Sepsis
Sepsis is a life-threatening condition that arises when the body's response to infection causes injury to its tissues and organs.[5] This initial stage is followed by suppression of the immune system.[9] Common signs and symptoms include fever, increased heart rate, increased breathing rate, and confusion.[2] There may also be symptoms related to a specific infection, such as a cough with pneumonia, or painful urination with a kidney infection.[3] The very young, old, and people with a weakened immune system may have no symptoms of a specific infection, and the body temperature may be low or normal instead of having a fever.[3] Severe sepsis is sepsis causing poor organ function or blood flow.[10] The presence of low blood pressure, high blood lactate, or low urine output may suggest poor blood flow.[10] Septic shock is low blood pressure due to sepsis that does not improve after fluid replacement.[10]
Sepsis | |
---|---|
Other names | Septicemia, blood poisoning |
Blood culture bottles: orange cap for anaerobes, green cap for aerobes, and yellow cap for blood samples from children[1] | |
Pronunciation |
|
Specialty | Infectious disease |
Symptoms | Fever, increased heart rate, low blood pressure, increased breathing rate, confusion[2] |
Causes | Immune response triggered by an infection[3][4] |
Risk factors | Young or old age, cancer, diabetes, major trauma, burns[2] |
Diagnostic method | Systemic inflammatory response syndrome (SIRS),[3] qSOFA[5] |
Treatment | Intravenous fluids, antimicrobials[2][6] |
Prognosis | 10 to 80% risk of death[5][7] |
Frequency | 0.2–3 per 1000 a year (developed world)[7][8] |
Sepsis is an inflammatory immune response triggered by an infection.[3][4] Bacterial infections are the most common cause, but fungal, viral, and protozoan infections can also lead to sepsis.[3] Common locations for the primary infection include the lungs, brain, urinary tract, skin, and abdominal organs.[3] Risk factors include being very young, older age, a weakened immune system from conditions such as cancer or diabetes, major trauma, or burns.[2] Previously, a sepsis diagnosis required the presence of at least two systemic inflammatory response syndrome (SIRS) criteria in the setting of presumed infection.[3] In 2016, a shortened sequential organ failure assessment score (SOFA score), known as the quick SOFA score (qSOFA), replaced the SIRS system of diagnosis.[5] qSOFA criteria for sepsis include at least two of the following three: increased breathing rate, change in the level of consciousness, and low blood pressure.[5] Sepsis guidelines recommend obtaining blood cultures before starting antibiotics; however, the diagnosis does not require the blood to be infected.[3] Medical imaging is helpful when looking for the possible location of the infection.[10] Other potential causes of similar signs and symptoms include anaphylaxis, adrenal insufficiency, low blood volume, heart failure, and pulmonary embolism.[3]
Sepsis requires immediate treatment with intravenous fluids and antimicrobials.[2][6] Ongoing care often continues in an intensive care unit.[2] If an adequate trial of fluid replacement is not enough to maintain blood pressure, then the use of medications that raise blood pressure becomes necessary.[2] Mechanical ventilation and dialysis may be needed to support the function of the lungs and kidneys, respectively.[2] A central venous catheter and an arterial catheter may be placed for access to the bloodstream and to guide treatment.[10] Other helpful measurements include cardiac output and superior vena cava oxygen saturation.[10] People with sepsis need preventive measures for deep vein thrombosis, stress ulcers, and pressure ulcers unless other conditions prevent such interventions.[10] Some people might benefit from tight control of blood sugar levels with insulin.[10] The use of corticosteroids is controversial, with some reviews finding benefit,[11][12] and others not.[13]
Disease severity partly determines the outcome.[7] The risk of death from sepsis is as high as 30%, while for severe sepsis it is as high as 50%, and septic shock 80%.[7] Sepsis affected about 49 million people in 2017, with 11 million deaths (1 in 5 deaths worldwide).[14] In the developed world, approximately 0.2 to 3 people per 1000 are affected by sepsis yearly, resulting in about a million cases per year in the United States.[7][8] Rates of disease have been increasing.[10] Sepsis is more common among males than females.[3] Descriptions of sepsis date back to the time of Hippocrates.[15] The terms "septicemia" and "blood poisoning" have been used in various ways and are no longer recommended.[15][16]
Signs and symptoms
In addition to symptoms related to the actual cause, people with sepsis may have a fever, low body temperature, rapid breathing, a fast heart rate, confusion, and edema.[17] Early signs include a rapid heart rate, decreased urination, and high blood sugar. Signs of established sepsis include confusion, metabolic acidosis (which may be accompanied by a faster breathing rate that leads to respiratory alkalosis), low blood pressure due to decreased systemic vascular resistance, higher cardiac output, and disorders in blood-clotting that may lead to organ failure.[18] Fever is the most common presenting symptom in sepsis, but fever may be absent in some people such as the elderly or those who are immunocompromized.[19]
The drop in blood pressure seen in sepsis can cause lightheadedness and is part of the criteria for septic shock.[20]
Cause
Infections leading to sepsis are usually bacterial but may be fungal or viral.[21] Gram-positive bacteria were the primary cause of sepsis before the introduction of antibiotics in the 1950s. After the introduction of antibiotics, gram-negative bacteria became the predominant cause of sepsis from the 1960s to the 1980s.[22] After the 1980s, gram-positive bacteria, most commonly staphylococci, are thought to cause more than 50% of cases of sepsis.[8][23] Other commonly implicated bacteria include Streptococcus pyogenes, Escherichia coli, Pseudomonas aeruginosa, and Klebsiella species.[24] Fungal sepsis accounts for approximately 5% of severe sepsis and septic shock cases; the most common cause of fungal sepsis is an infection by Candida species of yeast,[25] a frequent hospital-acquired infection.
The most common sites of infection resulting in severe sepsis are the lungs, the abdomen, and the urinary tract.[21] Typically, 50% of all sepsis cases start as an infection in the lungs. In one third to one-half of cases, the source of infection is unclear.[21]
Diagnosis
Early diagnosis is necessary to properly manage sepsis, as the initiation of rapid therapy is key to reducing deaths from severe sepsis.[10] Some hospitals use alerts generated from electronic health records to bring attention to potential cases as early as possible.[26]
Within the first three hours of suspected sepsis, diagnostic studies should include white blood cell counts, measuring serum lactate, and obtaining appropriate cultures before starting antibiotics, so long as this does not delay their use by more than 45 minutes.[10] To identify the causative organism(s), at least two sets of blood cultures using bottles with media for aerobic and anaerobic organisms are necessary. At least one should be drawn through the skin and one through each vascular access device (such as an IV catheter) that has been in place more than 48 hours.[10] Bacteria are present in the blood in only about 30% of cases.[27] Another possible method of detection is by polymerase chain reaction. If other sources of infection are suspected, cultures of these sources, such as urine, cerebrospinal fluid, wounds, or respiratory secretions, also should be obtained, as long as this does not delay the use of antibiotics.[10]
Within six hours, if blood pressure remains low despite initial fluid resuscitation of 30 ml/kg, or if initial lactate is ≥ four mmol/l (36 mg/dl), central venous pressure and central venous oxygen saturation should be measured.[10] Lactate should be re-measured if the initial lactate was elevated.[10] Evidence for point of care lactate measurement over usual methods of measurement, however, is poor.[28]
Within twelve hours, it is essential to diagnose or exclude any source of infection that would require emergent source control, such as a necrotizing soft tissue infection, an infection causing inflammation of the abdominal cavity lining, an infection of the bile duct, or an intestinal infarction.[10] A pierced internal organ (free air on an abdominal x-ray or CT scan), an abnormal chest x-ray consistent with pneumonia (with focal opacification), or petechiae, purpura, or purpura fulminans may indicate the presence of an infection.
Definitions
Finding | Value |
---|---|
Temperature | <36 °C (96.8 °F) or >38 °C (100.4 °F) |
Heart rate | >90/min |
Respiratory rate | >20/min or PaCO2<32 mmHg (4.3 kPa) |
WBC | <4x109/L (<4000/mm³), >12x109/L (>12,000/mm³), or ≥10% bands |
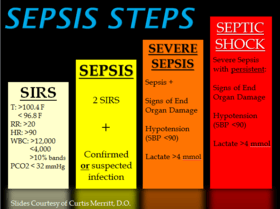
Previously, SIRS criteria had been used to define sepsis. If the SIRS criteria are negative, it is very unlikely the person has sepsis; if it is positive, there is just a moderate probability that the person has sepsis. According to SIRS, there were different levels of sepsis: sepsis, severe sepsis, and septic shock.[16] The definition of SIRS is shown below:
- SIRS is the presence of two or more of the following: abnormal body temperature, heart rate, respiratory rate, or blood gas, and white blood cell count.
- Sepsis is defined as SIRS in response to an infectious process.[30]
- Severe sepsis is defined as sepsis with sepsis-induced organ dysfunction or tissue hypoperfusion (manifesting as hypotension, elevated lactate, or decreased urine output). Severe sepsis is an infectious disease state associated with multiple organ dysfunction syndrome (MODS)[10]
- Septic shock is severe sepsis plus persistently low blood pressure, despite the administration of intravenous fluids.[10]
In 2016 a new consensus was reached to replace screening by systemic inflammatory response syndrome (SIRS) with the sequential organ failure assessment (SOFA score) and the abbreviated version (qSOFA).[5] The three criteria for the qSOFA score include a respiratory rate greater than or equal to 22 breaths per minute, systolic blood pressure 100 mmHg or less and altered mental status.[5] Sepsis is suspected when 2 of the qSOFA criteria are met.[5] The SOFA score was intended to be used in the intensive care unit (ICU) where it is administered upon admission to the ICU and then repeated every 48 hours, whereas the qSOFA could be used outside the ICU.[19] Some advantages of the qSOFA score are that it can be administered quickly and does not require labs.[19] However, the American College of Chest Physicians (CHEST) raised concerns that qSOFA and SOFA criteria may lead to delayed diagnosis of serious infection, leading to delayed treatment.[31] Although SIRS criteria can be too sensitive and not specific enough in identifying sepsis, SOFA also has its limitations and is not intended to replace the SIRS definition.[32] qSOFA has also been found to be poorly sensitive though decently specific for the risk of death with SIRS possibly better for screening.[33]
End-organ dysfunction
Examples of end-organ dysfunction include the following:[34]
- Lungs: acute respiratory distress syndrome (ARDS) (PaO2/FiO2 ratio< 300), different ratio in pediatric acute respiratory distress syndrome
- Brain: encephalopathy symptoms including agitation, confusion, coma; causes may include ischemia, bleeding, formation of blood clots in small blood vessels, microabscesses, multifocal necrotizing leukoencephalopathy
- Liver: disruption of protein synthetic function manifests acutely as progressive disruption of blood clotting due to an inability to synthesize clotting factors and disruption of metabolic functions leads to impaired bilirubin metabolism, resulting in elevated unconjugated serum bilirubin levels
- Kidney: low urine output or no urine output, electrolyte abnormalities, or volume overload
- Heart: systolic and diastolic heart failure, likely due to chemical signals that depress myocyte function, cellular damage, manifest as a troponin leak (although not necessarily ischemic in nature)
More specific definitions of end-organ dysfunction exist for SIRS in pediatrics.[35]
- Cardiovascular dysfunction (after fluid resuscitation with at least 40 ml/kg of crystalloid)
- hypotension with blood pressure < 5th percentile for age or systolic blood pressure < 2 standard deviations below normal for age, or
- vasopressor requirement, or
- two of the following criteria:
- unexplained metabolic acidosis with base deficit > 5 mEq/l
- lactic acidosis: serum lactate 2 times the upper limit of normal
- oliguria (urine output < 0.5 ml/kg/h)
- prolonged capillary refill > 5 seconds
- core to peripheral temperature difference > 3 °C
- Respiratory dysfunction (in the absence of a cyanotic heart defect or a known chronic respiratory disease)
- the ratio of the arterial partial-pressure of oxygen to the fraction of oxygen in the gases inspired (PaO2/FiO2) < 300 (the definition of acute lung injury), or
- arterial partial-pressure of carbon dioxide (PaCO2) > 65 torr (20 mmHg) over baseline PaCO2 (evidence of hypercapnic respiratory failure), or
- supplemental oxygen requirement of greater than FiO2 0.5 to maintain oxygen saturation ≥ 92%
- Neurologic dysfunction
- Glasgow Coma Score (GCS) ≤ 11, or
- altered mental status with drop in GCS of 3 or more points in a person with developmental delay/intellectual disability
- Hematologic dysfunction
- platelet count < 80,000/mm3 or 50% drop from maximum in chronically thrombocytopenic, or
- international normalized ratio (INR) > 2
- Disseminated intravascular coagulation
- Kidney dysfunction
- serum creatinine ≥ 2 times the upper limit of normal for age or 2-fold increase in baseline creatinine in people with chronic kidney disease
- Liver dysfunction (only applicable to infants > 1 month)
- total serum bilirubin ≥ 4 mg/dl, or
- alanine aminotransferase (ALT) ≥ 2 times the upper limit of normal
Consensus definitions, however, continue to evolve, with the latest expanding the list of signs and symptoms of sepsis to reflect clinical bedside experience.[17]
Biomarkers
A 2013 review concluded moderate-quality evidence exists to support the use of the procalcitonin level as a method to distinguish sepsis from non-infectious causes of SIRS.[27] The same review found the sensitivity of the test to be 77% and the specificity to be 79%. The authors suggested that procalcitonin may serve as a helpful diagnostic marker for sepsis, but cautioned that its level alone does not definitively make the diagnosis.[27] A 2012 systematic review found that soluble urokinase-type plasminogen activator receptor (SuPAR) is a nonspecific marker of inflammation and does not accurately diagnose sepsis.[36] This same review concluded, however, that SuPAR has prognostic value, as higher SuPAR levels are associated with an increased rate of death in those with sepsis.[36] Serial measurement of lactate levels (approximately every 4 to 6 hours) may guide treatment and is associated with lower mortality in sepsis.[19]
Differential diagnosis
The differential diagnosis for sepsis is broad and has to examine (to exclude) the non-infectious conditions that may cause the systemic signs of SIRS: alcohol withdrawal, acute pancreatitis, burns, pulmonary embolism, thyrotoxicosis, anaphylaxis, adrenal insufficiency, and neurogenic shock.[18][37] Hyperinflammatory syndromes such as hemophagocytic lymphohistiocytosis (HLH) may have similar symptoms and are on the differential diagnosis.[38]
Neonatal sepsis
In common clinical usage, neonatal sepsis refers to a bacterial blood stream infection in the first month of life, such as meningitis, pneumonia, pyelonephritis, or gastroenteritis,[39] but neonatal sepsis also may be due to infection with fungi, viruses, or parasites.[39] Criteria with regard to hemodynamic compromise or respiratory failure are not useful because they present too late for intervention.
Pathophysiology
Sepsis is caused by a combination of factors related to the particular invading pathogen(s) and to the status of the immune system of the host.[40] The early phase of sepsis characterized by excessive inflammation (sometimes resulting in a cytokine storm) may be followed by a prolonged period of decreased functioning of the immune system.[41][9] Either of these phases may prove fatal. On the other hand, systemic inflammatory response syndrome (SIRS) occurs in people without the presence of infection, for example, in those with burns, polytrauma, or the initial state in pancreatitis and chemical pneumonitis. However, sepsis also causes similar response to SIRS.[16]
Microbial factors
Bacterial virulence factors, such as glycocalyx and various adhesins, allow colonization, immune evasion, and establishment of disease in the host.[40] Sepsis caused by gram-negative bacteria is thought to be largely due to a response by the host to the lipid A component of lipopolysaccharide, also called endotoxin.[42][43] Sepsis caused by gram-positive bacteria may result from an immunological response to cell wall lipoteichoic acid.[44] Bacterial exotoxins that act as superantigens also may cause sepsis.[40] Superantigens simultaneously bind major histocompatibility complex and T-cell receptors in the absence of antigen presentation. This forced receptor interaction induces the production of pro-inflammatory chemical signals (cytokines) by T-cells.[40]
There are a number of microbial factors that may cause the typical septic inflammatory cascade. An invading pathogen is recognized by its pathogen-associated molecular patterns (PAMPs). Examples of PAMPs include lipopolysaccharides and flagellin in gram-negative bacteria, muramyl dipeptide in the peptidoglycan of the gram-positive bacterial cell wall, and CpG bacterial DNA. These PAMPs are recognized by the pattern recognition receptors (PRRs) of the innate immune system, which may be membrane-bound or cytosolic.[45] There are four families of PRRs: the toll-like receptors, the C-type lectin receptors, the NOD-like receptors, and the RIG-I-like receptors. Invariably, the association of a PAMP and a PRR will cause a series of intracellular signalling cascades. Consequentially, transcription factors such as nuclear factor-kappa B and activator protein-1, will up-regulate the expression of pro-inflammatory and anti-inflammatory cytokines.[46]
Host factors
Upon detection of microbial antigens, the host systemic immune system is activated. Immune cells not only recognise pathogen-associated molecular patterns but also damage-associated molecular patterns from damaged tissues. An uncontrolled immune response is then activated because leukocytes are not recruited to the specific site of infection, but instead they are recruited all over the body. Then, an immunosuppression state ensues when the proinflammatory T helper cell 1 (TH1) is shifted to TH2,[47] mediated by interleukin 10, which is known as "compensatory anti-inflammatory response syndrome".[22] The apoptosis (cell death) of lymphocytes further worsens the immunosuppression. Neutrophils, monocytes, macrophages, dendritic cells, CD4+ T cells, and B cells all undergo apoptosis, whereas regulatory T cells are more apoptosis resistant.[9] Subsequently, multiple organ failure ensues because tissues are unable to use oxygen efficiently due to inhibition of cytochrome c oxidase.[47]
Inflammatory responses cause multiple organ dysfunction syndrome through various mechanisms as described below. Increased permeability of the lung vessels causes leaking of fluids into alveoli, which results in pulmonary edema and acute respiratory distress syndrome (ARDS). Impaired utilization of oxygen in the liver impairs bile salt transport, causing jaundice (yellowish discoloration of skin). In kidneys, inadequate oxygenation results in tubular epithelial cell injury (of the cells lining the kidney tubules), and thus causes acute kidney injury (AKI). Meanwhile, in the heart, impaired calcium transport, and low production of adenosine triphosphate (ATP), can cause myocardial depression, reducing cardiac contractility and causing heart failure. In the gastrointestinal tract, increased permeability of the mucosa alters the microflora, causing mucosal bleeding and paralytic ileus. In the central nervous system, direct damage of the brain cells and disturbances of neurotransmissions causes altered mental status.[48] Cytokines such as tumor necrosis factor, interleukin 1, and interleukin 6 may activate procoagulation factors in the cells lining blood vessels, leading to endothelial damage. The damaged endothelial surface inhibits anticoagulant properties as well as increases antifibrinolysis, which may lead to intravascular clotting, the formation of blood clots in small blood vessels, and multiple organ failure.[49]
The low blood pressure seen in those with sepsis is the result of various processes, including excessive production of chemicals that dilate blood vessels such as nitric oxide, a deficiency of chemicals that constrict blood vessels such as vasopressin, and activation of ATP-sensitive potassium channels.[50] In those with severe sepsis and septic shock, this sequence of events leads to a type of circulatory shock known as distributive shock.[51]
Management
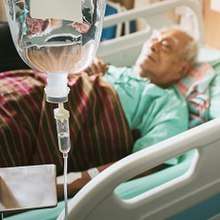
Early recognition and focused management may improve the outcomes in sepsis. Current professional recommendations include a number of actions ("bundles") to be followed as soon as possible after diagnosis. Within the first three hours, someone with sepsis should have received antibiotics and, intravenous fluids if there is evidence of either low blood pressure or other evidence for inadequate blood supply to organs (as evidenced by a raised level of lactate); blood cultures also should be obtained within this time period. After six hours the blood pressure should be adequate, close monitoring of blood pressure and blood supply to organs should be in place, and the lactate should be measured again if initially it was raised.[10] A related bundle, the "Sepsis Six", is in widespread use in the United Kingdom; this requires the administration of antibiotics within an hour of recognition, blood cultures, lactate and hemoglobin determination, urine output monitoring, high-flow oxygen, and intravenous fluids.[52][53]
Apart from the timely administration of fluids and antibiotics, the management of sepsis also involves surgical drainage of infected fluid collections and appropriate support for organ dysfunction. This may include hemodialysis in kidney failure, mechanical ventilation in lung dysfunction, transfusion of blood products, and drug and fluid therapy for circulatory failure. Ensuring adequate nutrition—preferably by enteral feeding, but if necessary, by parenteral nutrition—is important during prolonged illness.[10] Medication to prevent deep vein thrombosis and gastric ulcers also may be used.[10]
Antibiotics
Two sets of blood cultures (aerobic and anaerobic) are recommended without delaying the initiation of antibiotics. Cultures from other sites such as respiratory secretions, urine, wounds, cerebrospinal fluid, and catheter insertion sites (in-situ more than 48 hours) are recommended if infections from these sites are suspected.[6] In severe sepsis and septic shock, broad-spectrum antibiotics (usually two, a β-lactam antibiotic with broad coverage, or broad-spectrum carbapenem combined with fluoroquinolones, macrolides, or aminoglycosides) are recommended. The choice of antibiotics is important in determining the survival of the person.[51][6] Some recommend they be given within one hour of making the diagnosis, stating that for every hour of delay in the administration of antibiotics, there is an associated 6% rise in mortality.[30][51] Others did not find a benefit with early administration.[54]
Several factors determine the most appropriate choice for the initial antibiotic regimen. These factors include local patterns of bacterial sensitivity to antibiotics, whether the infection is thought to be a hospital or community-acquired infection, and which organ systems are thought to be infected.[51][19] Antibiotic regimens should be reassessed daily and narrowed if appropriate. Treatment duration is typically 7–10 days with the type of antibiotic used directed by the results of cultures. If the culture result is negative, antibiotics should be de-escalated according to person's clinical response or stopped altogether if infection is not present to decrease the chances that the person is infected with multiple drug resistance organisms. In case of people having high risk of being infected with multiple drug resistance organisms such as Pseudomonas aeruginosa, Acinetobacter baumannii, addition of antibiotic specific to gram-negative organism is recommended. For Methicillin-resistant Staphylococcus aureus (MRSA), vancomycin or teicoplanin is recommended. For Legionella infection, addition of macrolide or fluoroquinolone is chosen. If fungal infection is suspected, an echinocandin, such as caspofungin or micafungin, is chosen for people with severe sepsis, followed by triazole (fluconazole and itraconazole) for less ill people.[6] Prolonged antibiotic prophylaxis is not recommended in people who has SIRS without any infectious origin such as acute pancreatitis and burns unless sepsis is suspected.[6]
Once daily dosing of aminoglycoside is sufficient to achieve peak plasma concentration for clinical response without kidney toxicity. Meanwhile, for antibiotics with low volume distribution (vancomycin, teicoplanin, colistin), loading dose is required to achieve adequate therapeutic level to fight infections. Frequent infusions of beta-lactam antibiotics without exceeding total daily dose would help to keep the antibiotics level above minimum inhibitory concentration (MIC), thus providing better clinical response.[6] Giving beta-lactam antibiotics continuously may be better than giving them intermittently.[55] Access to therapeutic drug monitoring is important to ensure adequate drug therapeutic level while at the same time preventing the drug from reaching toxic level.[6]
Intravenous fluids
The Surviving Sepsis Campaign has recommended 30 ml/kg of fluid to be given in adults in the first three hours followed by fluid titration according to blood pressure, urine output, respiratory rate, and oxygen saturation with a target mean arterial pressure (MAP) of 65 mmHg.[6] In children an initial amount of 20 ml/kg is reasonable in shock.[56] In cases of severe sepsis and septic shock where a central venous catheter is used to measure blood pressures dynamically, fluids should be administered until the central venous pressure reaches 8–12 mmHg.[50] Once these goals are met, the central venous oxygen saturation (ScvO2), i.e., the oxygen saturation of venous blood as it returns to the heart as measured at the vena cava, is optimized.[6] If the ScvO2 is less than 70%, blood may be given to reach a hemoglobin of 10 g/dL and then inotropes are added until the ScvO2 is optimized.[40] In those with acute respiratory distress syndrome (ARDS) and sufficient tissue blood fluid, more fluids should be given carefully.[10]
Crystalloid solution is recommended as the fluid of choice for resuscitation.[6] Albumin can be used if a large amount of crystalloid is required for resuscitation.[6] Crystalloid solutions shows little difference with hydroxyethyl starch in terms of risk of death.[57] Starches also carry an increased risk of acute kidney injury,[57][58] and need for blood transfusion.[59][60] Various colloid solutions (such as modified gelatin) carry no advantage over crystalloid.[57] Albumin also appears to be of no benefit over crystalloids.[61]
Blood products
The Surviving Sepsis Campaign recommended packed red blood cells transfusion for hemoglobin levels below 70 g/L if there is no myocardial ischemia, hypoxemia, or acute bleeding.[6] In a 2014 trial, blood transfusions to keep target hemoglobin above 70 or 90 g/L did not make any difference to survival rates; meanwhile, those with a lower threshold of transfusion received fewer transfusions in total.[62] Erythropoietin is not recommended in the treatment of anemia with septic shock because it may precipitate blood clotting events. Fresh frozen plasma transfusion usually does not correct the underlying clotting abnormalities before a planned surgical procedure. However, platelet transfusion is suggested for platelet counts below (10 × 109/L) without any risk of bleeding, or (20 × 109/L) with high risk of bleeding, or (50 × 109/L) with active bleeding, before a planned surgery or an invasive procedure.[6] IV immunoglobulin is not recommended because its beneficial effects are uncertain.[6] Monoclonal and polyclonal preparations of intravenous immunoglobulin (IVIG) do not lower the rate of death in newborns and adults with sepsis.[63] Evidence for the use of IgM-enriched polyclonal preparations of IVIG is inconsistent.[63] On the other hand, the use of antithrombin to treat disseminated intravascular coagulation is also not useful. Meanwhile, the blood purification technique (such as hemoperfusion, plasma filtration, and coupled plasma filtration adsorption) to remove inflammatory mediators and bacterial toxins from the blood also does not demonstrate any survival benefit for septic shock.[6]
Vasopressors
If the person has been sufficiently fluid resuscitated but the mean arterial pressure is not greater than 65 mmHg, vasopressors are recommended.[6] Norepinephrine (noradrenaline) is recommended as the initial choice.[6] Delaying initiation of vasopressor therapy during septic shock is associated with increased mortality.[64]
Norepinephrine is often used as a first-line treatment for hypotensive septic shock because evidence shows that there is a relative deficiency of vasopressin, when shock continues for 24 to 48 hours.[65] Norepinephrine raises blood pressure through a vasoconstriction effect, with little effect on stroke volume and heart rate.[6] In some people, the required dose of vasopressor needed to increase the mean arterial pressure can become exceedingly high that it becomes toxic.[66] In order to reduce the required dose of vasopressor, epinephrine may be added.[66] Epinephrine is not often used as a first-line treatment for hypotensive shock because it reduces blood flow to the abdominal organs and increases lactate levels.[65] Vasopressin can be used in septic shock because studies have shown that there is a relative deficiency of vasopressin when shock continues for 24 to 48 hours. However, vasopressin reduces blood flow to the heart, finger/toes, and abdominal organs, resulting in a lack of oxygen supply to these tissues.[6] Dopamine is typically not recommended. Although dopamine is useful to increase the stroke volume of the heart, it causes more abnormal heart rhythms than norepinephrine and also has an immunosuppressive effect. Dopamine is not proven to have protective properties on the kidneys.[6] Dobutamine can also be used in hypotensive septic shock to increase cardiac output and correct blood flow to the tissues.[67] Dobutamine is not used as often as epinephrine due to its associate side effects, which include reducing blood flow to the gut.[67] Additionally, dobutamine increases the cardiac output by abnormally increasing the heart rate.[67]
Steroids
The use of steroids in sepsis is controversial.[68] Studies do not give a clear picture as to whether and when glucocorticoids should be used.[69] The 2016 Surviving Sepsis Campaign recommends low dose hydrocortisone only if both intravenous fluids and vasopressors are not able to adequately treat septic shock.[6] A 2019 Cochrane review found low-quality evidence of benefit,[11] as did two 2019 reviews.[12][70]
During critical illness, a state of adrenal insufficiency and tissue resistance to corticosteroids may occur. This has been termed critical illness–related corticosteroid insufficiency.[71] Treatment with corticosteroids might be most beneficial in those with septic shock and early severe ARDS, whereas its role in others such as those with pancreatitis or severe pneumonia is unclear.[71] However, the exact way of determining corticosteroid insufficiency remains problematic. It should be suspected in those poorly responding to resuscitation with fluids and vasopressors. Neither ACTH stimulation testing[71] nor random cortisol levels are recommended to confirm the diagnosis.[6] The method of stopping glucocorticoid drugs is variable, and it is unclear whether they should be slowly decreased or simply abruptly stopped. However, the 2016 Surviving Sepsis Campaign recommended to taper steroids when vasopressors are no longer needed.[6]
Anesthesia
A target tidal volume of 6 mL/kg of predicted body weight (PBW) and a plateau pressure less than 30 cm H2O is recommended for those who require ventilation due to sepsis-induced severe ARDS. High positive end expiratory pressure (PEEP) is recommended for moderate to severe ARDS in sepsis as it opens more lung units for oxygen exchange. Predicted body weight is calculated based on sex and height, and tools for this are available.[72] Recruitment maneuvers may be necessary for severe ARDS by briefly raising the transpulmonary pressure. It is recommended that the head of the bed be raised if possible to improve ventilation. However, β2 adrenergic receptor agonists are not recommended to treat ARDS because it may reduce survival rates and precipitate abnormal heart rhythms. A spontaneous breathing trial using continuous positive airway pressure (CPAP), T piece, or inspiratory pressure augmentation can be helpful in reducing the duration of ventilation. Minimizing intermittent or continuous sedation is helpful in reducing the duration of mechanical ventilation.[6]
General anesthesia is recommended for people with sepsis who require surgical procedures to remove the infective source. Usually, inhalational and intravenous anesthetics are used. Requirements for anesthetics may be reduced in sepsis. Inhalational anesthetics can reduce the level of proinflammatory cytokines, altering leukocyte adhesion and proliferation, inducing apoptosis (cell death) of the lymphocytes, possibly with a toxic effect on mitochondrial function.[47] Although etomidate has a minimal effect on the cardiovascular system, it is often not recommended as a medication to help with intubation in this situation due to concerns it may lead to poor adrenal function and an increased risk of death.[73][74] The small amount of evidence there is, however, has not found a change in the risk of death with etomidate.[75]
Paralytic agents are not suggested for use in sepsis cases in the absence of ARDS, as a growing body of evidence points to reduced durations of mechanical ventilation, ICU and hospital stays.[10] However, paralytic use in ARDS cases remains controversial. When appropriately used, paralytics may aid successful mechanical ventilation, however evidence has also suggested that mechanical ventilation in severe sepsis does not improve oxygen consumption and delivery.[10]
Source control
Source control refers to physical interventions to control a focus of infection and reduce conditions favorable to microorganism growth or host defense impairment, such as drainage of pus from an abscess. It is one of the oldest procedures for control of infections, giving rise to the Latin phrase Ubi pus, ibi evacua, and remains important despite the emergence of more modern treatments.[76][77]
Early goal directed therapy
Early goal directed therapy (EGDT) is an approach to the management of severe sepsis during the initial 6 hours after diagnosis.[78] It is a step-wise approach, with the physiologic goal of optimizing cardiac preload, afterload, and contractility.[79] It includes giving early antibiotics.[79] EGDT also involves monitoring of hemodynamic parameters and specific interventions to achieve key resuscitation targets which include maintaining a central venous pressure between 8–12 mmHg, a mean arterial pressure of between 65–90 mmHg, a central venous oxygen saturation (ScvO2) greater than 70% and a urine output of greater than 0.5 ml/kg/hour. The goal is to optimize oxygen delivery to tissues and achieve a balance between systemic oxygen delivery and demand.[79] An appropriate decrease in serum lactate may be equivalent to ScvO2 and easier to obtain.[80]
In the original trial, early goal directed therapy was found to reduce mortality from 46.5% to 30.5% in those with sepsis,[79] and the Surviving Sepsis Campaign has been recommending its use.[10] However, three more recent large randomized control trials (ProCESS, ARISE, and ProMISe), did not demonstrate a 90-day mortality benefit of early goal directed therapy when compared to standard therapy in severe sepsis.[81] It is likely that some parts of EGDT are more important than others.[81] Following these trials the use of EGDT is still considered reasonable.[82]
Newborns
Neonatal sepsis can be difficult to diagnose as newborns may be asymptomatic.[83] If a newborn shows signs and symptoms suggestive of sepsis, antibiotics are immediately started and are either changed to target a specific organism identified by diagnostic testing or discontinued after an infectious cause for the symptoms has been ruled out.[84] Despite early intervention, death occurs in 13% of children who develop septic shock, with the risk partly based on other health problems. Those without multiple organ system failure or who require only one inotropic agent mortality is low.[85]
Other
Treating fever in sepsis, including people in septic shock, has not been associated with any improvement in mortality over a period of 28 days.[86] Treatment of fever still occurs for other reasons.[87][88]
A 2012 Cochrane review concluded that N-acetylcysteine does not reduce mortality in those with SIRS or sepsis and may even be harmful.[89]
Recombinant activated protein C (drotrecogin alpha) was originally introduced for severe sepsis (as identified by a high APACHE II score), where it was thought to confer a survival benefit.[78] However, subsequent studies showed that it increased adverse events—bleeding risk in particular—and did not decrease mortality.[90] It was removed from sale in 2011.[90] Another medication known as eritoran also has not shown benefit.[91]
In those with high blood sugar levels, insulin to bring it down to 7.8–10 mmol/L (140–180 mg/dL) is recommended with lower levels potentially worsening outcomes.[92] Glucose levels taken from capillary blood should be interpreted with care because such measurements may not be accurate. If a person has an arterial catheter, arterial blood is recommended for blood glucose testing.[6]
Intermittent or continuous renal replacement therapy may be used if indicated. However, sodium bicarbonate is not recommended for a person with lactic acidosis secondary to hypoperfusion. Low-molecular-weight heparin (LMWH), unfractionated heparin (UFH), and mechanical prophylaxis with intermittent pneumatic compression devices are recommended for any person with sepsis at moderate to high risk of venous thromboembolism.[6] Stress ulcer prevention with proton-pump inhibitor (PPI) and H2 antagonist are useful in a person with risk factors of developing upper gastrointestinal bleeding (UGIB) such as on mechanical ventilation for more than 48 hours, coagulation disorders, liver disease, and renal replacement therapy.[6] Achieving partial or full enteral feeding (delivery of nutrients through a feeding tube) is chosen as the best approach to provide nutrition for a person who is contraindicated for oral intake or unable to tolerate orally in the first seven days of sepsis when compared to intravenous nutrition. However, omega-3 fatty acids are not recommended as immune supplements for a person with sepsis or septic shock. The usage of prokinetic agents such as metoclopramide, domperidone, and erythromycin are recommended for those who are septic and unable to tolerate enteral feeding. However, these agents may precipitate prolongation of the QT interval and consequently provoke a ventricular arrhythmia such as torsades de pointes. The usage of prokinetic agents should be reassessed daily and stopped if no longer indicated.[6]
Prognosis
Severe sepsis will prove fatal in approximately 20–35% of people, and septic shock will prove fatal in 30–70% of people.[93] Lactate is a useful method of determining prognosis, with those who have a level greater than 4 mmol/L having a mortality of 40% and those with a level of less than 2 mmol/L having a mortality of less than 15%.[30]
There are a number of prognostic stratification systems, such as APACHE II and Mortality in Emergency Department Sepsis. APACHE II factors in the person's age, underlying condition, and various physiologic variables to yield estimates of the risk of dying of severe sepsis. Of the individual covariates, the severity of underlying disease most strongly influences the risk of death. Septic shock is also a strong predictor of short- and long-term mortality. Case-fatality rates are similar for culture-positive and culture-negative severe sepsis. The Mortality in Emergency Department Sepsis (MEDS) score is simpler, and useful in the emergency department environment.[94]
Some people may experience severe long-term cognitive decline following an episode of severe sepsis, but the absence of baseline neuropsychological data in most people with sepsis makes the incidence of this difficult to quantify or to study.[95]
Epidemiology
Sepsis causes millions of deaths globally each year and is the most common cause of death in people who have been hospitalized.[4][78] The number of new cases worldwide of sepsis is estimated to be 18 million cases per year.[96] In the United States sepsis affects approximately 3 in 1,000 people,[30] and severe sepsis contributes to more than 200,000 deaths per year.[97]
Sepsis occurs in 1–2% of all hospitalizations and accounts for as much as 25% of ICU bed utilization. Due to it rarely being reported as a primary diagnosis (often being a complication of cancer or other illness), the incidence, mortality, and morbidity rates of sepsis are likely underestimated.[40] A study of U.S. states found approximately 651 hospital stays per 100,000 population with a sepsis diagnosis in 2010.[98] It is the second-leading cause of death in non-coronary intensive care unit (ICU) and the tenth-most-common cause of death overall (the first being heart disease).[99] Children under 12 months of age and elderly people have the highest incidence of severe sepsis.[40] Among people from the U.S. who had multiple sepsis hospital admissions in 2010, those who were discharged to a skilled nursing facility or long-term care following the initial hospitalization were more likely to be readmitted than those discharged to another form of care.[98] A study of 18 U.S. states found that, amongst people with Medicare in 2011, sepsis was the second most common principal reason for readmission within 30 days.[100]
Several medical conditions increase a person's susceptibility to infection and developing sepsis. Common sepsis risk factors include age (especially the very young and old); conditions that weaken the immune system such as cancer, diabetes, or the absence of a spleen; and major trauma and burns.[2][101][102]
From 1979 to 2000, data from the United States National Hospital Discharge Survey showed that the incidence of sepsis increased fourfold, to 240 cases per 100,000 population, with higher incidence in men when compared to women. During the same time frame, the in-hospital case fatality rate was reduced from 28% to 18%. However, according to the nationwide inpatient sample from the United States, the incidence of severe sepsis increased from 200 per 10,000 population in 2003 to 300 cases in 2007 for population aged more than 18 years. The incidence rate is particularly high among infants, with the incidence of 500 cases per 100,000 population. Mortality related to sepsis increases with age, from less than 10% in the age group of 3 to 5 years to 60% by sixth decade of life.[21] The increase in average age of the population, alongside the presence of more people with chronic diseases or on immunosuppressive medications, and also the increase in the number of invasive procedures being performed, has led to an increased rate of sepsis.[22]
History

The term "σήψις" (sepsis) was introduced by Hippocrates in the fourth century BC, and it meant the process of decay or decomposition of organic matter.[103][104][105] In the eleventh century, Avicenna used the term "blood rot" for diseases linked to severe purulent process. Though severe systemic toxicity had already been observed, it was only in the 19th century that the specific term – sepsis – was used for this condition.
The terms "septicemia", also spelled "septicaemia", and "blood poisoning" referred to the microorganisms or their toxins in the blood. The International Statistical Classification of Diseases and Related Health Problems (ICD) version 9, which was in use in the US until 2013, used the term septicemia with numerous modifiers for different diagnoses, such as "Streptococcal septicemia".[106] All those diagnoses have been converted to sepsis, again with modifiers, in ICD-10, such as "Sepsis due to streptococcus".[106]
The current terms are dependent on the microorganism that is present: bacteremia if bacteria are present in the blood at abnormal levels and are the causative issue, viremia for viruses, and fungemia for a fungus.[107]
By the end of the 19th century, it was widely believed that microbes produced substances that could injure the mammalian host and that soluble toxins released during infection caused the fever and shock that were commonplace during severe infections. Pfeiffer coined the term endotoxin at the beginning of the 20th century to denote the pyrogenic principle associated with Vibrio cholerae. It was soon realised that endotoxins were expressed by most and perhaps all gram-negative bacteria. The lipopolysaccharide character of enteric endotoxins was elucidated in 1944 by Shear.[108] The molecular character of this material was determined by Luderitz et al. in 1973.[109]
It was discovered in 1965 that a strain of C3H/HeJ mice were immune to the endotoxin-induced shock.[110] The genetic locus for this effect was dubbed Lps. These mice were also found to be hypersusceptible to infection by gram-negative bacteria.[111] These observations were finally linked in 1998 by the discovery of the toll-like receptor gene 4 (TLR 4).[112] Genetic mapping work, performed over a period of five years, showed that TLR4 was the sole candidate locus within the Lps critical region; this strongly implied that a mutation within TLR4 must account for the lipopolysaccharide resistance phenotype. The defect in the TLR4 gene that led to the endotoxin resistant phenotype was discovered to be due to a mutation in the cytoplasm.[113]
Controversy occurred in the scientific community over the use of mouse models in research into sepsis in 2013, when scientists published a review of the mouse immune system compared to the human immune system, and showed that on a systems level, the two worked very differently; the authors noted that as of the date of their article over 150 clinical trials of sepsis had been conducted in humans, almost all of them supported by promising data in mice, and that all of them had failed. The authors called for abandoning the use of mouse models in sepsis research; others rejected that but called for more caution in interpreting the results of mouse studies[114], and more careful design of preclinical studies.[115][116][117][118] One approach is to rely more on studying biopsies and clinical data from people who have had sepsis, to try to identify biomarkers and drug targets for intervention.[119]
Society and culture
Economics
Sepsis was the most expensive condition treated in United States' hospital stays in 2013, at an aggregate cost of $23.6 billion for nearly 1.3 million hospitalizations.[120] Costs for sepsis hospital stays more than quadrupled since 1997 with an 11.5 percent annual increase.[121] By payer, it was the most costly condition billed to Medicare and the uninsured, the second-most costly billed to Medicaid, and the fourth-most costly billed to private insurance.[120]
Education
A large international collaboration entitled the "Surviving Sepsis Campaign" was established in 2002[122] to educate people about sepsis and to improve outcomes with sepsis. The Campaign has published an evidence-based review of management strategies for severe sepsis, with the aim to publish a complete set of guidelines in subsequent years.[78]
Sepsis Alliance is a charitable organization that was created to raise sepsis awareness among both the general public and healthcare professionals.[123]
Research

Some authors suggest that initiating sepsis by the normally mutualistic (or neutral) members of the microbiome may not always be an accidental side effect of the deteriorating host immune system. Rather it is often an adaptive microbial response to a sudden decline of host survival chances. Under this scenario, the microbe species provoking sepsis benefit from monopolizing the future cadaver, utilizing its biomass as decomposers, and then transmitting through soil or water to establish mutualistic relations with new individuals. The bacteria Streptococcus pneumoniae, Escherichia coli, Proteus spp., Pseudomonas aeruginosa, Staphylococcus aureus, Klebsiella spp., Clostridium spp., Lactobacillus spp., Bacteroides spp. and the fungi Candida spp. are all capable of such a high level of phenotypic plasticity. Evidently, not all cases of sepsis arise through such adaptive microbial strategy switches.[124]
References
- "Blood Culture Collection" (PDF). WVUH Laboratories. 7 April 2012. Retrieved 23 March 2020.
- "Sepsis Questions and Answers". cdc.gov. Centers for Disease Control and Prevention (CDC). 22 May 2014. Archived from the original on 4 December 2014. Retrieved 28 November 2014.
- Jui J, et al. (American College of Emergency Physicians) (2011). "Ch. 146: Septic Shock". In Tintinalli JE, et al. (eds.). Tintinalli's Emergency Medicine: A Comprehensive Study Guide (7th ed.). New York: McGraw-Hill. pp. 1003–14. ISBN 9780071484800.
- Deutschman CS, Tracey KJ (April 2014). "Sepsis: Current dogma and new perspectives". Immunity. 40 (4): 463–75. doi:10.1016/j.immuni.2014.04.001. PMID 24745331.
- Singer M, Deutschman CS, et al. (February 2016). "The Third International Consensus Definitions for Sepsis and Septic Shock (Sepsis-3)". JAMA. 315 (8): 801–10. doi:10.1001/jama.2016.0287. PMC 4968574. PMID 26903338.
- Rhodes A, Evans LE, et al. (March 2017). "Surviving Sepsis Campaign: International Guidelines for Management of Sepsis and Septic Shock: 2016". Intensive Care Medicine. 43 (3): 304–377. doi:10.1007/s00134-017-4683-6. PMID 28101605.
- Jawad I, Lukšić I, et al. (June 2012). "Assessing available information on the burden of sepsis: Global estimates of incidence, prevalence and mortality". Journal of Global Health. 2 (1): 010404. doi:10.7189/jogh.01.010404. PMC 3484761. PMID 23198133.
- Martin GS (June 2012). "Sepsis, severe sepsis and septic shock: Changes in incidence, pathogens and outcomes". Expert Review of Anti-infective Therapy. 10 (6): 701–6. doi:10.1586/eri.12.50. PMC 3488423. PMID 22734959.
- Chao C, Muming Y, Yanfen C (2019). "Pathological Alteration and Therapeutic Implications of Sepsis-Induced Immune Cell Apoptosis". Cell Death & Disease. 10 (10): 782. doi:10.1038/s41419-019-2015-1. PMC 6791888. PMID 31611560.
- Dellinger RP, Levy MM, et al. (February 2013). "Surviving Sepsis Campaign: International Guidelines for Management of Severe Sepsis and Septic Shock: 2012". Critical Care Medicine. 41 (2): 580–637. doi:10.1097/CCM.0b013e31827e83af. PMID 23353941.
- Annane, D; Bellissant, E; Bollaert, PE; Briegel, J; Keh, D; Kupfer, Y; Pirracchio, R; Rochwerg, B (6 December 2019). "Corticosteroids for treating sepsis in children and adults". The Cochrane Database of Systematic Reviews. 12: CD002243. doi:10.1002/14651858.CD002243.pub4. PMC 6953403. PMID 31808551.
- Fang F, Zhang Y, et al. (February 2019). "Association of corticosteroid treatment with outcomes in adult patients with sepsis: A systematic review and meta-analysis". JAMA Internal Medicine. 179 (2): 213–223. doi:10.1001/jamainternmed.2018.5849. PMC 6439648. PMID 30575845.
- Long B, Koyfman A (November 2017). "Controversies in corticosteroid use for sepsis". The Journal of Emergency Medicine. 53 (5): 653–661. doi:10.1016/j.jemermed.2017.05.024. PMID 28916121.
- Rudd, Kristina E; Johnson, Sarah Charlotte; Agesa, Kareha M; Shackelford, Katya Anne; Tsoi, Derrick; Kievlan, Daniel Rhodes; Colombara, Danny V; Ikuta, Kevin S; Kissoon, Niranjan; Finfer, Simon; Fleischmann-Struzek, Carolin; Machado, Flavia R; Reinhart, Konrad K; Rowan, Kathryn; Seymour, Christopher W; Watson, R Scott; West, T Eoin; Marinho, Fatima; Hay, Simon I; Lozano, Rafael; Lopez, Alan D; Angus, Derek C; Murray, Christopher J L; Naghavi, Mohsen (January 2020). "Global, regional, and national sepsis incidence and mortality, 1990–2017: analysis for the Global Burden of Disease Study". The Lancet. 395 (10219): 200–211. doi:10.1016/S0140-6736(19)32989-7. PMC 6970225. PMID 31954465.
- Angus DC, van der Poll T (August 2013). "Severe sepsis and septic shock". The New England Journal of Medicine. 369 (9): 840–51. doi:10.1056/NEJMra1208623. PMID 23984731. Lay summary (30 August 2013).
- Bone RC, Balk RA, et al. (The ACCP/SCCM Consensus Conference Committee. American College of Chest Physicians/Society of Critical Care Medicine) (June 1992). "Definitions for sepsis and organ failure and guidelines for the use of innovative therapies in sepsis". Chest. 101 (6): 1644–55. doi:10.1378/chest.101.6.1644. PMID 1303622.
Septicemia... has been used... in a variety of ways... We therefore suggest that this term be eliminated from current usage.
- Levy MM, Fink MP, et al. (April 2003). "2001 SCCM/ESICM/ACCP/ATS/SIS International Sepsis Definitions Conference" (PDF). Critical Care Medicine. 31 (4): 1250–6. doi:10.1097/01.CCM.0000050454.01978.3B. PMID 12682500. Archived (PDF) from the original on 24 September 2015.
- Felner K, Smith RL (2012). "Ch. 138: Sepsis". In McKean S, et al. (eds.). Principles and Practice of Hospital Medicine. New York: McGraw-Hill. pp. 1099–109. ISBN 978-0071603898.
- Gauer, RL (1 July 2013). "Early recognition and management of sepsis in adults: the first six hours". American Family Physician. 88 (1): 44–53. PMID 23939605.
- MedlinePlus Encyclopedia: Sepsis. Retrieved 29 November 2014.
- Munford RS, Suffredini AF (2014). "Ch. 75: Sepsis, Severe Sepsis and Septic Shock". In Bennett JE, et al. (eds.). Mandell, Douglas, and Bennett's Principles and Practice of Infectious Diseases (8th ed.). Philadelphia: Elsevier Health Sciences. pp. 914–34. ISBN 9780323263733.
- Polat G, Ugan RA, et al. (February 2017). "Sepsis and septic shock: Current treatment strategies and new approaches". The Eurasian Journal of Medicine. 49 (1): 53–8. doi:10.5152/eurasianjmed.2017.17062. PMC 5389495. PMID 28416934.
- Bloch KC (2009). "Ch. 4: Infectious Diseases". In McPhee SJ, et al. (eds.). Pathophysiology of Disease (6th ed.). New York: McGraw-Hill. ISBN 9780071621670.
- Ramachandran G (January 2014). "Gram-positive and gram-negative bacterial toxins in sepsis: A brief review". Virulence. 5 (1): 213–8. doi:10.4161/viru.27024. PMC 3916377. PMID 24193365.
- Delaloye J, Calandra T (January 2014). "Invasive candidiasis as a cause of sepsis in the critically ill patient". Virulence. 5 (1): 161–9. doi:10.4161/viru.26187. PMC 3916370. PMID 24157707.
- Harris R (22 February 2018). "Synergy Between Nurses And Automation Could Be Key To Finding Sepsis Early". All Things Considered. NPR. Archived from the original on 27 February 2018. Retrieved 26 February 2018.
- Wacker C, Prkno A, et al. (May 2013). "Procalcitonin as a diagnostic marker for sepsis: A systematic review and meta-analysis". The Lancet Infectious Diseases. 13 (5): 426–35. doi:10.1016/S1473-3099(12)70323-7. PMID 23375419.
- Morris E, McCartney D, et al. (December 2017). "Point-of-care lactate testing for sepsis at presentation to health care: A systematic review of patient outcomes". The British Journal of General Practice. 67 (665): e859–70. doi:10.3399/bjgp17X693665. PMC 5697556. PMID 29158243.
- Bone RC, Balk RA, et al. (The ACCP/SCCM Consensus Conference Committee. American College of Chest Physicians/Society of Critical Care Medicine) (June 1992). "Definitions for sepsis and organ failure and guidelines for the use of innovative therapies in sepsis". Chest. 101 (6): 1644–55. doi:10.1378/chest.101.6.1644. PMID 1303622.
- Soong J, Soni N (June 2012). "Sepsis: Recognition and treatment". Clinical Medicine. 12 (3): 276–80. doi:10.7861/clinmedicine.12-3-276. PMC 4953494. PMID 22783783.
- Simpson SQ (May 2016). "New sepsis criteria: A change we should not make". Chest. 149 (5): 1117–8. doi:10.1016/j.chest.2016.02.653. PMID 26927525.
We believe that adopting a more restrictive definition that requires further progression along the sepsis pathway may delay intervention in this highly time-dependent condition, with additional risk to patients.
- Vincent JL, Martin GS, et al. (July 2016). "qSOFA does not replace SIRS in the definition of sepsis". Critical Care. 20 (1): 210. doi:10.1186/s13054-016-1389-z. PMC 4947518. PMID 27423462.
We hope this editorial will clarify that the qSOFA is meant to be used to raise suspicion of sepsis and prompt further action—it is not a replacement for SIRS and is not part of the definition of sepsis.
- Fernando SM, Tran A, et al. (February 2018). "Prognostic accuracy of the quick Sequential Organ Failure Assessment for mortality in patients with suspected infection: A systematic review and meta-analysis". Annals of Internal Medicine. 168 (4): 266–75. doi:10.7326/M17-2820. PMID 29404582. S2CID 3441582.
- Abraham E, Singer M (October 2007). "Mechanisms of sepsis-induced organ dysfunction". Critical Care Medicine. 35 (10): 2408–16. doi:10.1097/01.CCM.0000282072.56245.91. PMID 17948334. S2CID 12657048.
- Goldstein B, Giroir B, et al. (January 2005). "International pediatric sepsis consensus conference: Definitions for sepsis and organ dysfunction in pediatrics". Pediatric Critical Care Medicine. 6 (1): 2–8. doi:10.1097/01.PCC.0000149131.72248.E6. PMID 15636651.
- Backes Y, van der Sluijs KF, et al. (September 2012). "Usefulness of suPAR as a biological marker in patients with systemic inflammation or infection: A systematic review". Intensive Care Medicine. 38 (9): 1418–28. doi:10.1007/s00134-012-2613-1. PMC 3423568. PMID 22706919.
- Mayr FB, Yende S, et al. (January 2014). "Epidemiology of severe sepsis". Virulence. 5 (1): 4–11. doi:10.4161/viru.27372. PMC 3916382. PMID 24335434.
- Machowicz R, Janka G, et al. (June 2017). "Similar but not the same: Differential diagnosis of HLH and sepsis". Critical Reviews in Oncology/Hematology. 114: 1–12. doi:10.1016/j.critrevonc.2017.03.023. PMID 28477737.
- Satar M, Ozlü F (September 2012). "Neonatal sepsis: A continuing disease burden" (PDF). The Turkish Journal of Pediatrics. 54 (5): 449–57. PMID 23427506. Archived from the original (PDF) on 19 December 2014.
- Ely EW, Goyette RE (2005). "Ch. 46: Sepsis with Acute Organ Dysfunction". In Hall JB, et al. (eds.). Principles of Critical Care (3rd ed.). New York: McGraw-Hill Medical. ISBN 978-0071416405.
- Shukla P, Rao GM, et al. (November 2014). "Therapeutic interventions in sepsis: Current and anticipated pharmacological agents". British Journal of Pharmacology. 171 (22): 5011–31. doi:10.1111/bph.12829. PMC 4253453. PMID 24977655.
- Park BS, Lee JO (December 2013). "Recognition of lipopolysaccharide pattern by TLR4 complexes". Experimental & Molecular Medicine. 45 (12): e66. doi:10.1038/emm.2013.97. PMC 3880462. PMID 24310172.
- Cross AS (January 2014). "Anti-endotoxin vaccines: Back to the future". Virulence. 5 (1): 219–25. doi:10.4161/viru.25965. PMC 3916378. PMID 23974910.
- Fournier B, Philpott DJ (July 2005). "Recognition of Staphylococcus aureus by the innate immune system". Clinical Microbiology Reviews. 18 (3): 521–40. doi:10.1128/CMR.18.3.521-540.2005. PMC 1195972. PMID 16020688.
- Leentjens J, Kox M, et al. (June 2013). "Immunotherapy for the adjunctive treatment of sepsis: From immunosuppression to immunostimulation. Time for a paradigm change?". American Journal of Respiratory and Critical Care Medicine. 187 (12): 1287–93. doi:10.1164/rccm.201301-0036CP. PMID 23590272.
- Antonopoulou A, Giamarellos-Bourboulis EJ (January 2011). "Immunomodulation in sepsis: State of the art and future perspective". Immunotherapy. 3 (1): 117–28. doi:10.2217/imt.10.82. PMID 21174562.
- Yuki K, Murakami N (6 January 2016). "Sepsis pathophysiology and anesthetic consideration". Cardiovascular & Hematological Disorders Drug Targets. 15 (1): 57–69. doi:10.2174/1871529x15666150108114810. PMC 4704087. PMID 25567335.
- Fujishima S (1 November 2016). "Organ dysfunction as a new standard for defining sepsis". Inflammation and Regeneration. 36 (24): 24. doi:10.1186/s41232-016-0029-y. PMC 5725936. PMID 29259697.
- Nimah M, Brilli RJ (July 2003). "Coagulation dysfunction in sepsis and multiple organ system failure". Critical Care Clinics. 19 (3): 441–58. doi:10.1016/s0749-0704(03)00008-3. PMID 12848314.
- Marik PE (June 2014). "Iatrogenic salt water drowning and the hazards of a high central venous pressure". Annals of Intensive Care. 4: 21. doi:10.1186/s13613-014-0021-0. PMC 4122823. PMID 25110606.
- Marik PE (June 2014). "Early management of severe sepsis: Concepts and controversies". Chest. 145 (6): 1407–18. CiteSeerX 10.1.1.661.7518. doi:10.1378/chest.13-2104. PMID 24889440.
- Daniels R (April 2011). "Surviving the first hours in sepsis: Getting the basics right (an intensivist's perspective)" (PDF). Journal of Antimicrobial Chemotherapy. 66 (Suppl 2): ii11–23. doi:10.1093/jac/dkq515. PMID 21398303.
- Scottish Intercollegiate Guidelines Network (SIGN) (May 2014). Care of Deteriorating Patients (PDF). Guideline 139. Edinburgh: SIGN. ISBN 978-1-909103-26-9. Archived from the original (PDF) on 11 August 2014. Retrieved 6 December 2014.
- Sterling SA, Miller WR, et al. (September 2015). "The impact of timing of antibiotics on outcomes in severe sepsis and septic shock: A systematic review and meta-Analysis". Critical Care Medicine. 43 (9): 1907–15. doi:10.1097/CCM.0000000000001142. PMC 4597314. PMID 26121073.
- Roberts JA, Abdul-Aziz MH, et al. (September 2016). "Continuous versus intermittent β-Lactam infusion in severe sepsis. A meta-analysis of individual patient data from randomized trials". American Journal of Respiratory and Critical Care Medicine. 194 (6): 681–91. doi:10.1164/rccm.201601-0024oc. PMID 26974879.
- de Caen AR, Berg MD, et al. (November 2015). "Part 12: Pediatric Advanced Life Support: 2015 American Heart Association guidelines update for cardiopulmonary resuscitation and emergency cardiovascular care". Circulation. 132 (18 Suppl 2): S526–42. doi:10.1161/cir.0000000000000266. PMC 6191296. PMID 26473000.
- Lewis SR, Pritchard MW, et al. (August 2018). "Colloids versus crystalloids for fluid resuscitation in critically ill people". The Cochrane Database of Systematic Reviews. 8: CD000567. doi:10.1002/14651858.CD000567.pub7. PMC 6513027. PMID 30073665.
- Zarychanski R, Abou-Setta AM, et al. (February 2013). "Association of hydroxyethyl starch administration with mortality and acute kidney injury in critically ill patients requiring volume resuscitation: A systematic review and meta-analysis". JAMA. 309 (7): 678–88. doi:10.1001/jama.2013.430. PMID 23423413.
- Haase N, Perner A, et al. (February 2013). "Hydroxyethyl starch 130/0.38-0.45 versus crystalloid or albumin in patients with sepsis: Systematic review with meta-analysis and trial sequential analysis". BMJ. 346: f839. doi:10.1136/bmj.f839. PMC 3573769. PMID 23418281.
- Serpa Neto A, Veelo DP, et al. (February 2014). "Fluid resuscitation with hydroxyethyl starches in patients with sepsis is associated with an increased incidence of acute kidney injury and use of renal replacement therapy: A systematic review and meta-analysis of the literature". Journal of Critical Care. 29 (1): 185.e1–7. doi:10.1016/j.jcrc.2013.09.031. PMID 24262273.
- Patel A, Laffan MA, et al. (July 2014). "Randomised trials of human albumin for adults with sepsis: Systematic review and meta-analysis with trial sequential analysis of all-cause mortality". BMJ. 349: g4561. doi:10.1136/bmj.g4561. PMC 4106199. PMID 25099709. Archived from the original on 5 October 2014.
- Holst LB, Haase N, et al. (October 2014). "Lower versus higher hemoglobin threshold for transfusion in septic shock". The New England Journal of Medicine. 371 (15): 1381–91. doi:10.1056/NEJMoa1406617. PMID 25270275. S2CID 16280618.
- Alejandria MM, Lansang MA, et al. (September 2013). "Intravenous immunoglobulin for treating sepsis, severe sepsis and septic shock". The Cochrane Database of Systematic Reviews. 9 (9): CD001090. doi:10.1002/14651858.CD001090.pub2. PMC 6516813. PMID 24043371.
- Bai, X; Yu, W; Ji, W; Lin, Z; Tan, S; Duan, K; Dong, Y; Xu, L; Li, N (3 October 2014). "Early versus delayed administration of norepinephrine in patients with septic shock". Critical Care. 18 (5): 532. doi:10.1186/s13054-014-0532-y. PMC 4194405. PMID 25277635.
- Avni T, Lador A, et al. (2015). "Vasopressors for the treatment of septic shock: Systematic review and meta-analysis". PLOS One. 10 (8): e0129305. Bibcode:2015PLoSO..1029305A. doi:10.1371/journal.pone.0129305. PMC 4523170. PMID 26237037.
- Hamzaoui O, Scheeren TW, et al. (August 2017). "Norepinephrine in septic shock: When and how much?". Current Opinion in Critical Care. 23 (4): 342–7. doi:10.1097/mcc.0000000000000418. PMID 28509668.
- Dubin A, Lattanzio B, et al. (2017). "The spectrum of cardiovascular effects of dobutamine - from healthy subjects to septic shock patients". Revista Brasileira de Terapia Intensiva. 29 (4): 490–8. doi:10.5935/0103-507x.20170068. PMC 5764562. PMID 29340539.
- Patel GP, Balk RA (January 2012). "Systemic steroids in severe sepsis and septic shock". American Journal of Respiratory and Critical Care Medicine. 185 (2): 133–9. doi:10.1164/rccm.201011-1897CI. PMID 21680949.
- Volbeda M, Wetterslev J, et al. (July 2015). "Glucocorticosteroids for sepsis: Systematic review with meta-analysis and trial sequential analysis". Intensive Care Medicine. 41 (7): 1220–34. doi:10.1007/s00134-015-3899-6. PMC 4483251. PMID 26100123.
- Ni YN, Liu YM, et al. (September 2019). "Can corticosteroids reduce the mortality of patients with severe sepsis? A systematic review and meta-analysis". The American Journal of Emergency Medicine. 37 (9): 1657–64. doi:10.1016/j.ajem.2018.11.040. PMID 30522935.
- Marik PE, Pastores SM, et al. (June 2008). "Recommendations for the diagnosis and management of corticosteroid insufficiency in critically ill adult patients: Consensus statements from an international task force by the American College of Critical Care Medicine". Critical Care Medicine. 36 (6): 1937–49. doi:10.1097/CCM.0b013e31817603ba. PMID 18496365. S2CID 7861625.
- NHLBI–NIH ARDS Network (2014). "Tools". ardsnet.org. National Heart, Lung, and Blood Institute (NHLBI), National Institutes of Health (NIH). Archived from the original on 3 December 2019.
- Cherfan AJ, Arabi YM, et al. (May 2012). "Advantages and disadvantages of etomidate use for intubation of patients with sepsis". Pharmacotherapy. 32 (5): 475–82. doi:10.1002/j.1875-9114.2012.01027.x. PMID 22488264.
- Chan CM, Mitchell AL, et al. (November 2012). "Etomidate is associated with mortality and adrenal insufficiency in sepsis: A meta-analysis*". Critical Care Medicine. 40 (11): 2945–53. doi:10.1097/CCM.0b013e31825fec26. PMID 22971586. S2CID 916535.
- Gu WJ, Wang F, et al. (February 2015). "Single-dose etomidate does not increase mortality in patients with sepsis: A systematic review and meta-analysis of randomized controlled trials and observational studies". Chest. 147 (2): 335–46. doi:10.1378/chest.14-1012. PMID 25255427. S2CID 22739840.
- Lagunes, Leonel; Encina, Belen; Ramirez-Estrada, Sergio (September 2016). "Current understanding in source control management in septic shock patients: a review". Annals of Translational Medicine. 4 (17): 330. doi:10.21037/atm.2016.09.02. ISSN 2305-5839. PMC 5050189. PMID 27713888.
- De Waele, J. J.; Malbrain, M. M. L. G.; De Laet, I. E. (2009), Vincent, Jean-Louis (ed.), "Source Control in the ICU", Yearbook of Intensive Care and Emergency Medicine, Springer Berlin Heidelberg, pp. 93–101, doi:10.1007/978-3-540-92276-6_9, ISBN 978-3-540-92275-9
- Dellinger RP, Levy MM, et al. (January 2008). "Surviving Sepsis Campaign: International Guidelines for Management of Severe Sepsis and Septic Shock: 2008". Intensive Care Medicine. 34 (1): 17–60. doi:10.1007/s00134-007-0934-2. PMC 2249616. PMID 18058085.
- Rivers E, Nguyen B, et al. (November 2001). "Early goal-directed therapy in the treatment of severe sepsis and septic shock". The New England Journal of Medicine. 345 (19): 1368–77. doi:10.1056/NEJMoa010307. PMID 11794169.
- Fuller BM, Dellinger RP (June 2012). "Lactate as a hemodynamic marker in the critically ill". Current Opinion in Critical Care. 18 (3): 267–72. doi:10.1097/MCC.0b013e3283532b8a. PMC 3608508. PMID 22517402.
- Dell'Anna AM, Taccone FS (October 2015). "Early-goal directed therapy for septic shock: Is it the end?". Minerva Anestesiologica. 81 (10): 1138–43. PMID 26091011.
- Rusconi AM, Bossi I, et al. (September 2015). "Early goal-directed therapy vs usual care in the treatment of severe sepsis and septic shock: A systematic review and meta-analysis". Internal and Emergency Medicine. 10 (6): 731–43. doi:10.1007/s11739-015-1248-y. PMID 25982917. S2CID 207311061.
- Shane AL, Stoll BJ (January 2014). "Neonatal sepsis: Progress towards improved outcomes". Journal of Infection. 68 (Suppl 1): S24–32. doi:10.1016/j.jinf.2013.09.011. PMID 24140138.
- Camacho-Gonzalez A, Spearman PW, et al. (April 2013). "Neonatal infectious diseases: Evaluation of neonatal sepsis". Pediatric Clinics of North America. 60 (2): 367–89. doi:10.1016/j.pcl.2012.12.003. PMC 4405627. PMID 23481106.
- Kutko MC, Calarco MP, et al. (July 2003). "Mortality rates in pediatric septic shock with and without multiple organ system failure". Pediatric Critical Care Medicine. 4 (3): 333–7. doi:10.1097/01.PCC.0000074266.10576.9B. PMID 12831416. S2CID 18089789.
- Drewry AM, Ablordeppey EA, et al. (May 2017). "Antipyretic therapy in critically ill septic patients: A systematic review and meta-analysis". Critical Care Medicine. 45 (5): 806–13. doi:10.1097/CCM.0000000000002285. PMC 5389594. PMID 28221185.
- Niven DJ, Laupland KB, et al. (December 2013). "Diagnosis and management of temperature abnormality in ICUs: A EUROBACT investigators' survey". Critical Care. 17 (6): R289. doi:10.1186/cc13153. PMC 4057370. PMID 24326145.
- Launey Y, Nesseler N, et al. (2011). "Clinical Review: Fever in septic ICU patients--friend or foe?". Critical Care. 15 (3): 222. doi:10.1186/cc10097. PMC 3218963. PMID 21672276.
- Szakmany T, Hauser B, et al. (September 2012). "N-acetylcysteine for sepsis and systemic inflammatory response in adults". The Cochrane Database of Systematic Reviews. 9 (9): CD006616. doi:10.1002/14651858.CD006616.pub2. PMC 6517277. PMID 22972094.
- Martí-Carvajal AJ, Solà I, et al. (December 2012). "Human recombinant protein C for severe sepsis and septic shock in adult and paediatric patients". The Cochrane Database of Systematic Reviews. 12: CD004388. doi:10.1002/14651858.CD004388.pub6. PMC 6464614. PMID 23235609.
- Fink MP, Warren HS (October 2014). "Strategies to improve drug development for sepsis". Nature Reviews Drug Discovery. 13 (10): 741–58. doi:10.1038/nrd4368. PMID 25190187. S2CID 20904332.
- Hirasawa H, Oda S, et al. (September 2009). "Blood glucose control in patients with severe sepsis and septic shock". World Journal of Gastroenterology. 15 (33): 4132–6. doi:10.3748/wjg.15.4132. PMC 2738808. PMID 19725146.
- Russel JA (October 2008). "The current management of septic shock". Minerva Medica. 99 (5): 431–58. PMID 18971911.
- Carpenter CR, Keim SM, et al. (October 2009). "Risk stratification of the potentially septic patient in the emergency department: The Mortality in the Emergency Department Sepsis (MEDS) score". The Journal of Emergency Medicine. 37 (3): 319–27. doi:10.1016/j.jemermed.2009.03.016. PMID 19427752.
- Jackson JC, Hopkins RO, et al. (November 2009). "Acute respiratory distress syndrome, sepsis, and cognitive decline: A review and case study". Southern Medical Journal. 102 (11): 1150–7. doi:10.1097/SMJ.0b013e3181b6a592. PMC 3776422. PMID 19864995.
- Lyle NH, Pena OM, et al. (September 2014). "Barriers to the effective treatment of sepsis: Antimicrobial agents, sepsis definitions, and host-directed therapies". Annals of the New York Academy of Sciences. 1323 (2014): 101–14. Bibcode:2014NYASA1323..101L. doi:10.1111/nyas.12444. PMID 24797961.
- Munford RS (2011). "Ch. 271: Severe Sepsis and Septic Shock". In Longo DL, et al. (eds.). Harrison's Principles of Internal Medicine (18th ed.). New York: McGraw-Hill. pp. 2223–31. ISBN 9780071748896.
- Sutton JP, Friedman B (September 2013). "Trends in Septicemia Hospitalizations and Readmissions in Selected HCUP States, 2005 and 2010". Healthcare Cost and Utilization Project. Statistical Brief #161. United States National Library of Medicine. PMID 24228290. Archived from the original on 6 September 2017.
- Martin GS, Mannino DM, et al. (April 2003). "The epidemiology of sepsis in the United States from 1979 through 2000". The New England Journal of Medicine. 348 (16): 1546–54. doi:10.1056/NEJMoa022139. PMID 12700374.
- Hines AL, Barrett ML, et al. (April 2014). "Conditions with the Largest Number of Adult Hospital Readmissions by Payer, 2011". Healthcare Cost and Utilization Project. Statistical Brief #172. Agency for Healthcare Research and Quality: United States National Library of Medicine. PMID 24901179. Archived from the original on 4 March 2016.
- Koh GC, Peacock SJ, et al. (April 2012). "The impact of diabetes on the pathogenesis of sepsis". European Journal of Clinical Microbiology & Infectious Diseases. 31 (4): 379–88. doi:10.1007/s10096-011-1337-4. PMC 3303037. PMID 21805196.
- Rubin LG, Schaffner W (July 2014). "Clinical Practice: Care of the asplenic patient". The New England Journal of Medicine. 371 (4): 349–56. doi:10.1056/NEJMcp1314291. PMID 25054718.
- Geroulanos S, Douka ET (December 2006). "Historical perspective of the word "sepsis"". Intensive Care Medicine. 32 (12): 2077. doi:10.1007/s00134-006-0392-2. PMID 17131165. S2CID 37084190.
- Vincent J (2008). "Ch. 1: Definition of Sepsis and Non-infectious SIRS". In Cavaillon J, et al. (eds.). Sepsis and Non-infectious Systemic Inflammation: From Biology to Critical Care. John Wiley & Sons. p. 3. ISBN 9783527319350.
- Marshall JC (March 2008). "Sepsis: Rethinking the approach to clinical research". Journal of Leukocyte Biology. 83 (3): 471–82. CiteSeerX 10.1.1.492.7774. doi:10.1189/jlb.0607380. PMID 18171697.
- Stewart, Cynthia. "Understand How ICD-10 Expands Sepsis Coding - AAPC Knowledge Center". AAPC. Retrieved 6 February 2020.
- "Bacteremia". The Merck Manual—Home Health Handbook. Merck & Co. Archived from the original on 28 July 2017. Retrieved 25 November 2017.
- Shear MJ (1944). "Chemical treatment of tumors, IX: Reactions of mice with primary subcutaneous tumors to injection of a hemorrhage-producing bacterial polysaccharide". Journal of the National Cancer Institute. 4 (5): 461–76. doi:10.1093/jnci/4.5.461.
- Lüderitz O, Galanos C, et al. (1973). "Lipid A: Chemical structure and biological activity". The Journal of Infectious Diseases. 128: S17–S29. doi:10.1093/infdis/128.Supplement_1.S17. JSTOR 30106029. PMID 4352586.
- Heppner G, Weiss DW (September 1965). "High susceptibility of Strain A mice to endotoxin and endotoxin-red blood cell mixtures". Journal of Bacteriology. 90 (3): 696–703. doi:10.1128/JB.90.3.696-703.1965. PMC 315712. PMID 16562068.
- O'Brien AD, Rosenstreich DL, et al. (January 1980). "Genetic control of susceptibility to Salmonella typhimurium in mice: Role of the LPS gene". Journal of Immunology. 124 (1): 20–4. PMID 6985638.
- Poltorak A, Smirnova I, et al. (September 1998). "Genetic and physical mapping of the Lps locus: Identification of the toll-4 receptor as a candidate gene in the critical region". Blood Cells, Molecules and Diseases. 24 (3): 340–55. doi:10.1006/bcmd.1998.0201. PMID 10087992.
- Poltorak A, He X, et al. (December 1998). "Defective LPS signaling in C3H/HeJ and C57BL/10ScCr mice: Mutations in Tlr4 gene". Science. 282 (5396): 2085–8. Bibcode:1998Sci...282.2085P. doi:10.1126/science.282.5396.2085. PMID 9851930.
- Korneev, K. V. (18 October 2019). "Mouse Models of Sepsis and Septic Shock". Molecular Biology. 53 (5): 704–717. doi:10.1134/S0026893319050108.
- Lewis AJ, Seymour CW, et al. (August 2016). "Current murine models of sepsis". Surgical Infections. 17 (4): 385–93. doi:10.1089/sur.2016.021. PMC 4960474. PMID 27305321.
- Mills M, Estes MK (September 2016). "Physiologically relevant human tissue models for infectious diseases". Drug Discovery Today. 21 (9): 1540–52. doi:10.1016/j.drudis.2016.06.020. PMC 5365153. PMID 27352632.
- Engber D (13 February 2013). "Septic Shock". Slate. Archived from the original on 9 April 2017.
- Seok J, Warren HS, et al. (February 2013). "Genomic responses in mouse models poorly mimic human inflammatory diseases". Proceedings of the National Academy of Sciences of the United States of America. 110 (9): 3507–12. Bibcode:2013PNAS..110.3507S. doi:10.1073/pnas.1222878110. PMC 3587220. PMID 23401516.
- Hazeldine J, Hampson P, et al. (2016). "The diagnostic and prognostic value of systems biology research in major traumatic and thermal injury: A review". Burns & Trauma. 4: 33. doi:10.1186/s41038-016-0059-3. PMC 5030723. PMID 27672669.
- Torio CM, Moore BJ (1 January 2006). "National Inpatient Hospital Costs: The Most Expensive Conditions by Payer, 2013". Healthcare Cost and Utilization Project. Statistical Brief #204. Agency for Healthcare Research and Quality, National Library of Medicine. PMID 27359025. Archived from the original on 6 September 2017.
- Pfuntner A, Wier LM, et al. (December 2013). "Costs for Hospital Stays in the United States, 2011". Healthcare Cost and Utilization Project. Statistical Brief #168. National Library of Medicine. PMID 24455786.
- "History". Surviving Sepsis Campaign. Society of Critical Care Medicine. Archived from the original on 4 March 2014. Retrieved 24 February 2014.
- Sepsis Alliance. "About Us". sepsis.org. Archived from the original on 8 September 2015. Retrieved 8 October 2015.
- Rozsa L, Apari P, et al. (2017). "The evolutionary logic of sepsis". Infection, Genetics and Evolution. 55: 135–41. doi:10.1016/j.meegid.2017.09.006. PMID 28899789.
External links
![]() |
Wikimedia Commons has media related to Sepsis. |

- Sepsis at Curlie
- SIRS, Sepsis, and Septic Shock Criteria
- "Sepsis". MedlinePlus. U.S. National Library of Medicine.
Classification | |
---|---|
External resources |
|