Cyclohexane conformation
A cyclohexane conformation is any of several three-dimensional shapes adopted by a cyclohexane molecule. Because many compounds feature structurally similar six-membered rings, the structure and dynamics of cyclohexane are important prototypes of a wide range of compounds.[1][2]
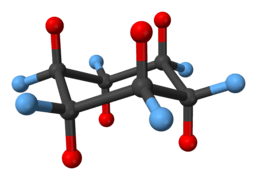
The internal angles of a flat regular hexagon are 120°, while the preferred angle between successive bonds in a carbon chain is about 109.5°, the tetrahedral angle. Therefore, the cyclohexane ring tends to assume certain non-planar (warped) conformations, which have all angles closer to 109.5° and therefore a lower strain energy than the flat hexagonal shape. The most important shapes are chair, half-chair, boat, and twist-boat. Their relative stabilities are: chair > twist boat > boat > half-chair. All relative conformational energies are shown below.[3][4] The molecule can easily switch between these conformations, and only two of them—chair and twist-boat—can be isolated in pure form.
Principal conformers
Chair conformation
The chair conformation is the most stable conformer. At 25 °C, 99.99% of all molecules in a cyclohexane solution adopt this conformation.
The symmetry is D3d. All carbon centers are equivalent. Six hydrogen centers are poised in axial positions, roughly parallel with the C3 axis. Six hydrogen atoms are poised nearly perpendicular to the C3 symmetry axis. These H atoms are respectively referred to as axial and equatorial.
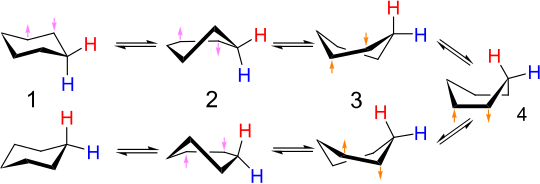
Each carbon bears one "up" and one "down" hydrogen. The C-H bonds in successive carbons are thus staggered so that there is little torsional strain. The chair geometry is often preserved when the hydrogen atoms are replaced by halogens or other simple groups.
Boat and twist-boat conformations
The boat conformations have higher energy than the chair conformations. The interaction between the two flagpole hydrogens, in particular, generates steric strain. Torsional strain also exists between the C2–C3 and C5–C6 bonds, which are eclipsed. Because of this strain, the boat configuration is unstable (i.e. is not a local energy minimum).
The symmetry is C2v.
The boat conformations spontaneously distorts to twist-boat conformations. Here the symmetry is D2, a purely rotational point group. This conformation can be derived from the boat conformation by applying a slight twist to the molecule so as to remove eclipsing of two pairs of methylene groups.
The concentration of the twist-boat conformation at room temperature is less than 0.1%, but at 1073 kelvins it can reach 30%. Rapid cooling of a sample of cyclohexane from 1073 K to 40 K will freeze in a large concentration of twist-boat conformation, which will then slowly convert to the chair conformation upon heating.[5]
Dynamics
Chair-chair
The interconversion of chair conformers is called ring flipping or chair-flipping. Carbon-hydrogen bonds that are axial in one configuration become equatorial in the other, and vice versa. At room temperature the two chair conformations rapidly equilibrate. The proton NMR spectrum of cyclohexane is a singlet at room temperature.
The detailed mechanism of the chair-to-chair interconversion has been the subject of much study and debate.[6] The half-chair state (D, in figure below) is the key transition state in the interconversion between the chair and twist-boat conformations. The half-chair has D2 symmetry. The interconversion between the two chair conformations involves the following sequence: chair → half-chair → twist-boat → half-chair′ → chair′.
Twist-boat - twist-boat
The boat conformation (C, below) is a transition state, allowing the interconversion between two different twist-boat conformations. While the boat conformation is not necessary for interconversion between the two chair conformations of cyclohexane, it is often included in the reaction coordinate diagram used to describe this interconversion because its energy is considerably lower than that of the half-chair, so any molecule with enough energy to go from twist-boat to chair also has enough energy to go from twist-boat to boat. Thus, there are multiple pathways by which a molecule of cyclohexane in the twist-boat conformation can achieve the chair conformation again.
- Conformations: chair (A), twist-boat (B), boat (C) and half-chair (D). Energies are 43 kJ/mol (10 kcal/mol), 25 kJ/mol (6 kcal/mol) and 21 kJ/mol (5 kcal/mol).[3]
Substituted derivatives
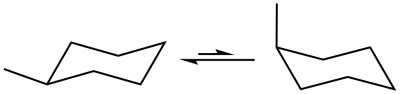
In cyclohexane, the two chair conformations have the same energy. The situation is more complex is substituted derivatives. In methylcyclohexane the two chair conformers are not isoenergetic. The methyl group prefers the equatorial orientation. The preference of a substituent towards the equatorial conformation is measured in terms of its A value, which is the Gibbs free energy difference between the two chair conformations. A positive A value indicates preference towards the equatorial position. The magnitude of the A values ranges from nearly zero for very small substituents such as deuterium, to about 5 kcal/mol (21 kJ/mol) for very bulky substituents such as the tert-butyl group.
Disubstituted cyclohexanes
For 1,2- and 1,4-disubstituted cyclohexanes, a cis configuration leads to one axial and one equatorial group. Such species undergo rapid, degenerate chair flipping. For 1,2- and 1,4-disubstituted cyclohexane, a trans configuration, the diaxial conformation is effectively prevented by its high steric strain. For 1,3-disubstituted cyclohexanes, the cis form is diequatorial and the flipped conformation suffers additional steric interaction between the two axial groups. trans-1,3-Disubstituted cyclohexanes are like cis-1,2- and cis-1,4- and can flip between the two equivalent axial/equatorial forms.[2]
Cis-1,4-Di-tert-butylcyclohexane has an axial tert-butyl group in the chair conformation and conversion to the twist-boat conformation places both groups in more favorable equatorial positions. As a result, the twist-boat conformation is more stable by 0.47 kJ/mol (0.11 kcal/mol) at 125 K as measured by NMR spectroscopy.[6]
Heterocyclic analogs
Heterocyclic analogs of cyclohexane are pervasive in sugars, piperidines, dioxanes, etc. They exist generally follow the trends seen for cyclohexane, i.e. the chair conformer being most stable. The axial-equatorial equilibria (A values) are however strongly affected by the replacement of a methylene by O or NH. Illustrative are the conformations of the glucosides.[2] 1,2,4,5-Tetrathiane ((SCH2)3) lacks the unfavorable 1,3-diaxial interactions of cyclohexane. Consequently its twist-boat conformation is populated; in the corresponding tetramethyl structure, 3,3,6,6-tetramethyl-1,2,4,5-tetrathiane, the twist-boat conformation dominates.
Historical background
In 1890, Hermann Sachse, a 28-year-old assistant in Berlin, published instructions for folding a piece of paper to represent two forms of cyclohexane he called symmetrical and unsymmetrical (what we would now call chair and boat). He clearly understood that these forms had two positions for the hydrogen atoms (again, to use modern terminology, axial and equatorial), that two chairs would probably interconvert, and even how certain substituents might favor one of the chair forms (Sachse–Mohr theory ). Because he expressed all this in mathematical language, few chemists of the time understood his arguments. He had several attempts at publishing these ideas, but none succeeded in capturing the imagination of chemists. His death in 1893 at the age of 31 meant his ideas sank into obscurity. It was only in 1918 when Ernst Mohr, based on the molecular structure of diamond that had recently been solved using the then very new technique of X-ray crystallography,[7][8] was able to successfully argue that Sachse's chair was the pivotal motif.[9][10][11][12][13][14] Derek Barton and Odd Hassel shared the 1969 Nobel Prize for work on the conformations of cyclohexane and various other molecules.
References
- Eliel, Ernest Ludwig; Wilen, Samuel H. (2008). Stereochemistry of Organic Compounds. Wiley India. ISBN 978-8126515707.
- Smith, Michael B.; March, Jerry (2007), Advanced Organic Chemistry: Reactions, Mechanisms, and Structure (6th ed.), New York: Wiley-Interscience, ISBN 978-0-471-72091-1
- J, Clayden (2003). Organic chemistry (2nd ed.). Oxford. p. 373. ISBN 9780191666216.
- Nelson, Donna J.; Brammer, Christopher N. (2011). "Toward Consistent Terminology for Cyclohexane Conformers in Introductory Organic Chemistry". J. Chem. Educ. 88 (3): 292–294. Bibcode:2011JChEd..88..292N. doi:10.1021/ed100172k.
- Squillacote, M.; Sheridan, R. S.; Chapman, O. L.; Anet, F. A. L. (1975-05-01). "Spectroscopic detection of the twist-boat conformation of cyclohexane. Direct measurement of the free energy difference between the chair and the twist-boat". J. Am. Chem. Soc. 97 (11): 3244–3246. doi:10.1021/ja00844a068.
- Gill, G.; Pawar, D. M.; Noe, E. A. (2005). "Conformational Study of cis-1,4-Di-tert-butylcyclohexane by Dynamic NMR Spectroscopy and Computational Methods. Observation of Chair and Twist-Boat Conformations". J. Org. Chem. 70 (26): 10726–10731. doi:10.1021/jo051654z. PMID 16355992.
- Bragg, W. H.; Bragg, W. L. (1913). "The structure of the diamond". Nature. 91 (2283): 557. Bibcode:1913Natur..91..557B. doi:10.1038/091557a0.
- Bragg, W. H.; Bragg, W. L. (1913). "The structure of the diamond". Proc. R. Soc. A. 89 (610): 277–291. Bibcode:1913RSPSA..89..277B. doi:10.1098/rspa.1913.0084.
- Sachse, H. (1890). "Ueber die geometrischen Isomerien der Hexamethylenderivate". Berichte der deutschen chemischen Gesellschaft (in German). Wiley. 23 (1): 1363–1370. doi:10.1002/cber.189002301216. ISSN 0365-9496.
- Sachse, H. (1892-01-01). "Über die Konfigurationen der Polymethylenringe". Zeitschrift für Physikalische Chemie. Walter de Gruyter GmbH. 10U (1): 203. doi:10.1515/zpch-1892-1013. ISSN 2196-7156.
- Sachse, H. (1893-01-01). "Eine Deutung der Affinität". Zeitschrift für Physikalische Chemie. Walter de Gruyter GmbH. 11U (1): 185–219. doi:10.1515/zpch-1893-1114. ISSN 2196-7156.
- Mohr, Ernst (1918-09-20). "Die Baeyersche Spannungstheorie und die Struktur des Diamanten". Journal für Praktische Chemie (in German). Wiley. 98 (1): 315–353. doi:10.1002/prac.19180980123. ISSN 0021-8383.
- Mohr, Ernst (1922-01-14). "Zur Theorie dercis-trans-Isomerie des Dekahydro-naphthalins". Berichte der deutschen chemischen Gesellschaft. Wiley. 55 (1): 230–231. doi:10.1002/cber.19220550128. ISSN 0365-9488.
- This history is nicely summarized here: Archived 2012-02-28 at the Wayback Machine.
Further reading
- Colin A. Russell, 1975, "The Origins of Conformational Analysis," in Van 't Hoff-Le Bel Centennial, O. B. Ramsay, Ed. (ACS Symposium Series 12), Washington, D.C.: American Chemical Society, pp. 159–178.
- William Reusch, 2010, "Ring Conformations" and "Substituted Cyclohexane Compounds," in Virtual Textbook of Organic Chemistry, East Lansing, MI, USA:Michigan State University, see and , accessed 20 June 2015.
External links
- Java applets of all conformations from the University of Nijmegen