Tipping points in the climate system
A tipping point in the climate system is a threshold that, when exceeded, can lead to large changes in the state of the system. Potential tipping points have been identified in the physical climate system, in impacted ecosystems, and sometimes in both.[2] For instance, feedback from the global carbon cycle is a driver for the transition between glacial and interglacial periods, with orbital forcing providing the initial trigger.[3] Earth's geologic temperature record includes many more examples of geologically rapid transitions between different climate states.[4]
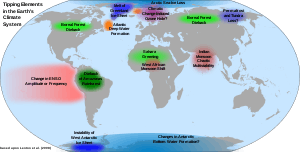
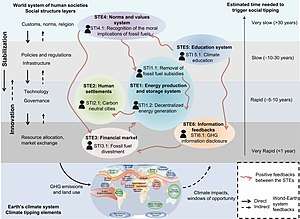
Climate tipping points are of particular interest in reference to concerns about global warming in the modern era. Possible tipping point behaviour has been identified for the global mean surface temperature by studying self-reinforcing feedbacks and the past behavior of Earth's climate system. Self-reinforcing feedbacks in the carbon cycle and planetary reflectivity could trigger a cascading set of tipping points that lead the world into a hothouse climate state.[5][6]
Large-scale components of the Earth system that may pass a tipping point have been referred to as tipping elements.[7] Tipping elements are found in the Greenland and Antarctic ice sheets, possibly causing tens of meters of sea level rise. These tipping points are not always abrupt. For example, at some level of temperature rise the melt of a large part of the Greenland ice sheet and/or West Antarctic Ice Sheet will become inevitable; but the ice sheet itself may persist for many centuries.[8] Some tipping elements, like the collapse of ecosystems, are irreversible.[2]
Definition
The IPCC AR5 defines a tipping point as an irreversible change in the climate system. It states that the precise levels of climate change sufficient to trigger a tipping point remain uncertain, but that the risk associated with crossing multiple tipping points increases with rising temperature.[9][Notes 1] A broader definition of tipping points is sometimes used as well, which includes abrupt but reversible tipping points.[10][11]
In the context of climate change, an "adaptation tipping point" has been defined as "the threshold value or specific boundary condition where ecological, technical, economic, spatial or socially acceptable limits are exceeded."[12]
Tipping point behaviour in the climate can also be described in mathematical terms. Tipping points are then seen as any type of bifurcation with hysteresis.[13][14] Hysteresis is the dependence of the state of a system on its history. For instance, depending on how warm and cold it was in the past, there can be differing amounts of ice present on the poles at the same concentration of greenhouse gases or temperature.[15]
In a study inspired by "mathematical and statistical approaches to climate modelling and prediction", the authors identify three types of tipping points in open systems such as the climate system—bifurcation, noise-induced and rate-dependent.[16] The idea of tipping points in climate science, as indicated by palaeoclimate data and global climate models, suggest that the "climate system may abruptly 'tip' from one regime to another in a comparatively short time."[16]
Bifurcation-induced tipping refers to changes in dynamical systems that occur when a small smooth change made to bifurcation parameters of the system causes an abrupt or sudden topological change in the behavior of the system. In the Atlantic Meridional Overturning Circulation (AMOC), slow passage to the bifurcation parameters—the salinity, temperature and density of water—could cause the AMOC to abruptly collapse when it reaches a critical tipping point.[17] Currents of warm, seawater in the upper layers of the Atlantic flow north, while currents of colder, deep waters from the North Atlantic flow south, like a conveyor belt known as thermohaline circulation. Downwelling occurs when the warmer, higher-density seawater accumulates and sinks beneath the colder, lower density less saline water from glacier melt. An AMOC collapse would occur if downwelling was inhibited.[18] [critical slowing down] (CSD) "occurs because a restoring feedback is weakening as a bifurcation-type tipping point is approached."[17]
Noise-induced tipping refers to transitions due to random fluctuations or internal variability of the system, as in the Dansgaard-Oeschger events[19] during the last glacial period, with 25 occurrences of rapid climate fluctuations.
Rate-induced tipping occurs in an "excitable system"—such as peatlands—when one of the systems parameters is "ramped" through a "steady, slow and monotonic change" eliciting a "large excitable response". In the case of peatlands, the rate-induced tipping point results in an "explosive release of soil carbon from peatlands into the atmosphere"—"compost bomb instability".[20][21]
Tipping points for global temperature
There are many positive and negative feedbacks to global temperatures and the carbon cycle that have been identified. The IPCC reports that feedbacks to increased temperatures are net positive for the remainder of this century, with the impact of cloud cover the largest uncertainty.[22] IPCC carbon cycle models show higher ocean uptake of carbon corresponding to higher concentration pathways, but land carbon uptake is uncertain due to the combined effect of climate change and land use changes.[23]
The geologic record of temperature and greenhouse gas concentration allows climate scientists to gather information on climate feedbacks that lead to different climate states, such as the Late Quaternary (past 1.2 million years), the Pliocene period five million years ago and the Cretaceous period, 100 million years ago. Combining this information with the understanding of current climate change resulted in the finding that "A 2 °C warming could activate important tipping elements, raising the temperature further to activate other tipping elements in a domino-like cascade that could take the Earth System to even higher temperatures".[5]
The speed of tipping point feedbacks is a critical concern and the geologic record often fails to provide clarity as to whether past temperature changes have taken only a few decades or many millennia of time. For instance, a tipping point that was once feared to be abrupt and overwhelming is the release of clathrate compounds buried in seabeds and seabed permafrost,[24] but that feedback is now thought to be chronic and long term.[25]
Some individual feedbacks may be strong enough to trigger tipping points on their own. A 2019 study predicts that if greenhouse gases reach three times the current level of atmospheric carbon dioxide that stratocumulus clouds could abruptly disperse, contributing an additional 8 degrees Celsius of warming.[26]
Runaway greenhouse effect
The runaway greenhouse effect is used in astronomical circles to refer to a greenhouse effect that is so extreme that oceans boil away and render a planet uninhabitable, an irreversible climate state that happened on Venus. The IPCC Fifth Assessment Report states that "a 'runaway greenhouse effect' —analogous to Venus— appears to have virtually no chance of being induced by anthropogenic activities."[27] Venus-like conditions on the Earth require a large long-term forcing that is unlikely to occur until the sun brightens by a few tens of percents, which will take a few billion years.[28]
While a runaway greenhouse effect on Earth is virtually impossible, there are indications that Earth could enter a moist greenhouse state that renders large parts of Earth uninhabitable if the climate forcing is large enough to make water vapour (H2O) a major atmospheric constituent.[29] Conceivable levels of human-made climate forcing would increase water vapour to about 1% of the atmosphere's mass, thus increasing the rate of hydrogen escape to space. If such a forcing were entirely due to CO2, the weathering process would remove the excess atmospheric CO2 well before the ocean was significantly depleted.[28]
Tipping elements
Large scale tipping elements
A smooth or abrupt change in temperature can trigger global-scale tipping points. In the cryosphere these include the irreversible melting of Greenland and Antarctic ice sheets. In Greenland, a positive feedback cycle exists between melting and surface elevation. At lower elevations, temperatures are higher, leading to additional melting. This feedback loop can become so strong that irreversible melting occurs.[7] Marine ice sheet instability could trigger a tipping point in West Antarctica.[2] Crossing either of these tipping points leads to accelerated global sea level rise.[8]
When fresh water gets released as a consequence of Greenland melting, a threshold may be crossed which leads to disruption of the thermohaline circulation.[30] The thermohaline circulation transports heat northward which is important for temperature regulation in the Atlantic region.[31] Risks for a complete shutdown are low to moderate under the Paris agreement levels of warming.[2]
Other examples of possible large scale tipping elements are a shift in El Niño–Southern Oscillation. After crossing a tipping point, the warm phase (El Niño) would start to occur more often. Lastly, the southern ocean, which now absorbs a lot of carbon, might switch to a state where it does not do this anymore.[2]
Regional tipping elements
Climate change can trigger regional tipping points as well. Examples are the disappearance of Arctic sea ice,[32][33] the establishment of woody species in tundra, permafrost loss, the collapse of the monsoon of South Asia and a strengthening of the West African monsoon which would lead to greening of the Sahara and Sahel.[2] Deforestation may trigger a tipping point in rainforests (i.e. Savannization in the Amazon rainforest, ...). As rain forests recycle a large part of their rainfall, when a portion of the forest is destroyed local droughts may threaten the remainder.[2] Finally, boreal forests are considered a tipping element as well. Local warming causes trees to die at a higher rate than before, in proportion to the rise in temperature. As more trees die, the woodland becomes more open, leading to further warming and making forests more susceptible to fire. The tipping point is difficult to predict, but is estimated to be between 3–4 °C of global temperature rise.[2]
Cascading tipping points
Crossing a threshold in one part of the climate system may trigger another tipping element to tip into a new state. These are so-called cascading tipping points.[34] Ice loss in West Antarctica and Greenland will significantly alter ocean circulation. Sustained warming of the northern high latitudes as a result of this process could activate tipping elements in that region, such as permafrost degradation, loss of Arctic sea ice, and Boreal forest dieback.[6] This illustrates that even at relatively low levels of global warming, relatively stable tipping elements may be activated.[35]
Timothy Lenton at Exeter University, England and his team of researchers, had first warned in their landmark 7 February 2008 PNAS paper, about the "risks of climate tipping points."[36] In 2008, Lenton and his team "thought the dangers would only arise when global warming exceeded 5 degrees Celsius (9 degrees Fahrenheit) above pre-industrial levels."[36][7] A new study published in Nature on 27 November 2019 by Lenton and 6 co-authors, warned in language that is "much starker" than the Intergovernmental Panel on Climate Change's forecasts,[37] that risks are "much more likely and much more imminent" and that some "may already have been breached."[37]
Early warning signals
For some of the tipping points described above, it may be possible to detect whether that part of the climate system is getting closer to a tipping point;[38] however, detection can note only that abrupt changes are likely, while predicting when and where they will occur remains difficult.[39] A premier mode of detection for these warning signals is through natural archives like sediments, ice caps, and tree rings, where past changes in climate can be observed.[40][38]All parts of the climate system are sometimes disturbed by weather events. After the disruption, the system moves back to its equilibrium. A storm may damage sea ice, which grows back after the storm has passed. If a system is getting closer to tipping, this restoration to its normal state might take increasingly longer, which can be used as a warning sign of tipping.[41][42]
Changes in the Arctic
A 2019 UNEP study indicates that now at least for the Arctic and the Greenland ice sheet a tipping point has already been reached.[43][44] Because of dewing of permafrost soil, more methane (in addition to other short-lived climate pollutant) could enter the atmosphere earlier than previously predicted and the loss of reflecting ice shields has started a powerful positive feedback loop leading to ever higher temperatures. The resulting accelerating climate instability in the polar region has potential to affect the global climate, outdating previous predictions[45][46][47][48][49] about the point in the future when global tipping will occur.
A more regional tipping point may have already been reached in the form of a mass recession of Arctic sea ice. According to scientist Ron Lindsay at the University of Washington Applied Physics Laboratory, a tipping point in the Arctic materializes as a positive feedback loop, where "increased summer melt means decreased winter growth and then even more melting the next summer, and so on."[50] The loss of Arctic sea ice, while detrimental to the region, also holds severe consequences for the rest of the globe. Critically important is the role of sea ice in increasing the Earth's albedo, or reflectivity. Sea ice has an albedo level of 0.5 to 0.7, reflecting fifty to seventy percent of incoming energy, while the ocean beneath has an albedo of only .06, reflecting only six percent of incoming energy.[51] As sea ice decreases and exposes the less reflective ocean, albedo decreases across the region. Summer sea ice is of particular importance, reflecting approximately fifty percent of incoming radiation back into space at a time when there is already an increase in daylight in the Arctic.[52] NOAA (National Oceanic and Atmospheric Administration) notes that in September of 2019, "the sea ice cover reached its annual summer minimum, tying with 2007 and 2016 for second-smallest on record."[53]
In June 2019, satellite images from around the Arctic showed burning fires that are farther north and of greater magnitude than at any time in the 16-year satellite record, and some of the fires appear to have ignited peat soils.[54] Peat is an accumulation of partially decayed vegetation and is an efficient carbon sink.[55] Scientists are concerned because the long-lasting peat fires release their stored carbon back to the atmosphere, contributing to further warming. The fires in June 2019, for example, released as much carbon dioxide as Sweden's annual greenhouse gas emissions.[56]
Tipping point effects
If the climate tips into a hothouse Earth scenario, some scientists warn of food and water shortages, hundreds of millions of people being displaced by rising sea levels, unhealthy and unlivable conditions, and coastal storms having larger impacts.[35] Runaway climate change of 4–5 °C can make swathes of the planet around the equator uninhabitable, with sea levels up to 60 metres (197 ft) higher than they are today.[57] Humans cannot survive if the air is too moist and hot, which would happen for the majority of human populations if global temperatures rise by 11–12 °C, as land masses warm faster than the global average.[58] Effects like these have been popularized in books like The Uninhabitable Earth and The End of Nature.
Notes
- Valerie Masson-Delmotte included the IPCC_SROCC definition on 27 April 2020 in her Twitter series #1day1wordforclimate: "Tipping point A level of change in system properties beyond which a system reorganises, often in a nonlinear manner, and does not return to the initial state even if the drivers of the change are abated. For the climate system, the term refers to a critical threshold when global or regional climate changes from one stable state to another stable state. Tipping points are also used when referring to impact: the term can imply that an impact tipping point is (about to be) reached in a natural or human system. See also Adaptation, Human system, Impact, Irreversibility, and Natural Systems."
See also
References
- Otto, I.M. (4 February 2020). "Social tipping elements for stabilizing climate by 2050". PNAS. 117 (5): 2354–2365. doi:10.1073/pnas.1900577117. PMC 7007533. PMID 31964839.
- Hoegh-Guldberg, O.D.; Jacob, M.; Taylor, M.; S., Bindi; Brown, I. (2018). "Impacts of 1.5°C of Global Warming on Natural and Human Systems" (PDF). Global Warming of 1.5°C.
- Shackleton, N. J. (2000). "The 100,000-Year Ice-Age Cycle Identified and Found to Lag Temperature, Carbon Dioxide, and Orbital Eccentricity". Science. 289 (5486): 1897–902. Bibcode:2000Sci...289.1897S. doi:10.1126/science.289.5486.1897. PMID 10988063.
- Zachos, J.; Pagani, M.; Sloan, L.; Thomas, E.; Billups, K. (2001). "Trends, rhythms, and aberrations in global climate 65 Ma to present". Science. 292 (5517): 686–693. Bibcode:2001Sci...292..686Z. doi:10.1126/science.1059412. PMID 11326091.
- Sheridan, Kerry (6 August 2018). "Earth risks tipping into 'hothouse' state: study". Phys.org. Retrieved 8 August 2018.
Hothouse Earth is likely to be uncontrollable and dangerous to many ... global average temperatures would exceed those of any interglacial period—meaning warmer eras that come in between Ice Ages—of the past 1.2 million years.
- Lenton, Timothy M.; Rockström, Johan; Gaffney, Owen; Rahmstorf, Stefan; Richardson, Katherine; Steffen, Will; Schellnhuber, Hans Joachim (27 November 2019). "Climate tipping points — too risky to bet against". Nature. 575 (7784): 592–595. Bibcode:2019Natur.575..592L. doi:10.1038/d41586-019-03595-0. PMID 31776487.
- Lenton, T.M.; Held, H.; Kriegler, E.; Hall, J.W.; Lucht, W.; Rahmstorf, S.; Schellnhuber, H.J. (2008). "Tipping elements in the Earth's climate system". Proceedings of the National Academy of Sciences. 105 (6): 1786–1793. Bibcode:2008PNAS..105.1786L. doi:10.1073/pnas.0705414105. PMC 2538841. PMID 18258748.
- "Tipping points in Antarctic and Greenland ice sheets". NESSC. 12 November 2018. Retrieved 25 February 2019.
- IPCC AR5 WGII (2014). "Summary for policymakers" (PDF). Climate change 2014: Impacts, Adaptation and Vulnerability (Report).
- Lenton, Timothy M. (2011). "Early warning of climate tipping points". Nature Climate Change. 1 (4): 201–209. Bibcode:2011NatCC...1..201L. doi:10.1038/nclimate1143. ISSN 1758-6798.
- Livina, V.N.; Lohmann, G.; Mudelsee, M.; Lenton, T.M. (2013). "Forecasting the underlying potential governing the time series of a dynamical system". Physica A: Statistical Mechanics and Its Applications. 392 (18): 3891–3902. arXiv:1212.4090. Bibcode:2013PhyA..392.3891L. doi:10.1016/j.physa.2013.04.036.
- Ahmed, Farhana; Khan, M Shah Alam; Warner, Jeroen; Moors, Eddy; Terwisscha Van Scheltinga, Catharien (28 June 2018). "Integrated Adaptation Tipping Points (IATPs) for urban flood resilience". Environment and Urbanization. 30 (2): 575–596. doi:10.1177/0956247818776510. ISSN 0956-2478.
- Lenton, Timothy M.; Williams, Hywel T.P. (2013). "On the origin of planetary-scale tipping points". Trends in Ecology and Evolution. 28 (7): 380–382. doi:10.1016/j.tree.2013.06.001. PMID 23777818.
- Smith, Adam B.; Revilla, Eloy; Mindell, David P.; Matzke, Nicholas; Marshall, Charles; Kitzes, Justin; Gillespie, Rosemary; Williams, John W.; Vermeij, Geerat (2012). "Approaching a state shift in Earth's biosphere". Nature. 486 (7401): 52–58. Bibcode:2012Natur.486...52B. doi:10.1038/nature11018. hdl:10261/55208. ISSN 1476-4687. PMID 22678279.
- Pollard, David; DeConto, Robert M. (2005). "Hysteresis in Cenozoic Antarctic ice-sheet variations". Global and Planetary Change. 45 (1–3): 9–12. doi:10.1016/j.gloplacha.2004.09.011.
- Ashwin, Peter; Wieczorek, Sebastian; Vitolo, Renato; Cox, Peter (13 March 2012). "Tipping points in open systems: bifurcation, noise-induced and rate-dependent examples in the climate system". Philosophical Transactions of the Royal Society A: Mathematical, Physical and Engineering Sciences. 370 (1962): 1166–1184. arXiv:1103.0169. Bibcode:2012RSPTA.370.1166A. doi:10.1098/rsta.2011.0306. ISSN 1364-503X. PMID 22291228.
- Boulton, Chris A.; Allison, Lesley C.; Lenton, Timothy M. (December 2014). "Early warning signals of Atlantic Meridional Overturning Circulation collapse in a fully coupled climate model". Nature Communications. 5 (1): 5752. Bibcode:2014NatCo...5.5752B. doi:10.1038/ncomms6752. ISSN 2041-1723. PMC 4268699. PMID 25482065.
- Dijkstra, Henk A. "Characterization of the multiple equilibria regime in a global ocean model." Tellus A: Dynamic Meteorology and Oceanography 59.5 (2007): 695–705.
- Ditlevsen, Peter D.; Johnsen, Sigfus J. (2010). "Tipping points: Early warning and wishful thinking". Geophysical Research Letters. 37 (19): n/a. Bibcode:2010GeoRL..3719703D. doi:10.1029/2010GL044486. ISSN 1944-8007.
- Wieczorek, S.; Ashwin, P.; Luke, C. M.; Cox, P. M. (8 May 2011). "Excitability in ramped systems: the compost-bomb instability". Proceedings of the Royal Society A: Mathematical, Physical and Engineering Sciences. 467 (2129): 1243–1269. Bibcode:2011RSPSA.467.1243W. doi:10.1098/rspa.2010.0485. ISSN 1364-5021.
- Luke, C. M.; Cox, P. M. (2011). "Soil carbon and climate change: from the Jenkinson effect to the compost-bomb instability". European Journal of Soil Science. 62 (1): 5–12. doi:10.1111/j.1365-2389.2010.01312.x. ISSN 1365-2389.
- IPCC AR5 (2013). "Technical Summary- TFE.6 Climate Sensitivity and Feedbacks" (PDF). Climate Change 2013: The Physical Science Basis (Report).
The water vapour/lapse rate, albedo and cloud feedbacks are the principal determinants of equilibrium climate sensitivity. All of these feedbacks are assessed to be positive, but with different levels of likelihood assigned ranging from likely to extremely likely. Therefore, there is high confidence that the net feedback is positive and the black body response of the climate to a forcing will therefore be amplified. Cloud feedbacks continue to be the largest uncertainty.
- IPCC AR5 (2013). "Technical Summary- TFE.7 Carbon Cycle Perturbation and Uncertainties" (PDF). Climate Change 2013: The Physical Science Basis (Report).
- Archer, David (2007). "Methane hydrate stability and anthropogenic climate change" (PDF). Biogeosciences. 4 (4): 521–544. Bibcode:2007BGeo....4..521A. doi:10.5194/bg-4-521-2007. Retrieved 25 May 2009.
- "Study finds hydrate gun hypothesis unlikely". Phys.org. 23 August 2017.
- Emiliano Rodríguez Mega (26 February 2019). "Clouds' cooling effect could vanish in a warmer world". Nature. doi:10.1038/d41586-019-00685-x. Retrieved 24 March 2019.
High concentrations of atmospheric carbon dioxide can result in the dispersal of cloud banks that reflect roughly 30% of the sunlight that hits them.
- Scoping of the IPCC 5th Assessment Report Cross Cutting Issues (PDF). Thirty-first Session of the IPCC Bali, 26–29 October 2009 (Report). Archived (PDF) from the original on 9 November 2009. Retrieved 24 March 2019.
- Hansen, James; Sato, Makiko; Russell, Gary; Kharecha, Pushker (2013). "Climate sensitivity, sea level and atmospheric carbon dioxide". Philosophical Transactions of the Royal Society A: Mathematical, Physical and Engineering Sciences. 371 (2001). 20120294. arXiv:1211.4846. Bibcode:2013RSPTA.37120294H. doi:10.1098/rsta.2012.0294. PMC 3785813. PMID 24043864.
- Kasting, JF (1988). "Runaway and moist greenhouse atmospheres and the evolution of Earth and Venus". Icarus. 74 (3): 472–494. Bibcode:1988Icar...74..472K. doi:10.1016/0019-1035(88)90116-9. PMID 11538226.
- Lenton, Timothy M. (2012). "Arctic Climate Tipping Points". AMBIO. 41 (1): 10–22. doi:10.1007/s13280-011-0221-x. ISSN 1654-7209. PMC 3357822. PMID 22270703.
- Belaia, Mariia; Funke, Michael; Glanemann, Nicole (2017). "Global Warming and a Potential Tipping Point in the Atlantic Thermohaline Circulation: The Role of Risk Aversion" (PDF). Environmental and Resource Economics. 67 (1): 93–125. doi:10.1007/s10640-015-9978-x. ISSN 1573-1502.
- Mary-Louise Timmermans, John Toole, Richard Krishfield (29 August 2018). "Warming of the interior Arctic Ocean linked to sea ice losses at the basin margins". Science Advances. 4 (8): eaat6773. Bibcode:2018SciA....4.6773T. doi:10.1126/sciadv.aat6773. PMC 6114986. PMID 30167462.CS1 maint: multiple names: authors list (link)
- Toth, Katie (29 August 2018). "Warm water under Arctic ice a 'ticking time bomb,' researcher says". CBC.
- Rocha, Juan C.; Peterson, Garry; Bodin, Örjan; Levin, Simon (2018). "Cascading regime shifts within and across scales". Science. 362 (6421): 1379–1383. Bibcode:2018Sci...362.1379R. doi:10.1126/science.aat7850. ISSN 0036-8075. PMID 30573623.
- Schellnhuber, Hans Joachim; Winkelmann, Ricarda; Scheffer, Marten; Lade, Steven J.; Fetzer, Ingo; Donges, Jonathan F.; Crucifix, Michel; Cornell, Sarah E.; Barnosky, Anthony D. (2018). "Trajectories of the Earth System in the Anthropocene". Proceedings of the National Academy of Sciences. 115 (33): 8252–8259. Bibcode:2018PNAS..115.8252S. doi:10.1073/pnas.1810141115. ISSN 0027-8424. PMC 6099852. PMID 30082409.
- Pearce, Fred (5 December 2019), "As Climate Change Worsens, A Cascade of Tipping Points Looms", Yale E360, retrieved 7 December 2019
- Lenton, Timothy M.; Rockström, Johan; Gaffney, Owen; Rahmstorf, Stefan; Richardson, Katherine; Steffen, Will; Schellnhuber, Hans Joachim (27 November 2019). "Climate tipping points — too risky to bet against". Nature. Comment. 575 (7784): 592–595. Bibcode:2019Natur.575..592L. doi:10.1038/d41586-019-03595-0. PMID 31776487.
- Bradley, Raymond S. (2011). "Natural archives, changing climates" (PDF). Contributions to Science. 7: 21–25.
- "Scientists identify climate 'tipping points'". ScienceDaily. Retrieved 20 April 2020.
- Thomas, Zoë A. (15 November 2016). "Using natural archives to detect climate and environmental tipping points in the Earth System". Quaternary Science Reviews. 152: 60–71. doi:10.1016/j.quascirev.2016.09.026. ISSN 0277-3791.
- Lenton, Timothy .M.; Livina, V.N.; Dakos, V.; Van Nes, E.H.; Scheffer, M. (2012). "Early warning of climate tipping points from critical slowing down: comparing methods to improve robustness". Philosophical Transactions of the Royal Society A: Mathematical, Physical and Engineering Sciences. 370 (1962): 1185–1204. Bibcode:2012RSPTA.370.1185L. doi:10.1098/rsta.2011.0304. ISSN 1364-503X. PMC 3261433. PMID 22291229.
- Williamson, Mark S.; Bathiany, Sebastian; Lenton, Tim (2016). "Early warning signals of tipping points in periodically forced systems". Earth System Dynamics. 7 (2): 313–326. Bibcode:2016ESD.....7..313W. doi:10.5194/esd-7-313-2016.
- "Temperature rise is 'locked-in' for the coming decades in the Arctic". UN Environment (Press release). 13 March 2019. Retrieved 15 July 2019.
- Schoolmeester T, Gjerdi HL, Crump J, Alfthan B, Fabres J, Johnsen K, Puikkonen L, Kurvits T, Baker E (11 March 2019). Global Linkages – A graphic look at the changing Arctic (PDF) (Report) (rev.1 ed.). UN Environment Programme and GRID-Arendal.
- Yumashev, Dmitry; Hope, Chris; Schaefer, Kevin; Riemann-Campe, Kathrin; Iglesias-Suarez, Fernando; Jafarov, Elchin; Burke, Eleanor J.; Young, Paul J.; Elshorbany, Yasin; Whiteman, Gail (2019). "Climate policy implications of nonlinear decline of Arctic land permafrost and other cryosphere elements". Nature Communications. 10 (1): 1900. Bibcode:2019NatCo..10.1900Y. doi:10.1038/s41467-019-09863-x. PMC 6478735. PMID 31015475.
- Hahn, Jonathan (25 January 2019). "Climate Could Hit a Tipping Point Sooner Than You Think". Sierra Club. Retrieved 10 July 2019.
- Harvey, Chelsea (24 April 2019). "Climate 'Tipping Points' Could Add Trillions to the Costs of Warming". Scientific American. Retrieved 10 July 2019.
- Saplakoglu, Yasemin (6 August 2018). "The Planet Is Dangerously Close to the Tipping Point for a 'Hothouse Earth'". Live Science. Retrieved 10 July 2019.
- "Climate change tipping point could be coming sooner than we think: study". Phys.org. 23 January 2019. Retrieved 10 July 2019.
- Renfrow, Stephanie. "Arctic sea ice on the wane: Now what?". Earthdata. NASA. Retrieved 20 April 2020.
- "Thermodynamics: Albedo". National Snow and Ice Data Center. Cooperative Institute for Research in Environmental Sciences. Retrieved 20 April 2020.
- Wadhams, Peter. "The Global Impacts of Rapidly Disappearing Arctic Sea Ice". Yale E360. Retrieved 20 April 2020.
- Lindsey, Rebecca; Scott, Michon (26 September 2019). "Climate Change: Arctic sea ice summer minimum | NOAA Climate.gov". Climate.gov. National Oceanic and Atmospheric Administration. Retrieved 20 April 2020.
- Hines, Morgan (23 January 2019). "Thanks to climate change, parts of the Arctic are on fire. Scientists are concerned". USA Today. Retrieved 30 August 2019.
- Hugron, Sandrine; Bussières, Julie; Rochefort, Line (2013). Tree plantations within the context of ecological restoration of peatlands: practical guide (PDF) (Report). Laval, Québec, Canada: Peatland Ecology Research Group (PERG). Retrieved 22 February 2014.
- Edward Helmore (26 July 2019). "'Unprecedented': more than 100 Arctic wildfires burn in worst ever season". The Guardian. Retrieved 30 August 2019.
- "Earth 'just decades away from global warming tipping point which threatens future of humanity'". ITV News. 6 August 2018. Retrieved 25 February 2019.
- Sherwood, S.C.; Huber, M. (2010). "An adaptability limit to climate change due to heat stress". PNAS. 107 (21): 9552–9555. Bibcode:2010PNAS..107.9552S. doi:10.1073/pnas.0913352107. PMC 2906879. PMID 20439769.
External links
![]() |
Wikiquote has quotations related to: Tipping points in the climate system |
- Billings, Lee (12 March 2010). "How the extinction of the dinosaurs, Arctic methane leaks, and nuclear weaponry reveal the precarious thresholds of life on Earth". Seed.
- Cascio, Jamais (9 March 2010). "Pushing Back Against the Methane Tipping Point". Worldchanging. Archived from the original on 29 April 2010.
- Robert McSweeny (10 February 2020). "Explainer: Nine ‘tipping points’ that could be triggered by climate change". Carbon Brief.
- Keim, Brandon (23 December 2009). "7 Tipping Points That Could Transform Earth". Wired.
- Quick-Change Planet: Do Global Climate Tipping Points Exist? March 25, 2013 Scientific American
- ClimateClock: time left to reaching the 1,5°C threshold