Seagrass
Seagrasses are flowering plants (angiosperms) which grow in marine environments. There are about 60 species of fully marine seagrasses which belong to four families (Posidoniaceae, Zosteraceae, Hydrocharitaceae and Cymodoceaceae), all in the order Alismatales (in the class of monocotyledons).[1] Seagrasses evolved from terrestrial plants which recolonised the ocean 70 to 100 million years ago.
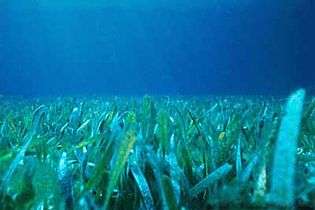
Seagrass
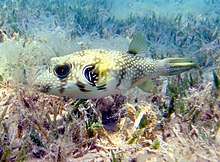
The name seagrass stems from the many species with long and narrow leaves, which grow by rhizome extension and often spread across large "meadows" resembling grassland; many species superficially resemble terrestrial grasses of the family Poaceae.
Like all autotrophic plants, seagrasses photosynthesize, in the submerged photic zone, and most occur in shallow and sheltered coastal waters anchored in sand or mud bottoms. Most species undergo submarine pollination and complete their life cycle underwater.
Seagrasses beds/meadows can be either monospecific (made up of a single species) or in mixed beds. In temperate areas, usually one or a few species dominate (like the eelgrass Zostera marina in the North Atlantic), whereas tropical beds usually are more diverse, with up to thirteen species recorded in the Philippines.
Seagrass beds are diverse and productive ecosystems, and can harbor hundreds of associated species from all phyla, for example juvenile and adult fish, epiphytic and free-living macroalgae and microalgae, mollusks, bristle worms, and nematodes. Few species were originally considered to feed directly on seagrass leaves (partly because of their low nutritional content), but scientific reviews and improved working methods have shown that seagrass herbivory is an important link in the food chain, feeding hundreds of species, including green turtles, dugongs, manatees, fish, geese, swans, sea urchins and crabs. Some fish species that visit/feed on seagrasses raise their young in adjacent mangroves or coral reefs.
Seagrasses trap sediment and slow down water movement, causing suspended sediment to settle out. Trapping sediment benefits coral by reducing sediment loads, improving photosynthesis for both coral and seagrass.[2]
Taxonomy
Family | Image | Genera | Description |
---|---|---|---|
Zosteraceae | The family Zosteraceae, also known as the seagrass family, includes two genera containing 14 marine species. It is found in temperate and subtropical coastal waters, with the highest diversity located around Korea and Japan. Species subtotal: | ||
![]() |
Phyllospadix | 6 species | |
![]() |
Zostera | 16 species | |
Hydrocharitaceae | The family Hydrocharitaceae, also known as tape-grasses, include Canadian waterweed and frogbit. The family includes both fresh and marine aquatics, although of the seventeen species currently recognised only three are marine. [3] They are found throughout the world in a wide variety of habitats, but are primarily tropical. Species subtotal: | ||
![]() |
Enhalus | 1 species | |
![]() |
Halophila | 19 species | |
![]() |
Thalassia | 2 species | |
Posidoniaceae | The family Posidoniaceae contains a single genus with two to nine marine species found in the seas of the Mediterranean and around the south coast of Australia. Species subtotal: 2 to 9 | ||
![]() |
Posidonia | 2 to 9 species | |
Cymodoceaceae | The family Cymodoceaceae, also known as manatee-grass, includes only marine species.[4] Some taxonomists do not recognized this family. Species subtotal: | ||
![]() |
Amphibolis | 2 species | |
Cymodocea | 4 species | ||
![]() |
Halodule | 6 species | |
![]() |
Syringodium | 2 species | |
![]() |
Thalassodendron | 3 species | |
Total species: |
Evolution
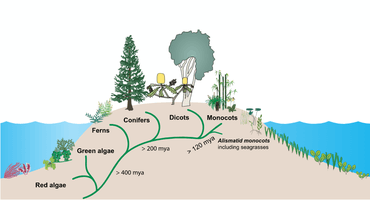
Terrestrial plants evolved perhaps as early as 850 million years ago from a group of green algae.[5] Seagrasses then evolved from terrestrial plants which migrated back into the ocean.[6][7] Between about 70 million and 100 million years ago, the three independent seagrass lineages (Hydrocharitaceae, Cymodoceaceae complex, and Zosteraceae) evolved from a single lineage of monocotyledonous flowering plants.[8]
Other plants that colonised the sea, such as salt marsh plants, mangroves, and marine algae, have more diverse evolutionary lineages. In spite of their low species diversity, seagrasses have succeeded in colonising the continental shelves of all continents except Antarctica.[9]
Role of microorganisms
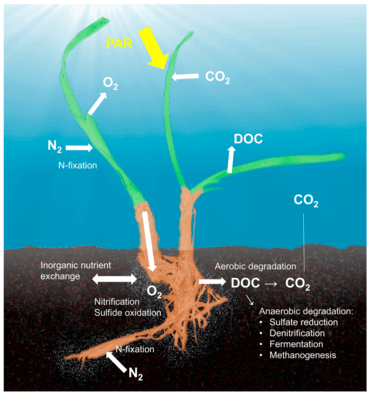
The seagrass microbiome
The seagrass holobiont
The concept of the holobiont,[12] which emphasizes the importance and interactions of a microbial host with associated microorganisms and viruses and describes their functioning as a single biological unit, has been investigated and discussed for many model systems, although there is substantial criticism on a concept that defines diverse host-microbe symbioses as a single biological unit.[13] The holobiont and hologenome concepts have evolved since the original definition [14] and there is no doubt that symbiotic microorganisms are pivotal for the biology and ecology of the host by providing vitamins, energy and inorganic or organic nutrients, participating in defense mechanisms, or by driving the evolution of the host.[15] Although most work on host-microbe interactions has been focused on animal systems such as corals, sponges, or humans, there is a substantial body of literature on plant holobionts.[16] Plant-associated microbial communities impact both key components of the fitness of plants, growth and survival,[17] and are shaped by nutrient availability and plant defense mechanisms.[18] Several habitats have been described to harbor plant-associated microbes, including the rhizoplane (surface of root tissue), the rhizosphere (periphery of the roots), the endosphere (inside plant tissue), and the phyllosphere (total above-ground surface area).[10]
Ecosystem services
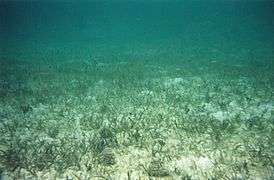
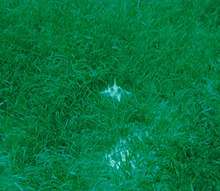
Although often overlooked, seagrasses provide coastal zones with a number of ecosystem goods and services. Seagrasses are considered ecosystem engineers.[19][7][6] This means that the plants alter the ecosystem around them. This adjusting occurs in both physical and chemical forms. Many seagrass species produce an extensive underground network of roots and rhizome which stabilizes sediment and reduces coastal erosion.[20] This system also assists in oxygenating the sediment, providing a hospitable environment for sediment-dwelling organisms.[19] Seagrasses also enhance water quality by stabilizing heavy metals, pollutants, and excess nutrients.[21][7][6] The long blades of seagrasses slow the movement of water which reduces wave energy and offers further protection against coastal erosion and storm surge. Furthermore, because seagrasses are underwater plants, they produce significant amounts of oxygen which oxygenate the water column. These meadows account for more than 10% of the ocean's total carbon storage. Per hectare, it holds twice as much carbon dioxide as rain forests and can sequester about 27.4 million tons of CO2 annually.[22] The storage of carbon is an essential ecosystem service as we move into a period of elevated atmospheric carbon levels. However, some climate change models suggest that some seagrasses will go extinct – Posidonia oceanica is expected to go extinct, or nearly so, by 2050.
Seagrass meadows also provide physical habitat in areas that would otherwise be bare of any vegetation. Due to this three dimensional structure in the water column, many species occupy seagrass habitats for shelter and foraging. It is estimated that 17 species of coral reef fish spend their entire juvenile life stage solely on seagrass flats.[23] These habitats also act as a nursery grounds for commercially and recreationally valued fishery species, including the gag grouper (Mycteroperca microlepis), red drum, common snook, and many others.[24][25] Some fish species utilize seagrass meadows and various stages of the life cycle. In a recent publication, Dr. Ross Boucek and colleagues discovered that two highly sought after flats fish, the common snook and spotted sea trout provide essential foraging habitat during reproduction.[26] Sexual reproduction is extremely energetically expensive to be completed with stored energy; therefore, they require seagrass meadows in close proximity to complete reproduction.[26] Furthermore, many commercially important invertebrates also reside in seagrass habitats including bay scallops (Argopecten irradians), horseshoe crabs, and shrimp. Charismatic fauna can also be seen visiting the seagrass habitats. These species include West Indian manatee, green sea turtles, and various species of sharks. The high diversity of marine organisms that can be found on seagrass habitats promotes them as a tourist attraction and a significant source of income for many coastal economies along the Gulf of Mexico and in the Caribbean.
Relation to humans
Historically, seagrasses were collected as fertilizer for sandy soil. This was an important use in the Aveiro Lagoon, Portugal, where the plants collected were known as moliço.
In the early 20th century, in France and, to a lesser extent, the Channel Islands, dried seagrasses were used as a mattress (paillasse) filling - such mattresses were in high demand by French forces during World War I. It was also used for bandages and other purposes.
In February 2017, researchers found that seagrass meadows may be able to remove various pathogens from seawater. On small islands without wastewater treatment facilities in central Indonesia, levels of pathogenic marine bacteria – such as Enterococcus – that affect humans, fish and invertebrates were reduced by 50 percent when seagrass meadows were present, compared to paired sites without seagrass,[27] although this could be a detriment to their survival.[28]
Disturbances and threats
Natural disturbances, such as grazing, storms, ice-scouring and desiccation, are an inherent part of seagrass ecosystem dynamics. Seagrasses display a high degree of phenotypic plasticity, adapting rapidly to changing environmental conditions.
Seagrasses are in global decline, with some 30,000 km2 (12,000 sq mi) lost during recent decades. The main cause is human disturbance, most notably eutrophication, mechanical destruction of habitat, and overfishing. Excessive input of nutrients (nitrogen, phosphorus) is directly toxic to seagrasses, but most importantly, it stimulates the growth of epiphytic and free-floating macro- and micro-algae. This weakens the sunlight, reducing the photosynthesis that nourishes the seagrass and the primary production results.
Decaying seagrass leaves and algae fuels increasing algal blooms, resulting in a positive feedback. This can cause a complete regime shift from seagrass to algal dominance. Accumulating evidence also suggests that overfishing of top predators (large predatory fish) could indirectly increase algal growth by reducing grazing control performed by mesograzers, such as crustaceans and gastropods, through a trophic cascade.
Macroalgal blooms cause the decline and eradication of seagrasses. Known as nuisance species, macroalgae grow in filamentous and sheet-like forms and form thick unattached mats over seagrass, occurring as epiphytes on seagrass leaves. Eutrophication leads to the forming of a bloom, causing the attenuation of light in the water column, which eventually leads to anoxic conditions for the seagrass and organisms living in/around the plant(s). In addition to the direct blockage of light to the plant, benthic macroalgae have low carbon/nitrogen content, causing their decomposition to stimulate bacterial activity, leading to sediment resuspension, an increase in water turbidity and further light attenuation.[29][30]
When humans drive motor boats over shallow seagrass areas, sometimes the propeller blade can damage the seagrass.
The most-used methods to protect and restore seagrass meadows include nutrient and pollution reduction, marine protected areas and restoration using seagrass transplanting. Seagrass is not seen as resilient to the impacts of future environmental change.[31]
Restoration
In various locations, communities are attempting to restore seagrass beds that were lost to human action, including in the US states of Virginia,[32] Florida[33] and Hawaii,[34] as well as the United Kingdom.[35] Such reintroductions have been shown to improve ecosystem services.[36]
As of 2019 the Coastal Marine Ecosystems Research Centre of Central Queensland University has been growing seagrass for six years and has been producing seagrass seeds. They have been running trials in germination and sowing techniques.[37]
See also
References
- Tomlinson and Vargo (1966). "On the morphology and anatomy of turtle grass, Thalassia testudinum (Hydrocharitaceae). I. Vegetative Morphology". Bulletin of Marine Science. 16: 748–761.
- Seagrass-Watch: What is seagrass? Retrieved 2012-11-16.
- Waycott, Michelle; McMahon, Kathryn; Lavery, Paul (2014). A Guide to Southern Temperate Seagrasses. CSIRO Publishing. ISBN 9781486300150.
- Waycott, Michelle; McMahon, Kathryn; Lavery, Paul (2014). A Guide to Southern Temperate Seagrasses. CSIRO Publishing. ISBN 9781486300150.
- Knauth, L. Paul; Kennedy, Martin J. (2009). "The late Precambrian greening of the Earth". Nature. 460 (7256): 728–732. Bibcode:2009Natur.460..728K. doi:10.1038/nature08213. PMID 19587681.
- Orth; et al. (2006). "A global crisis for seagrass ecosystems". BioScience. 56 (12): 987–996. doi:10.1641/0006-3568(2006)56[987:AGCFSE]2.0.CO;2. hdl:10261/88476.
- Papenbrock, J (2012). "Highlights in seagrass' phylogeny, physiology, and metabolism: what makes them so species?". International Scholarly Research Network: 1–15.
- Les, D.H., Cleland, M.A. and Waycott, M. (1997) "Phylogenetic studies in Alismatidae, II: evolution of marine angiosperms (seagrasses) and hydrophily". Systematic Botany 22(3): 443–463.
- Orth, R.J., Carruthers, T.J., Dennison, W.C., Duarte, C.M., Fourqurean, J.W., Heck, K.L., Hughes, A.R., Kendrick, G.A., Kenworthy, W.J., Olyarnik, S. and Short, F.T., 2006. A global crisis for seagrass ecosystems. Bioscience, 56(12), pp.987-996. doi:10.1641/0006-3568(2006)56[987:AGCFSE]2.0.CO;2.
- Ugarelli, K., Chakrabarti, S., Laas, P. and Stingl, U. (2017) "The seagrass holobiont and its microbiome". Microorganisms, 5(4): 81. doi:10.3390/microorganisms5040081.
Material was copied from this source, which is available under a Creative Commons Attribution 4.0 International License. - Tarquinio, F., Hyndes, G.A., Laverock, B., Koenders, A. and Säwström, C. (2019) "The seagrass holobiont: understanding seagrass-bacteria interactions and their role in seagrass ecosystem functioning". FEMS microbiology letters, 366(6): fnz057. doi:10.1093/femsle/fnz057.
- Margulis, Lynn (1991) "Symbiogenesis and Symbionticism". In: Symbiosis as a Source of Evolutionary Innovation; Margulis, L., Fester, R.(Eds.), Cambridge MIT Press. ISBN 9780262132695.
- Douglas, A.E.; Werren, J.H. (2016) "Holes in the Hologenome: Why Host-Microbe Symbioses Are Not Holobionts". mBio, 7: e02099-15. doi:10.1128/mBio.02099-15.
- Theis, K.R.; Dheilly, N.M.; Klassen, J.L.; Brucker, R.M.; Baines, J.F.; Bosch, T.C.G.; Cryan, J.F.; Gilbert, S.F.; Goodnight, C.J.; Lloyd, E.A.; et al. Getting the Hologenome Concept Right: An Eco-Evolutionary Framework for Hosts and Their Microbiomes. mSystems 2016, 1, e00028-16. doi:10.1128/mSystems.00028-16.
- Rosenberg, E. and Zilber-Rosenberg, I. (2016) "Microbes drive evolution of animals and plants: the hologenome concept". MBio, 7(2). doi:10.1128/mBio.01395-15.
- Zilber-Rosenberg, I. and Rosenberg, E. (2008) "Role of microorganisms in the evolution of animals and plants: the hologenome theory of evolution". FEMS Microbiology Reviews, 32(5): 723–735. doi:10.1111/j.1574-6976.2008.00123.x.
- Vandenkoornhuyse, P., Quaiser, A., Duhamel, M., Le Van, A. and Dufresne, A. (2015) "The importance of the microbiome of the plant holobiont". New Phytologist, 206(4): 1196-1206. doi:10.1111/nph.13312.
- Sánchez-Cañizares, C., Jorrín, B., Poole, P.S. and Tkacz, A. (2017) "Understanding the holobiont: the interdependence of plants and their microbiome". Current Opinion in Microbiology, 38: 188–196. doi:10.1016/j.mib.2017.07.001.
- Jones, Clive G.; Lawton, John H.; Shachak, Moshe (1994). "Organisms as ecosystem engineers". Oikos. 69 (3): 373–386. doi:10.2307/3545850. JSTOR 3545850.
- Grey, William; Moffler, Mark (1987). "Flowering of the seagrass Thalassia testudinum (Hydrocharitacea) in the Tampa Bay, Florida area". Aquatic Botany. 5: 251–259. doi:10.1016/0304-3770(78)90068-2.
- Darnell, Kelly; Dunton, Kenneth (2016). "Reproductive phenology of the subtropical seagrasses Thalassia testudinum (Turtle grass) and Halodule wrightii (Shoal grass) in the northwest Gulf of Mexico". Botanica Marina. 59 (6): 473–483. doi:10.1515/bot-2016-0080.
- Macreadie, P. I.; Baird, M. E.; Trevathan-Tackett, S. M.; Larkum, A. W. D.; Ralph, P. J. (2013). "Quantifying and modelling the carbon sequestration capacity of seagrass meadows". Marine Pollution Bulletin. 83 (2): 430–439. doi:10.1016/j.marpolbul.2013.07.038. PMID 23948090.
- Nagelkerken, I.; Roberts, C. M.; van der Velde, G.; Dorenbosch, M.; van Riel, M. C.; Cocheret de la Morinière, E.; Nienhuis, P. H. (2002). "How important are mangroves and seagrass beds for coral-reef fish? The nursery hypothesis tested on an island scale". Marine Ecology Progress Series. 244: 299–305. Bibcode:2002MEPS..244..299N. doi:10.3354/meps244299.
- Nordlund, L. M.; Unsworth, R. K. F.; Gullstrom, M.; Cullen-Unsworth, L. C. (2018). "Global significance of seagrass fishery activity". Fish and Fisheries. 19 (3): 399–412. doi:10.1111/faf.12259.
- Unsworth, R. K. F.; Nordlund, L. M.; Cullen-Unsworth, L. C. (2019). "Seagrass meadows support global fisheries production". Conserv Lett. e12566: e12566. doi:10.1111/conl.12566.
- Boucek, R. E.; Leone, E.; Bickford, J.; Walters-Burnsed, S.; Lowerre-Barbieri, S. (2017). "More than just a spawning location: Examining fine-scale s[ace use of two estuarine fish species at a spawning aggregation site". Frontiers in Marine Science (4): 1–9.
- Byington, Cara (2017-02-17). "New Science Shows Seagrass Meadows Suppress Pathogens". Nature.org. NatureNet Fellows for Cool Green Science. Retrieved 17 February 2017.
- Jones, BJ; Cullen-Unsworth, L. C.; Unsworth, R. K. F. (2018). "Tracking Nitrogen Source Using δ15N Reveals Human and Agricultural Drivers of Seagrass Degradation across the British Isles". Frontiers in Plant Science. 9: 133. doi:10.3389/fpls.2018.00133. PMC 5808166. PMID 29467789.
- McGlathery, KJ (2001). "Macroalgal blooms contribute to the decline of seagrass in nutrient‐enriched coastal waters" (PDF). Journal of Phycology. 37 (4): 453–456. doi:10.1046/j.1529-8817.2001.037004453.x.
- Fox SE, YS Olsen and AC Spivak (2010) "Effects of bottom-up and top-down controls and climate change on estuarine macrophyte communities and the ecosystem services they provide" In: PF Kemp (Ed) Eco-DAS Symposium Proceedings, ALSO, Chapter 8: 129–145.
- Unsworth, Richard K.F.; Collier, Catherine J.; Waycott, Michelle; McKenzie, Len J.; Cullen-Unsworth, Leanne C. (2015). "A framework for the resilience of seagrass ecosystems". Marine Pollution Bulletin. 100 (1): 34–46. doi:10.1016/j.marpolbul.2015.08.016. PMID 26342389.
- "Eelgrass Restoration | The Nature Conservancy in Virginia". www.nature.org. Retrieved 2018-08-06.
- "Seagrass Restoration". myfwc.com. Retrieved 2018-08-06.
- "Seagrass Restoration Initiative – Malama Maunalua". www.malamamaunalua.org. Retrieved 2018-08-06.
- Unsworth, Richard K. F.; McKenzie, Len J.; Collier, Catherine J.; Cullen-Unsworth, Leanne C.; Duarte, Carlos M.; Eklöf, Johan S.; Jarvis, Jessie C.; Jones, Benjamin L.; Nordlund, Lina M. (2019-08-01). "Global challenges for seagrass conservation". Ambio. 48 (8): 801–815. doi:10.1007/s13280-018-1115-y. ISSN 1654-7209. PMC 6541581. PMID 30456457.
- van Katwijk, Marieke M.; Thorhaug, Anitra; Marbà, Núria; Orth, Robert J.; Duarte, Carlos M.; Kendrick, Gary A.; Althuizen, Inge H. J.; Balestri, Elena; Bernard, Guillaume (2015-11-25). "Global analysis of seagrass restoration: the importance of large-scale planting". Journal of Applied Ecology. 53 (2): 567–578. doi:10.1111/1365-2664.12562. ISSN 0021-8901.
- Mackay, Jacquie; Stünzner, Inga (24 October 2019). "Seagrass nursery in central Queensland could offset carbon emissions". ABC News. Australian Broadcasting Corporation. Retrieved 24 October 2019.
One flower can produce 15 seeds, and one seed planted in the right conditions can create a hectare of seagrass.
Further references
- den Hartog, C. 1970. The Sea-grasses of the World. Verhandl. der Koninklijke Nederlandse Akademie van Wetenschappen, Afd. Natuurkunde, No. 59(1).
- Duarte, Carlos M. and Carina L. Chiscano “Seagrass biomass and production: a reassessment” Aquatic Botany Volume 65, Issues 1–4, November 1999, Pages 159–174.
- Green, E.P. & Short, F.T.(eds). 2003. World Atlas of Seagrasses. University of California Press, Berkeley, CA. 298 pp.
- Hemminga, M.A. & Duarte, C. 2000. Seagrass Ecology. Cambridge University Press, Cambridge. 298 pp.
- Hogarth, Peter The Biology of Mangroves and Seagrasses (Oxford University Press, 2007)
- Larkum, Anthony W.D., Robert J. Orth, and Carlos M. Duarte (Editors) Seagrasses: Biology, Ecology and Conservation (Springer, 2006)
- Orth, Robert J. et al. "A Global Crisis for Seagrass Ecosystems" BioScience December 2006 / Vol. 56 No. 12, Pages 987–996.
- Short, F.T. & Coles, R.G.(eds). 2001. Global Seagrass Research Methods. Elsevier Science, Amsterdam. 473 pp.
- A.W.D. Larkum, R.J. Orth, and C.M. Duarte (eds). Seagrass Biology: A Treatise. CRC Press, Boca Raton, FL, in press.
- A. Schwartz; M. Morrison; I. Hawes; J. Halliday. 2006. Physical and biological characteristics of a rare marine habitat: sub-tidal seagrass beds of offshore islands. Science for Conservation 269. 39 pp.
- Waycott, M, McMahon, K, & Lavery, P 2014, A guide to southern temperate seagrasses, CSIRO Publishing, Melbourne
External links
- Cullen-Unsworth, Leanne C.; Unsworth, Richard (2018-08-03). "A call for seagrass protection". Science. 361 (6401): 446–448. Bibcode:2018Sci...361..446C. doi:10.1126/science.aat7318 (inactive 2020-03-20). ISSN 0036-8075. PMID 30072524.
- Project Seagrass - Charity advancing the conservation of seagrass through education, influence, research and action
- SeagrassSpotter - Citizen Science project raising awaress for seagrass meadows and mapping their locations
- Seagrass and Seagrass Beds overview from the Smithsonian Ocean Portal
- Nature Geoscience article describing the locations of the seagrass meadows around the world
- Seagrass-Watch - the largest scientific, non-destructive, seagrass assessment and monitoring program in the world
- Seagrass Ecosystem Research Group at Swansea University - Inter-disciplinary marine research for conservation
- Restore-A-Scar - a non-profit campaign to restore seagrass meadows damaged by boat props
- SeagrassNet - global seagrass monitoring program
- The Seagrass Fund at The Ocean Foundation
- Taxonomy of seagrasses
- World Seagrass Association
- SeagrassLI
- Seagrass Science and Management in the South China Sea and Gulf of Thailand
- Marine Ecology (December 2006) - special issue on seagrasses
- Cambodian Seagrasses
- Seagrass Productivity - COST Action ES0906
- Fisheries Western Australia - Seagrass Fact Sheet