend on their connection with the rest of the manufacturing elements. For instance, an operator may require advanced intelligence from the production system in order to make the most efficient decisions for scheduling maintenance or production orders. Advanced communication technologies such as 5G technology [20] would significantly enhance connectivity across manufacturing systems.
- Cyber Layer
This layer is a central hub for data storage where big data analytic tools are utilized for a better and more efficient decision making. A digital twin can be realized in this layer by integrating cyberspace to the physical components through tactile internet. Moreover, similarity-based methods can be used to perform peer to peer comparison across machines and help in better fault diagnosis and enhancing their efficiency.
- Cognition layer
In this layer, infographic tools are utilized to present the results of analytic studies to the users. Simple radar charts and degradation trends can be used for a simple representation of the component's health condition. Then operators can easily make a decision based on the presented data.
- Configuration layer
In this layer, the decisions made in the Cognition layer are applied to the physical system to make the systems self-adapt, self-configure, and self resilient.
Statistics
The Ministry of Economy, Trade and Industry in South Korea announced on 10 March 2016 that it had aided the construction of smart factories in 1,240 small and medium enterprises, which it said resulted in an average 27.6% decrease in defective products, 7.1% faster production of prototypes, and 29.2% lower cost.[21]
Action and effects
From Siemens' perspective,[19] there are three core elements to this evolution:
- Manufacturing execution. Manufacturing execution will play an even more important role. The degree of connectivity between the automation level and the manufacturing execution system (MES) will increase significantly, also across the borders of companies and locations. The integration of Enterprise Resource Planning (ERP) and MES levels will also advance to achieve complete transparency as well as connectivity to business data. That means that all necessary information is available in real time.
- The merging of the product and production life cycle. The second core element is the merging of product and production life cycle based on a common data model. This will allow manufacturers to meet the challenges that result through ever-shorter product life cycles, both technically and in business.
- Cyber-physical systems. Cyber-physical systems are a basis for the increase in manufacturing flexibility that results in shorter time to market. These production units can be flexibly integrated into existing production processes. Cyber physical systems combine communications, IT, data, and physical elements using core technologies, including sensor networks; Internet communication infrastructure; intelligent, real-time processing and event management; big data and data provisioning; embedded software for logic; and automated operations and management of systemic activities across enterprises.
See also
References
- Davis, Jim; Edgar, Thomas; Porter, James; Bernaden, John; Sarli, Michael (2012-12-20). "Smart manufacturing, manufacturing intelligence and demand-dynamic performance". Computers & Chemical Engineering. FOCAPO 2012. 47: 145–156. doi:10.1016/j.compchemeng.2012.06.037.
- SMLC 2011
- Shipp, Stephanie S. (March 2012). "Emerging Global Trends in Advanced Manufacturing" (PDF). Emerging Global Trends in Advanced Manufacturing. Insititute for Defense Analysis. Archived from the original (PDF) on 2012-06-06. Retrieved 2020-04-12.
- "On the Journey to a Smart Manufacturing Revolution". www.industryweek.com. 2015-12-30. Retrieved 2016-02-17.
- 20899, James S. Albus, National Institute of Standards and Technology, Gaithersburg, Maryland (1995-01-01), English: Architecture for the NBS Automated Manufacturing Research Facility (AMRF)., retrieved 2016-03-04CS1 maint: numeric names: authors list (link)
- Rachuri, Dr. Sudarsan (February 4, 2014). "Smart Manufacturing Systems Design and Analysis" (PDF). National Institute of Standards and Technology. Retrieved February 16, 2016.
- Leveling, J.; Edelbrock, M.; Otto, B. (2014-12-01). Big data analytics for supply chain management. 2014 IEEE International Conference on Industrial Engineering and Engineering Management (IEEM). pp. 918–922. doi:10.1109/IEEM.2014.7058772. ISBN 978-1-4799-6410-9.
- Yang, Chen; Shen, Weiming; Wang, Xianbin (January 2018). "The Internet of Things in Manufacturing: Key Issues and Potential Applications". IEEE Systems, Man, and Cybernetics Magazine. 4 (1): 6–15. doi:10.1109/MSMC.2017.2702391.
- Porter, Michael E. (November 2014). "How Smart, Connected Products Are Transforming Competition". Harvard Business Review. April 2016.
- "Building Smarter Manufacturing With The Internet of Things (IoT)". IT World Canada. Lopez Research. 2014. Retrieved 2020-04-12.
- W. Wang, R. Li, Y. Chen, Z. Diekel, and Y. Jia (2019). "Facilitating Human-Robot Collaborative Tasks by Teaching-Learning-Collaboration From Human Demonstrations". IEEE Transactions on Automation Science and Engineering. 16 (2): 640–653. doi:10.1109/tase.2018.2840345. ISSN 1545-5955.CS1 maint: multiple names: authors list (link)
- NIST, US Department of Commerce (October 2013). "Robotic Systems for Smart Manufacturing". www.nist.gov. Retrieved 2016-03-04.
- Bicchi, Antonio; Peshkin, Michael A.; Colgate, J. Edward (2008-01-01). Prof, Bruno Siciliano; Prof, Oussama Khatib (eds.). Safety for Physical Human–Robot Interaction. Springer Berlin Heidelberg. pp. 1335–1348. doi:10.1007/978-3-540-30301-5_58. ISBN 9783540239574.
- "Industry 4.0 – 3D Printing in Manufacturing Industries". Atos. Retrieved 2019-06-09.
- Hughes, Andrew. "Industry 4.0 is About More Than Data: 3D Printing in Manufacturing". blog.lnsresearch.com. Retrieved 2019-06-09.
- "ThingTrax". ThingTrax Connected Manufacturing. London. Archived from the original on 2017-04-12. Retrieved 2020-04-12.
- Jung, Kiwook (2015-03-16). "Mapping Strategic Goals and Operational Performance Metrics for Smart Manufacturing Systems". Procedia Computer Science. 44 (44 p.184–193): 184–193. doi:10.1016/j.procs.2015.03.051.
- Louchez, Alain (January 6, 2014). "From Smart Manufacturing to Manufacturing Smart". www.automationworld.com. Automation World. Retrieved 2016-03-04.
- Jacinto, Joan (July 31, 2014). "Smart Manufacturing? Industry 4.0? What's It All About?". The Vault - Siemens Totally Integrated Automation.
- Andrews, Jeffrey G.; Buzzi, Stefano; Choi, Wan; Hanly, Stephen V.; Lozano, Angel; Soong, Anthony C. K.; Zhang, Jianzhong Charlie (June 2014). "What Will 5G Be?". IEEE Journal on Selected Areas in Communications. 32 (6): 1065–1082. doi:10.1109/JSAC.2014.2328098. hdl:10230/23406.
- Jung Min-hee (March 11, 2016). "Smart Factories Improving Productivity of SMEs".