RNA-induced silencing complex
The RNA-induced silencing complex, or RISC, is a multiprotein complex, specifically a ribonucleoprotein, which incorporates one strand of a single-stranded RNA (ssRNA) fragment, such as microRNA (miRNA), or double-stranded small interfering RNA (siRNA).[1] The single strand acts as a template for RISC to recognize complementary messenger RNA (mRNA) transcript. Once found, one of the proteins in RISC, called Argonaute, activates and cleaves the mRNA. This process is called RNA interference (RNAi) and it is found in many eukaryotes; it is a key process in gene silencing and defense against viral infections.[2][3]
Discovery
The biochemical identification of RISC was conducted by Gregory Hannon and his colleagues at the Cold Spring Harbor Laboratory.[4] This was only a couple of years after the discovery of RNA interference in 1998 by Andrew Fire and Craig Mello, who shared the 2006 Nobel Prize in Physiology or Medicine.[2]
.jpg)
Hannon and his colleagues attempted to identify the RNAi mechanisms involved in gene silencing, by dsRNAs, in Drosophila cells. Drosophila S2 cells were transfected with a lacZ expression vector to quantify gene expression with β-galactosidase activity. Their results showed co-transfection with lacZ dsRNA significantly reduced β-galactosidase activity compared to control dsRNA. Therefore, dsRNAs control gene expression via sequence complementarity.
S2 cells were then transfected with Drosophila cyclin E dsRNA. Cycline E is an essential gene for cell cycle progression into the S phase. Cyclin E dsRNA arrested the cell cycle at the G1 phase (before the S phase). Therefore, RNAi can target endogenous genes.
In addition, cyclin E dsRNA only diminished cyclin E RNA — a similar result was also shown using dsRNA corresponding to cyclin A which acts in S, G2 and M phases of the cell cycle. This shows the characteristic hallmark of RNAi: the reduced levels of mRNAs correspond to the levels of dsRNA added.
To test whether their observation of decreased mRNA levels was a result of mRNA being targeted directly (as suggested by data from other systems), Drosophila S2 cells were transfected with either Drosophila cyclin E dsRNAs or lacZ dsRNAs and then incubated with synthetic mRNAs for cyclin E or lacZ.
Cells transfected with cyclin E dsRNAs only showed degradation in cyclin E transcripts — the lacZ transcripts were stable. Conversely, cells transfected with lacZ dsRNAs only showed degradation in lacZ transcripts and not cyclin E transcripts. Their results led Hannon and his colleagues to suggest RNAi degrades target mRNA through a 'sequence-specific nuclease activity'. They termed the nuclease enzyme RISC.[4]
Function in RNA interference
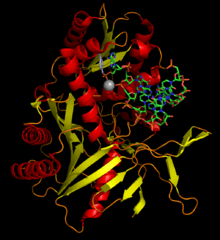
Loading of dsRNA
The RNase III Dicer aids RISC in RNA interference by cleaving dsRNA into 21-23 nucleotide long fragments with a two-nucleotide 3' overhang.[5][6] These dsRNA fragments are loaded into RISC and each strand has a different fate based on the asymmetry rule phenomenon.[7][8][9]
- The strand with the less stable 5' end is selected by the protein Argonaute and integrated into RISC.[9][10] This strand is known as the guide strand.
- The other strand, known as the passenger strand, is degraded by RISC.[11]
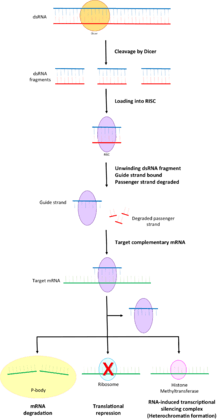
Gene regulation
RISC uses the bound guide strand to target complementary 3'-untranslated regions (3'UTR) of mRNA transcripts via Watson-Crick base pairing.[12][13] RISC can now regulate gene expression of the mRNA transcript in a number of ways.
mRNA degradation
The most understood function of RISC is degrading target mRNA which reduces the levels of transcript available to be translated by ribosomes. There are two main requirements for mRNA degradation to take place:
- a near-perfect complementary match between the guide strand and target mRNA sequence, and,
- a catalytically active Argonaute protein, called a 'slicer', to cleave the target mRNA.[13]
mRNA degradation is localised in cytoplasmic bodies called P-bodies.[14]
Translational repression
RISC can modulate the loading of ribosome and accessory factors in translation to repress expression of the bound mRNA transcript. Translational repression only requires a partial sequence match between the guide strand and target mRNA.[13]
Translation can be regulated at the initiation step by:
- preventing the binding of the eukaryotic translation initiation factor (eIF) to the 5' cap. It has been noted RISC can deadenylate the 3' poly(A) tail which might contribute to repression via the 5' cap.[1][12]
- preventing the binding of the 60S ribosomal subunit binding to the mRNA can repress translation.[15]
Translation can be regulated at post-initiation steps by:
There is still speculation on whether translational repression via initiation and post-initiation is mutually exclusive.
Heterochromatin formation
Some RISCs are able to directly target the genome by recruiting histone methyltransferases to form heterochromatin at the gene locus and thereby, silencing the gene. These RISCs take the form of a RNA-induced transcriptional silencing complex (RITS). The best studied example is with the yeast RITS.[13][18][19]
The mechanism is not well understood but RITS degrade nascent mRNA transcripts. It has been suggested this mechanism acts as a 'self-reinforcing feedback loop' as the degraded nascent transcripts are used by RNA-dependent RNA polymerase (RdRp) to generate more siRNAs.[20]
DNA elimination
RISCs seem to have a role in degrading DNA during somatic macronucleus development in protozoa Tetrahymena. It is similar to heterochromatin formation and is implied as a defence against invading genetic elements.[21]
RISC-associated proteins
The complete structure of RISC is still unsolved. Many studies have reported a range of sizes and components for RISC but it is not entirely sure whether this is due to there being a number of RISC complexes or due to the different sources that different studies use.[22]
Complex | Source | Known/apparent components | Estimated size | Apparent function in RNAi pathway |
---|---|---|---|---|
Dcr2-R2D2[23] | D. melanogaster S2 cells | Dcr2, R2D2 | ~250 kDa | dsRNA processing, siRNA binding |
RLC (A)[24][25] | D. melanogaster embryos | Dcr2, R2D2 | NR | dsRNA processing, siRNA binding, precursor to RISC |
Holo-RISC[24][25] | D. melanogaster embryos | Ago 2, Dcr1, Dcr2, Fmr1/Fxr, R2D2, Tsn, Vig | ~80S | Target-RNA binding and cleavage |
RISC[4][26][27][28] | D. melanogaster S2 cells | Ago2, Fmr1/Fxr, Tsn, Vig | ~500 kDa | Target-RNA binding and cleavage |
RISC[29] | D. melanogaster S2 cells | Ago2 | ~140 kDa | Target-RNA binding and cleavage |
Fmr1-associated complex[30] | D. melanogaster S2 cells | L5, L11, 5S rRNA, Fmr1/Fxr, Ago2, Dmp68 | NR | Possible target-RNA binding and cleavage |
Minimal RISC[31][32][33][34] | HeLa cells | eIF2C1 (Ago1) or eIF2C2 (Ago2) | ~160 kDa | Target-RNA binding and cleavage |
miRNP[35][36] | HeLa cells | eIF2C2 (ago2), Gemin3, Gemin4 | ~550 kDa | miRNA association, target-RNA binding and cleavage |
Ago, Argonaute; Dcr, Dicer; Dmp68, D. melanogaster orthologue of mammalian p68 RNA unwindase; eIF2C1, eukaryotic translation initiation factor 2C1; eIF2C2, eukaryotic translation initiation factor 2C2; Fmr1/Fxr, D. melanogaster orthologue of the fragile-X mental retardation protein; miRNP, miRNA-protein complex; NR, not reported; Tsn, Tudor-staphylococcal nuclease; Vig, vasa intronic gene.
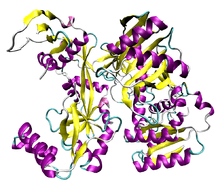
Regardless, it is apparent that Argonaute proteins are present and are essential for function. Furthermore, there are insights into some of the key proteins (in addition to Argonaute) within the complex, which allow RISC to carry out its function.
Argonaute proteins
Argonaute proteins are a family of proteins found in prokaryotes and eukaryotes. Their function in prokaryotes is unknown but in eukaryotes they are responsible for RNAi.[37] There are eight family members in human Argonautes of which only Argonaute 2 is exclusively involved in targeted RNA cleavage in RISC.[34]
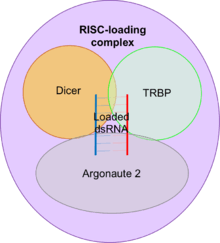
RISC-loading complex
The RISC-loading complex (RLC) is the essential structure required to load dsRNA fragments into RISC in order to target mRNA. The RLC consists of dicer, the transactivating response RNA-binding protein (TRBP) and Argonaute 2.
- Dicer is an RNase III endonuclease which generates the dsRNA fragments to be loaded that direct RNAi.
- TRBP is a protein with three double-stranded RNA-binding domains.
- Argonaute 2 is an RNase and is the catalytic centre of RISC.
Dicer associates with TRBP and Argonaute 2 to facilitate the transfer of the dsRNA fragments generated by Dicer to Argonaute 2.[38][39]
More recent research has shown the human RNA helicase A could help facilitate the RLC.[40]
Other proteins
Recently identified members of RISC are SND1 and MTDH.[41] SND1 and MTDH are oncogenes and regulate various gene expression.[42]
Protein | Species the protein is found |
---|---|
Dcr1[24] | D. melanogaster |
Dcr2[23][24][25] | D. melanogaster |
R2D2[24][25] | D. melanogaster |
Ago2[24][26][29][30] | D. melanogaster |
Dmp68[30] | D. melanogaster |
Fmr1/Fxr[24][27][30] | D. melanogaster |
Tsn[24][28] | D. melanogaster |
Vig[24][27] | D. melanogaster |
Polyribosomes, ribosome components[4][24][26][30][43] | D. melanogaster, T. brucei |
eIF2C1 (Ago1)[31] | H. sapiens |
eIF2C2 (Ago2)[31][32][34][36] | H. sapiens |
Gemin3[35][36] | H. sapiens |
Gemin4[35][36] | H. sapiens |
Ago, Argonaute; Dcr, Dicer; Dmp68, D. melanogaster orthologue of mammalian p68 RNA unwindase; eIF2C1, eukaryotic translation initiation factor 2C1; eIF2C2, eukaryotic translation initiation factor 2C2; Fmr1/Fxr, D. melanogaster orthologue of the fragile-X mental retardation protein; Tsn, Tudor-staphylococcal nuclease; Vig, vasa intronic gene.
Binding of mRNA
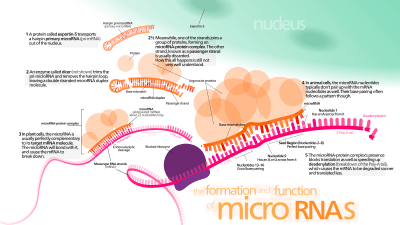
It is as yet unclear how the activated RISC complex locates the mRNA targets in the cell, though it has been shown that the process can occur in situations outside of ongoing protein translation from mRNA.[44]
Endogenously expressed miRNA in metazoans is usually not perfectly complementary to a large number of genes and thus, they modulate expression via translational repression.[45][46] However, in plants, the process has a much greater specificity to target mRNA and usually each miRNA only binds to one mRNA. A greater specificity means mRNA degradation is more likely to occur.[47]
See also
References
- Filipowicz W, Bhattacharyya SN, Sonenber N (2008). "Mechanisms of post-transcriptional regulation by microRNAs: are the answers in sight?". Nature Reviews Genetics. 9 (2): 102–114. doi:10.1038/nrg2290. PMID 18197166.
- Fire A, Xu S, Montgomery MK, Kostas SA, Driver SE, Mello CC (1998). "Potent and specific genetic interference by double-stranded RNA in Caenorhabditis elegans". Nature. 391 (6669): 806–811. doi:10.1038/35888. PMID 9486653.
- Watson, James D. (2008). Molecular Biology of the Gene. San Francisco, CA: Cold Spring Harbor Laboratory Press. pp. 641–648. ISBN 978-0-8053-9592-1.
- Hammond SM, Bernstein E, Beach D, Hannon GJ (2000). "An RNA-directed nuclease mediates post-transcriptional gene silencing in Drosophila cells". Nature. 404 (6775): 293–296. doi:10.1038/35005107. PMID 10749213.
- Zamore PD, Tuschl T, Sharp PA, Bartel DP (2000). "RNAi: double-stranded RNA directs the ATP-dependent cleavage of mRNA at 21 to 23 nucleotide intervals". Cell. 101 (1): 25–33. doi:10.1016/S0092-8674(00)80620-0. PMID 10778853.
- Vermeulen A, Behlen L, Reynolds A, Wolfson A, Marshall W, Karpilow J, Khvorova A (2005). "The contributions of dsRNA structure to Dicer specificity and efficiency". RNA. 11 (5): 674–682. doi:10.1261/rna.7272305. PMC 1370754. PMID 15811921.
- Schwarz DS, Hutvágner G, Du T, Xu Z, Aronin N, Zamore PD (2003). "Asymmetry in the assembly of the RNAi enzyme complex". Cell. 115 (2): 199–208. doi:10.1016/S0092-8674(03)00759-1. PMID 14567917.
- Khvorova A, Reynolds A, Jayasena SD (2003). "Functional siRNAs and miRNAs exhibit strand bias". Cell. 115 (2): 209–216. doi:10.1016/S0092-8674(03)00801-8. PMID 14567918.
- Siomi H, Siomi MC (2009). "On the road to reading the RNA-interference code". Nature. 457 (7228): 396–404. doi:10.1038/nature07754. PMID 19158785.
- Preall JB, He Z, Gorra JM, Sontheimer EJ (2006). "Short interfering RNA strand selection is independent of dsRNA processing polarity during RNAi in Drosophila". Current Biology. 16 (5): 530–535. doi:10.1016/j.cub.2006.01.061. PMID 16527750.
- Gregory RI, Chendrimada TP, Cooch N, Shiekhattar R (2005). "Human RISC couples microRNA biogenesis and posttranscriptional gene silencing". Cell. 123 (4): 631–640. doi:10.1016/j.cell.2005.10.022. PMID 16271387.
- Wakiyama M, Takimoto K, Ohara O, Yokoyama S (2007). "Let-7 microRNA-mediated mRNA deadenylation and translational repression in a mammalian cell-free system". Genes & Development. 21 (15): 1857–1862. doi:10.1101/gad.1566707. PMC 1935024. PMID 17671087.
- Pratt AJ, MacRae IJ (2009). "The RNA-induced silencing complex: A versatile gene-silencing machine". Journal of Biological Chemistry. 284 (27): 17897–17901. doi:10.1074/jbc.R900012200. PMC 2709356. PMID 19342379.
- Sen GL, Blau HM (2005). "Argonaute2/RISC resides in sites of mammalian mRNA decay known as cytoplasmic bodies". Nature Cell Biology. 7 (6): 633–636. doi:10.1038/ncb1265. PMID 15908945.
- Chendrimada TP, Finn KJ, Ji X, Baillat D, Gregory RI, Liebhaber SA, Pasquinelli AE, Shiekhattar R (2007). "MicroRNA silencing through RISC recruitment of eIF6". Nature. 447 (7146): 823–828. doi:10.1038/nature05841.
- Petersen CP, Bordeleau ME, Pelletier J, Sharp PA (2006). "Short RNAs repress translation after initiation in mammalian cells". Molecular Cell. 21 (4): 533–542. doi:10.1016/j.molcel.2006.01.031. PMID 16483934.
- Maroney PA, Yu Y, Fisher J, Nilsen TW (2006). "Evidence that microRNAs are associated with translating messenger RNAs in human cells". Nature Structural & Molecular Biology. 13 (12): 1102–1107. doi:10.1038/nsmb1174.
- Verdel A, Jia S, Gerber S, Sugiyama T, Gygi S, Grewal SI, Moazed D (2004). "RNAi-mediated targeting of heterchromatin by the RITS complex". Science. 303 (5658): 672–676. doi:10.1126/science.1093686. PMC 3244756. PMID 14704433.
- Verdel A, Jia S, Gerber S, Sugiyama T, Gygi S, Grewal SI, Moazed D (2004). "RITS acts in cis to promote RNA interference-mediated transcription and post-transcriptional silencing". Nature Genetics. 36 (11): 1174–1180. doi:10.1038/ng1452. PMID 15475954.
- Sugiyama T, Cam H, Verdel A, Moazed D, Grewal SI (2005). "RNA-dependent RNA polymerase is an essential component of a self-enforcing loop coupling heterochromatin assembly to siRNA production". Proceedings of the National Academy of Sciences of the United States of America. 102 (1): 152–157. doi:10.1073/pnas.0407641102. PMC 544066. PMID 15615848.
- Mochizuki K, Gorovsky MA (2004). "Small RNAs in genome arrangement in Tetrahymena". Current Opinion in Genetics & Development. 14 (2): 181–187. doi:10.1016/j.gde.2004.01.004.
- Sontheimer EJ (2005). "Assembly and function of RNA silencing complexes". Nature Reviews Molecular Cell Biology. 6 (2): 127–138. doi:10.1038/nrm1568.
- Liu Q, Rand TA, Kalidas S, Du F, Kim HE, Smith DP, Wang X (2003). "R2D2, a bridge between the initiation and effector steps of the Drosophila RNAi pathway". Science. 301 (5641): 1921–1925. doi:10.1126/science.1088710. PMID 14512631.
- Pham JW, Pellio JL, Lee YS, Carthew RW, Sontheimer EJ (2004). "A Dicer-2-dependent 80S complex cleaves targeted mRNAs during RNAi in Drosophila". Cell. 117 (1): 83–94. doi:10.1016/S0092-8674(04)00258-2.
- Tomari Y, Du T, Haley B, Schwarz DS, Bennett R, Cook HA, Koppetsch BS, Theurkauf WE, Zamore PD (2004). "RISC assembly defects in the Drosophila RNAi mutant armitage". Cell. 116 (6): 831–841. doi:10.1016/S0092-8674(04)00218-1. PMID 15035985.
- Hammond SM, Boettcher S, Caudy AA, Kobayashi R, Hannon GJ (2001). "Argonaute2, a link between genetic and biochemical analyses of RNAi". Science. 293 (5532): 1146–1150. doi:10.1126/science.1064023. PMID 11498593.
- Caudy AA, Myers M, Hannon GJ, Hammond SM (2002). "Fragile X-related protein and VIG associate with the RNA interference machinery". Genes & Development. 16 (19): 2491–2496. doi:10.1101/gad.1025202. PMC 187452. PMID 12368260.
- Caudy AA, Ketting RF, Hammond SM, Denli AM, Bathoorn AM, Tops BB, Silva JM, Myers MM, Hannon GJ, Plasterk RH (2003). "A micrococcal nuclease homologue in RNAi effector complexes". Nature. 425 (6956): 411–414. doi:10.1038/nature01956. PMID 14508492.
- Rand TA, Ginalski K, Grishin NV, Wang X (2004). "Biochemical identification of Argonaute 2 as the sole protein required for RNA-induced silencing complex activity". Proceedings of the National Academy of Sciences of the United States of America. 101 (40): 14385–14389. doi:10.1073/pnas.0405913101. PMC 521941. PMID 15452342.
- Ishizuka A, Siomi MC, Siomi H (2002). "A Drosophila fragile X protein interacts with components of RNAi and ribosomal proteins". Genes & Development. 16 (19): 2497–2508. doi:10.1101/gad.1022002. PMC 187455. PMID 12368261.
- Martinez J, Patkaniowska A, Urlaub H, Luhrmann R, Tuschl T (2002). "Single-stranded antisense siRNAs guide target RNA cleavage in RNAi". Cell. 110 (5): 563–574. doi:10.1016/S0092-8674(02)00908-X. hdl:11858/00-001M-0000-0012-F2FD-2. PMID 12230974.
- Liu J, Carmell MA, Rivas FV, Marsden CG, Thomson JM, Song JJ, Hammond SM, Joshua-Tor L, Hannon GJ (2004). "Argonaute2 is the catalytic engine of mammalian RNAi". Science. 305 (5689): 1437–1441. doi:10.1126/science.1102513. PMID 15284456.
- Martinez J, Tuschl T (2004). "RISC is a 5′ phosphomonoester-producing RNA endonuclease". Genes & Development. 18 (9): 975–980. doi:10.1101/gad.1187904. PMC 406288. PMID 15105377.
- Meister G, Landthaler M, Patkaniowska A, Dorsett Y, Teng G, Tuschl T (2004). "Human Argonaute2 mediates RNA cleavage targeted by miRNAs and siRNAs". Molecular Cell. 15 (2): 1403–1408. doi:10.1016/j.molcel.2004.07.007. PMID 15260970.
- Mourelatos Z, Dostie J, Paushkin S, Sharma A, Charroux B, Abel L, Rappsilber J, Mann M, Dreyfuss G (2002). "miRNPs: a novel class of ribonucleoproteins containing numerous microRNAs". Genes & Development. 16 (6): 720–728. doi:10.1101/gad.974702. PMC 155365. PMID 11914277.
- Hutvágner G, Zamore PD (2002). "A microRNA in a multiple-turnover RNAi enzyme complex". Science. 297 (5589): 2056–2060. doi:10.1126/science.1073827. PMID 12154197.
- Hall TM (2005). "Structure and function of Argonaute proteins". Cell. 13 (10): 1403–1408. doi:10.1016/j.str.2005.08.005.
- Chendrimada TP, Gregory RI, Kumaraswamy E, Norman J, Cooch N, Nishikura K, Shiekhatter R (2005). "TRBP recruits the Dicer complex to Ago2 for microRNA processing and gene silencing". Nature. 436 (7051): 740–744. doi:10.1038/nature03868. PMC 2944926. PMID 15973356.
- Wang HW, Noland C, Siridechadilok B, Taylor DW, Ma E, Felderer K, Doudna JA, Nogales E (2009). "Structural insights into RNA processing by the human RISC-loading complex". Nature Structural & Molecular Biology. 16 (11): 1148–1153. doi:10.1038/nsmb.1673. PMC 2845538. PMID 19820710.
- Fu Q, Yuan YA (2013). "Structural insights into RISC assembly facilitated by dsRNA-binding domains of human RNA helices A (DHX9)". Nucleic Acids Research. 41 (5): 3457–3470. doi:10.1093/nar/gkt042. PMC 3597700. PMID 23361462.
- Yoo BK, Santhekadur PK, Gredler R, Chen D, Emdad L, Bhutia S, Pannell L, Fisher PB, Sarkar D (2011). "Increased RNA-induced silencing complex (RISC) activity contributes to hepatocellular carcinoma". Hepatology. 53 (5): 1538–1548. doi:10.1002/hep.24216. PMC 3081619. PMID 21520169.
- Yoo BK, Emdad L, Lee SG, Su Z, Santhekadur P, Chen D, Gredler R, Fisher PB, Sarkar D (2011). "Astrocyte elevated gene (AEG-1): a multifunctional regulator of normal and abnormal physiology". Pharmacology & Therapeutics. 130 (1): 1–8. doi:10.1016/j.pharmthera.2011.01.008. PMC 3043119. PMID 21256156.
- Djikeng A, Shi H, Tschudi C, Shen S, Ullu E (2003). "An siRNA ribonucleoprotein is found associated with polyribosomes in Trypanosoma brucei". RNA. 9 (7): 802–808. doi:10.1261/rna.5270203. PMC 1370447. PMID 12810914.
- Sen GL, Wehrman TS, Blau HM (2005). "mRNA translation is not a prerequisite for small interfering RNA-mediated mRNA cleavage". Differentiation. 73 (6): 287–293. doi:10.1111/j.1432-0436.2005.00029.x. PMID 16138829.
- Saumet A, Lecellier CH (2006). "Anti-viral RNA silencing: do we look like plants?". Retrovirology. 3: 3. doi:10.1186/1742-4690-3-3. PMC 1363733. PMID 16409629.
- Bartel DP (2009). "MicroRNAs: target recognition and regulatory functions". Cell. 136 (2): 215–233. doi:10.1016/j.cell.2009.01.002. PMC 3794896. PMID 19167326.
- Jones-Rhoades MW, Bartel DP, Bartel B (2006). "MicroRNAs and their regulator roles in plants". Annual Review of Plant Biology. 57: 19–53. doi:10.1146/annurev.arplant.57.032905.105218. PMID 16669754.
Further reading
- Sontheimer, EJ (2005). "Assembly and function of RNA silencing complexes". Nature Reviews Molecular Cell Biology. 6 (2): 127–138. doi:10.1038/nrm1568.
- Fu Q, Yuan YA (March 2013). "Structural insights into RISC assembly facilitated by dsRNA-binding domains of human RNA helicase A (DHX9)". Nucleic Acids Research. 41 (5): 3457–70. doi:10.1093/nar/gkt042. PMC 3597700. PMID 23361462.
- Schwarz DS, Tomari Y, Zamore PD (2004). "The RNA-induced silencing complex is a Mg2+-dependent endonuclease". Current Biology. 14 (9): 787–91. doi:10.1016/j.cub.2004.03.008. PMID 15120070.
External links
- RNA-Induced+Silencing+Complex at the US National Library of Medicine Medical Subject Headings (MeSH)