Variable Specific Impulse Magnetoplasma Rocket
The Variable Specific Impulse Magnetoplasma Rocket (VASIMR) is an electrothermal thruster under development for possible use in spacecraft propulsion. It uses radio waves to ionize and heat an inert propellant, forming a plasma, then a magnetic field to confine and accelerate the expanding plasma, generating thrust. It is a plasma propulsion engine, one of several types of spacecraft electric propulsion systems.[1]
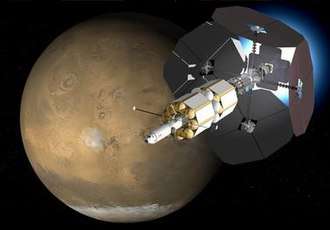
The VASIMR method for heating plasma was originally developed during nuclear fusion research. VASIMR is intended to bridge the gap between high thrust, low specific impulse chemical rockets and low thrust, high specific impulse electric propulsion, but has not yet demonstrated high thrust. The VASIMR concept originated in 1977 with former NASA astronaut Franklin Chang Díaz, who has been developing the technology ever since.[2]
Design and operation
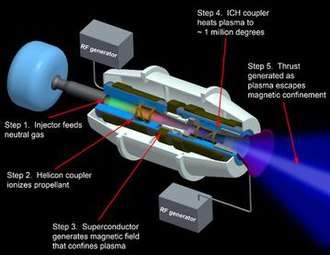
VASIMR is a type of electrothermal plasma thruster/electrothermal magnetoplasma thruster. In these engines, a neutral, inert propellant is ionized and heated using radio waves. The resulting plasma is then accelerated with magnetic fields to generate thrust. Other related electrically powered spacecraft propulsion concepts are the electrodeless plasma thruster, the microwave arcjet rocket, and the pulsed inductive thruster. Every part of a VASIMR engine is magnetically shielded and does not directly contact plasma, increasing durability. Additionally, the lack of electrodes eliminates the electrode erosion that shortens the life of conventional ion thruster designs.
The propellant, a neutral gas such as argon or xenon, is injected into a hollow cylinder surfaced with electromagnets. On entering the engine, the gas is first heated to a “cold plasma” by a helicon RF antenna/coupler that bombards the gas with electromagnetic energy, stripping electrons off the propellant atoms and producing a plasma of ions and free electrons. By varying the amount of RF heating energy and plasma, VASIMR is claimed to be capable of generating either low-thrust, high–specific impulse exhaust or relatively high-thrust, low–specific impulse exhaust.[3] The second phase of the engine is a strong solenoid-configuration electromagnet that channels the ionized plasma, acting as a convergent-divergent nozzle like the physical nozzle in conventional rocket engines.
A second coupler, known as the Ion Cyclotron Heating (ICH) section, emits electromagnetic waves in resonance with the orbits of ions and electrons as they travel through the engine. Resonance is achieved through a reduction of the magnetic field in this portion of the engine that slows the orbital motion of the plasma particles. This section further heats the plasma to greater than 1,000,000 K (1,000,000 °C; 1,800,000 °F) —about 173 times the temperature of the Sun's surface.[4]
The path of ions and electrons through the engine approximates lines parallel to the engine walls; however, the particles actually orbit those lines while traveling linearly through the engine. The final, diverging, section of the engine contains an expanding magnetic field that ejects the ions and electrons from the engine at velocities as great as 50,000 m/s (180,000 km/h).[3][5]
Advantages
In contrast to the typical cyclotron resonance heating processes, VASIMR ions are immediately ejected from the magnetic nozzle before they achieve thermalized distribution. Based on novel theoretical work in 2004 by Alexey V. Arefiev and Boris N. Breizman of University of Texas at Austin, virtually all of the energy in the ion cyclotron wave is uniformly transferred to ionized plasma in a single-pass cyclotron absorption process. This allows for ions to leave the magnetic nozzle with a very narrow energy distribution, and for significantly simplified and compact magnet arrangement in the engine.[3]
VASIMR does not use electrodes; instead, it magnetically shields plasma from most hardware parts, thus eliminating electrode erosion, a major source of wear in ion engines.[6] Compared to traditional rocket engines with very complex plumbing, high performance valves, actuators and turbopumps, VASIMR has almost no moving parts (apart from minor ones, like gas valves), maximizing long term durability.
Disadvantages
According to Ad Astra as of 2015, the VX-200 engine requires 200 kW electrical power to produce 5 N of thrust, or 40 kW/N.[5] In contrast, the conventional NEXT ion thruster produces 0.327 N with only 7.7 kW, or 24 kW/N.[5] Electrically speaking, NEXT is almost twice as efficient, and successfully completed a 48,000 hours (5.5 years) test in December 2009.[7][8]
New problems also emerge with VASIMR, such as interaction with strong magnetic fields and thermal management. The inefficiency with which VASIMR operates generates substantial waste heat that needs to be channeled away without creating thermal overload and thermal stress. The superconducting electromagnets necessary to contain hot plasma generate tesla-range magnetic fields[9] that can cause problems with other onboard devices and produce unwanted torque by interaction with the magnetosphere. To counter this latter effect, two thruster units can be packaged with magnetic fields oriented in opposite directions, making a net zero-torque magnetic quadrupole.[10]
The required power generation technology for fast interplanetary travel does not currently exist and is not feasible with current state-of-the-art technology.[11]
Research and development
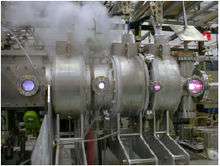
The first VASIMR experiment was conducted at Massachusetts Institute of Technology in 1983. Important refinements were introduced in the 1990s, including the use of the helicon plasma source, which replaced the plasma gun originally envisioned and its electrodes, adding to durability and long life.
In 1995, the Advanced Space Propulsion Laboratory (ASPL) was founded at NASA Lyndon B. Johnson Space Center, in the Sonny Carter Training Facility, with the equipment from MIT. The first plasma experiment in Houston was conducted with a microwave plasma source.
As of 2010, Ad Astra Rocket Company (AARC) was responsible for VASIMR development, signing the first Space Act Agreement in 23 June 2005 to privatize VASIMR technology. Franklin Chang Díaz is Ad Astra's chairman and CEO, and the company had a testing facility in Liberia, Costa Rica on the campus of Earth University.[12]
VX-10 to VX-50
In 1998, the first helicon plasma experiment was performed at the ASPL. VASIMR experiment (VX) 10 in 1998 achieved a helicon RF plasma discharge of up to 10 kW and VX-25 in 2002 of up to 25 kW. By 2005 progress at ASPL included full and efficient plasma production and acceleration of the plasma ions with the 50 kW, 0.5 newtons (0.1 lbf) thrust VX-50.[13] Published data on the 50 kW VX-50 showed the electrical efficiency to be 59% based on a 90% coupling efficiency and a 65% ion speed boosting efficiency.[13]
VX-100
The 100 kilowatt VASIMR experiment was successfully running by 2007 and demonstrated efficient plasma production with an ionization cost below 100 eV.[14] VX-100 plasma output tripled the prior record of the VX-50.[14]
The VX-100 was expected to have an ion speed boosting efficiency of 80%, but could not achieve this efficiency due to losses from the conversion of DC electric current to radio frequency power and the auxiliary equipment for the superconducting magnet.[13][15] In contrast, 2009 state-of-the-art, proven ion engine designs such as NASA's High Power Electric Propulsion (HiPEP) operated at 80% total thruster/PPU energy efficiency.[16]
VX-200
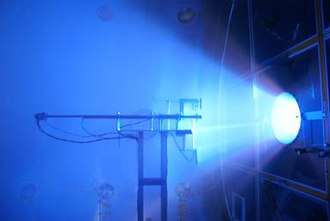
On 24 October 2008, the company announced in a press release that the helicon plasma generation component of the 200 kW VX-200 engine had reached operational status. The key enabling technology, solid-state DC-RF power-processing, reached 98% efficiency. The helicon discharge used 30 kW of radio waves to turn argon gas into plasma. The remaining 170 kW of power was allocated for acceleration of plasma in the second part of the engine, via ion cyclotron resonance heating.[17]
Based on data from VX-100 testing,[9] it was expected that the VX-200 engine would have a system efficiency of 60–65% and a potential thrust level of 5 N. Optimal specific impulse appeared to be around 5,000 s using low cost argon propellant. One of the remaining untested issues was whether the hot plasma actually detached from the rocket. Another issue was waste heat management. About 60% of input energy became useful kinetic energy. Much of the remaining 40% is secondary ionizations from plasma crossing magnetic field lines and exhaust divergence. A significant portion of that 40% was waste heat (see energy conversion efficiency). Managing and rejecting that waste heat is critical.[18]
Between April and September 2009, 200 kW tests were performed on the VX-200 prototype with integrated 2-tesla superconducting magnets.[19] During November 2010, long duration, full power firing tests were performed, reaching steady state operation for 25 seconds and validating basic design characteristics.[20]
Results presented in January 2011 confirmed that the design point for optimal efficiency on the VX-200 is 50 km/s exhaust velocity, or an Isp of 5000 s. Based on these data, thruster efficiency of 72% was achieved,[21] yielding overall system efficiency (DC electricity to thruster power) of 60% with argon propellant.[22] VX-200 generates a thrust of around 5.4 N at 200 kW total RF power, and 3.2 N at 100 kW RF power.[21]:5
The 200 kW VX-200 had executed more than 10,000 engine firings with argon propellant at full power by 2013, demonstrating greater than 70% thruster efficiency relative to RF power input.[23]
VX-200SS
In March 2015, Ad Astra announced a $10 million award from NASA to advance the technology readiness of the next version of the VASIMR engine, the VX-200SS to meet the needs of deep space missions.[24] The SS in the name stands for "steady state", as a goal of the long duration test is to demonstrate continuous operation at thermal steady state.[25]
In August 2016, Ad Astra announced completion of the milestones for the first year of its 3-year contract with NASA. This allowed for first high-power plasma firings of the engines, with a stated goal to reach 100 hr and 100 kW by mid-2018.[26] In August 2017, the company reported completing its Year 2 milestones for the VASIMR electric plasma rocket engine. NASA gave approval for Ad Astra to proceed with Year 3 after reviewing completion of a 10-hour cumulative test of the VX-200SS engine at 100 kW. It appears as though the planned 200 kW design is being run at 100 kW for reasons that are not mentioned in the press release.[27]
In August, 2019[28] Ad Astra announced the successful completion of tests of a new generation radio-frequency (RF) Power Processing Unit (PPU) for the VASIMR engine, built by Aethera Technologies Ltd. of Canada. Ad Astra declared a power of 120 kW and >97% electrical-to-RF power efficiency, and that, at 52 kg, the new RF PPU is about 10x lighter than the PPUs of competing electric thrusters (power-to-weight ratio: 2.31 kW/kg)
Potential applications
VASIMR has a low thrust-to-weight ratio and requires an ambient vacuum. The engine would function as an upper stage for cargo, drag compensation for space stations, lunar cargo delivery, satellite repositioning, satellite refueling, maintenance and repair, in space resource recovery, and deep space robotic missions.[29]
Other proposed applications for VASIMR such as the rapid transportation of people to Mars would require a very high power, low mass energy source, such as a nuclear reactor (see nuclear electric rocket). In 2010 NASA Administrator Charles Bolden said that VASIMR technology could be the breakthrough technology that would reduce the travel time on a Mars mission from 2.5 years to 5 months.[29]
In August 2008, Tim Glover, Ad Astra director of development, publicly stated that the first expected application of VASIMR engine is "hauling things [non-human cargo] from low-Earth orbit to low-lunar orbit" supporting NASA's return to Moon efforts.[30]
ISS reboost - cancelled
On 10 December 2007, AARC and NASA signed an Umbrella Space Act Agreement relating to the space agency's potential interest in the engine.[31] On 8 December 2008, NASA and AARC entered into a Space Act Agreement that was intended to result in a space flight test of the engine on the ISS.[32] A formal preliminary design review took place on 26 June 2013.[23]
In 2 March 2011, Ad Astra and NASA Johnson Space Center signed a Support Agreement to collaborate on research, analysis and development on space-based cryogenic magnet operations and electric propulsion systems currently under development by Ad Astra.[33] By February 2011, about 100 NASA people were working with Ad Astra to integrate the VF-200 onto the International Space Station.[34] On December 16, 2013, AARC and NASA signed another five-year Umbrella Space Act Agreement.[35]
However, in 2015 NASA ended plans for flying the VF-200 to the ISS. A NASA spokesperson stated that the ISS "was not an ideal demonstration platform for the desired performance level of the engines". Ad Astra stated that tests of a VASIMR thruster on the ISS would remain an option after a future in-space demonstration.[36] Work with NASA continued in 2015 under NASA's NextSTEP program with planning for a 100-hour vacuum chamber test of the VX-200SSTM thruster.[37]
Space tug/orbital transfer vehicle
A possible application of VASIMR-powered spacecraft is cargo transport. As with all ion engines, higher efficiency is balanced against longer transit times. An orbital transfer vehicle (OTV) powered by a single VF-200 engine could be capable of transporting about 7 metric tons of cargo from low Earth orbit (LEO) to low Lunar orbit (LLO) with about a six-month transit time.
NASA envisioned delivering about 34 metric tons of useful cargo to LLO in a single flight with a chemically propelled vehicle. To make that trip, about 60 metric tons of LOX-LH2 propellant would be expended. A comparable OTV would employ 5 VF-200 engines powered by a 1 MW solar array, expending about 8 metric tons of argon propellant. The total mass of such an electric OTV would be in the range of 49 t (outbound & return fuel: 9 t, hardware: 6 t, cargo 34 t).
OTV transit times can be reduced by carrying lighter loads and/or expending more argon propellant with VASIMR throttled up to higher thrust at less efficient (lower Isp) operating conditions. For instance, an empty OTV on the return trip to Earth covers the distance in about 23 days at optimal specific impulse of 5,000 s (50 kN·s/kg) or in about 14 days at Isp of 3,000 s (30 kN·s/kg). The total mass of the NASA specifications' OTV (including structure, solar array, fuel tank, avionics, propellant and cargo) was assumed to be 100 metric tons (98.4 long tons; 110 short tons)[38] allowing almost double the cargo capacity compared to chemically propelled vehicles but requiring even bigger solar arrays (or other source of power) capable of providing 2 MW.
Mars in 39 days
In order to conduct a proposed crewed trip to Mars in 39 days,[39] the VASIMR would require an electrical power level projected to be developed only by nuclear propulsion in an application of nuclear power in space.[40] This kind of nuclear fission reactor might use a traditional Rankine/Brayton/Stirling engine to convert heat to electricity. Examples of such reactors include the SAFE-400 and the DUFF Kilopower.
However, any power generation technology will produce waste heat. The necessary 200 megawatt reactor "with a power-to-mass density of 1,000 watts per kilogram" (Díaz quote) would require extremely efficient radiators to avoid the need for "football-field sized radiators" (Zubrin quote).[41] For comparison, a Seawolf-class nuclear-powered fast attack submarine uses a propulsion plant with a shaft output of 34 megawatts (MW), and the Gerald R. Ford-class aircraft carrier uses two A1B reactor plants, each with a 700 MW thermal output and an approximately 385 MW useful output.[42] Naval reactors have the advantage of an essentially infinite heat sink - the ocean - which can be easily accessed, and can be constructed of heavy materials such as steel and lead.
See also
Electric propulsion
- Helicon Double Layer Thruster
- Magnetoplasmadynamic thruster
- Nano-particle field extraction thruster
- Pulsed plasma thruster
Space reactors
- Project Prometheus
- Safe Affordable Fission Engine
- Systems for Nuclear Auxiliary Power
- TOPAZ nuclear reactor
References
- Ad Astra Rocket Company. "VASIMR". Ad Astra Rocket Company. Archived from the original on July 7, 2019. Retrieved July 9, 2019.
- Ad Astra Rocket Company. "History". Ad Astra Rocket Company. Retrieved July 9, 2019.
- Tim W. Glover; et al. (February 13–17, 2005). "Principal VASIMR Results and Present Objectives" (PDF). Space Technology and Applications International Forum - Staif 2005. 746: 976–982. Bibcode:2005AIPC..746..976G. doi:10.1063/1.1867222. Retrieved February 27, 2010.
- Beth Dickey (March 2004). "Star Power". Air & Space, Smithsonian. Retrieved February 7, 2014.
- Ad Astra Rocket Company (2009). "Technology". Ad Astra Rocket Company. Retrieved December 10, 2012.
- Jared P. Squire; Franklin R. Chang Díaz; Verlin T. Jacobson; Tim W. Glover; F. Wally Baity; Richard H. Goulding; Roger Bengtson; et al. "EXPERIMENTAL RESEARCH PROGRESS TOWARD THE VASIMR ENGINE" (PDF). 28th International Electric Propulsion Conference, Toulouse, France, 17–21 March 2003. 28th International Electric Propulsion Conference. Retrieved February 7, 2014.
- Dwayne Brown; Katherine K. Martin; Glenn Mahone. "NASA Thruster Achieves World-Record 5+ Years of Operation". Retrieved June 24, 2013.
- Nancy Smith Kilkenny, SGT, Inc. "NEXT Provides Lasting Propulsion and High Speeds for Deep Space Missions". Retrieved September 29, 2013.CS1 maint: multiple names: authors list (link)
- Jared P. Squire; et al. (September 5–6, 2008). "VASIMR Performance Measurements at Powers Exceeding 50 kW and Lunar Robotic Mission Applications" (PDF). International Interdisciplinary Symposium on Gaseous and Liquid Plasmas. Retrieved February 27, 2010.
- "International Space Station Mission". Ad Astra Rocket Company. 2011. Archived from the original on March 15, 2011. Retrieved February 8, 2011.
The VX-200 will provide the critical data set to build the VF-200-1, the first flight unit, to be tested in space aboard the International Space Station (ISS). The electrical energy will come from ISS at low power level, be stored in batteries and used to fire the engine at 200 kW.
- "Facts About the VASIMR® Engine and its Development" (PDF). Ad Astra Rocket Company. July 15, 2011.
- "Executive summary" (PDF). Ad Astra Rocket Company. January 24, 2010. Archived from the original (PDF) on March 31, 2010. Retrieved February 27, 2010.
- Squire, Jared P; et al. (September 17–20, 2007). "High Power VASIMR Experiments using Deuterium, Neon and Argon" (PDF). International Electric Propulsion Conference 2007. Retrieved February 27, 2010.
- "Ad Astra's VX-100 test bed achieves record plasma performance" (PDF). PRESS RELEASE 061207, June 12, 2007. Ad Astra. Retrieved February 7, 2014.
- Bering, Edgar A; et al. (January 9–12, 2006). "Recent Improvements In Ionization Costs And Ion Cyclotron Heating Efficiency In The VASIMR Engine" (PDF). AIAA Aerospace Sciences Meeting and Exhibit. Retrieved February 27, 2010.
- Elliott, Frederick W; et al. (July 11–14, 2004). "An Overview of the High Power Electric Propulsion (HiPEP) Project" (PDF). AIAA/ASME/SAE/ASEE Joint Propulsion Conference & Exhibit. Retrieved February 27, 2010.
- "VASIMR VX-200 first stage achieves full power rating" (PDF) (Press release). Ad Astra Rocket Company. October 24, 2008. Retrieved February 27, 2010.
- de Faoite, Daithí; Browne, David J; Chang-Díaz, Franklin R; Stanton, Kenneth T (November 17, 2011). "A review of the processing, composition, and temperature-dependent mechanical and thermal properties of dielectric technical ceramics". Journal of Materials Science. 47 (10): 4211–4235. Bibcode:2012JMatS..47.4211F. doi:10.1007/s10853-011-6140-1. hdl:10197/8477.
- "VASIMR VX-200 reaches 200 kW power milestone" (PDF) (Press release). Ad Astra Rocket Company. September 30, 2011. Retrieved February 24, 2012.
- Benwl (December 15, 2010). "Video of VASIMR VX-200 firing for 25 seconds at full power rating". Ad Astra Rocket Company. Retrieved January 4, 2011.
- Longmier, Benjamin. "VASIMR VX-200 Performance Measurements and Helicon Throttle Tables Using Argon and Krypton" (PDF). IEPC-2011.
- Glover, Tim (January 19, 2011). "VASIMR VX-200 Performance and Near-term SEP Capability for Unmanned Mars Flight" (PDF). UTexas.edu. Archived from the original (PDF) on March 11, 2011. Retrieved January 31, 2011.
- Ilin, Andrew V.; Gilman, Daniel A.; Carter, Mark D.; Chang Díaz, Franklin R.; Squire, Jared P.; Farrias, Joseph E. (2013). VASIMR Solar Powered Missions for NEA Retrieval and NEA Deflection (PDF). 33rd International Electric Propulsion Conference. October 6–10, 2013. Washington, D.C. IEPC-2013-336.
- NASA Announces New Partnerships with U.S. Industry for Key Deep-Space Capabilities. March 30, 2015.
- AD ASTRA Rocket Company Wins Major NASA Advanced Propulsion Contract. Press release. March 31, 2015
- [adastrarocket.com/pressReleases/AdAstra-Release-080316-final.pdf AD ASTRA ROCKET COMPANY SUCCESSFULLY COMPLETES ALL NASA NEXTSTEP CONTRACT MILESTONES FOR YEAR ONE, RECEIVES NASA APPROVAL TO PROCEED WITH YEAR TWO.]. PRESS RELEASE 080316, August 3, 2016
- "PRESS RELEASE Ad Astra Rocket Company successfully completes all NASA NextStep Contract Milestones for year two, receives NASA approval to proceed with year three, 08/09/17" (PDF). Ad Astra Rocket Company. Retrieved August 9, 2017.
- "PRESS RELEASE 082019" (PDF). August 20, 2019.
- Morring, Frank (2010). "Commercial Route". Aviation Week & Space Technology. 172 (6): 20–23.
- Irene Klotz (August 7, 2008). "Plasma Rocket May Be Tested at Space Station". Discovery News. Retrieved February 27, 2010.
- "Ad Astra Rocket Company and NASA sign second collaborative agreement relating to the VASIMR™ engine" (PDF). PRESS RELEASE 101207, December 10, 2007 (Press release). Ad Astra Rocket Company. December 10, 2007.
- "NASA and Ad Astra Rocket Company sign Agreement for flight test of the VASIMR rocket engine aboard the International Space Station" (PDF) (Press release). Ad Astra. December 12, 2008.
- "Ad Astra and NASA sign support agreement on VASIMR technology" (PDF) (Press release). Ad Astra. March 8, 2011.
- Lindsay, Clark S. (February 7, 2011). "Briefs: Japan & commercial crew; VASIMR on ISS update; Delta IV Heavy liftoff flames". HobbySpace.com. Archived from the original on November 2, 2011. Retrieved February 8, 2011.
About 100 NASA people are now working with AAR on the project. AAR is negotiating with NASA for a launcher and the leading contender currently is Orbital Science's Taurus II. The VASIMR system will provide re-boost for the station plus it can also offer access to its 50 kWh (180 MJ) batteries when not in operation. The thruster can fire for up to 15 minutes at 200 kW. The lab prototype has exceeded thruster output by a factor of two over the requirements set for the ISS version.
- "Ad Astra Rocket Company and NASA Sign New Agreements on VASIMR Development" (PDF) (Press release). Ad Astra. December 19, 2013. Retrieved January 1, 2014.
- NASA nixes Ad Astra rocket test on the space station. SEN News, Irene Klotz. 17 March 2015.
- Ad Astra Rocket Company and NASA move to execution phase of NextSTEP VASIMR partnership spaceref.com
- Tim W. Glover; et al. (September 17–20, 2007). "Projected Lunar Cargo Capabilities of High-Power VASIMR Propulsion" (PDF). International Electric Propulsion Conference. Retrieved February 27, 2010.
- Video: "Mars in 39 Days?: the VASIMR Plasma Engine. Franklin Chang-Diaz, Ph.D."
- David Buden, Space Nuclear Fission Electric Power System: Book 3: Space Nuclear Propulsion and Power
- Video: VASIMR Debate/The VASIMR Hoax – Dr. Robert Zubrin – 14th International Mars Society Convention, Time Index 14:30
- "US Navy Propulsion Systems". Federation of American Scientists. Archived from the original on October 9, 2006. Retrieved February 2, 2019.
power per reactor ... 140,000 shp
Further reading
- "Agreement to Commercialize Advanced NASA Rocket Concept; Former Astronaut Franklin Chang-Diaz to Lead Effort" (Press release). Johnson Space Center. January 23, 2006. J06-009. Retrieved January 18, 2008.
- Naone, Erica (September 25, 2007). "Rocket scientist Franklin Chang Diaz talks about finding the power and propulsion required to colonize space". Technology Review. Retrieved February 27, 2010.
- Upson, Sandra (June 2009). "Rockets For The Red Planet". IEEE Spectrum. Archived from the original on July 22, 2011. Retrieved February 27, 2010.
External links
![]() |
Wikimedia Commons has media related to VASIMR. |
- "Plasma Rocket" (Video). Brink. Science. December 18, 2008.
- NASA documents