Leonite
Leonite is a hydrated double sulfate of magnesium and potassium. It has the formula K2SO4·MgSO4·4H2O. The mineral was named after Leo Strippelmann, who was director of the salt works at Westeregeln in Germany.[3] The mineral is part of the blodite group of hydrated double sulfate minerals.[2]
Leonite | |
---|---|
![]() Leonite | |
General | |
Category | Sulfate mineral |
Formula (repeating unit) | K2Mg(SO4)2·4H2O |
Strunz classification | 7.CC.55 |
Dana classification | 29.03.03.01 |
Crystal system | Monoclinic |
Crystal class | Prismatic (2/m) (same H-M symbol) |
Space group | C2/m |
Unit cell | a = 11.78, b = 9.53 c = 9.88 [Å]; β = 95.4°; Z = 4 |
Identification | |
Formula mass | 366.69 g/mol |
Color | White to colorless, yellow |
Crystal habit | Tabular crystals |
Twinning | {100} |
Cleavage | none |
Fracture | conchoidal |
Mohs scale hardness | 2.5 - 3 |
Luster | Vitreous or Waxy |
Streak | White |
Diaphaneity | Transparent to translucent |
Specific gravity | 2.201 |
Optical properties | Biaxial (+) |
Refractive index | nα = 1.479 nβ = 1.482 nγ = 1.487 |
Birefringence | δ = 0.008 |
2V angle | Measured: 90° Calc: 76° |
Dispersion | none |
Fusibility | easy |
Other characteristics | Leonit, 钾镁矾, Leonita, Леонит, Kalium-Astrakanit, Kalium-Blödit |
References | [1][2] |
Properties
Leonite has a bitter taste.[4]
When leonite is analyzed for elements, it is usually contaminated with sodium and chloride ions, as it commonly occurs with sodium chloride.[4]
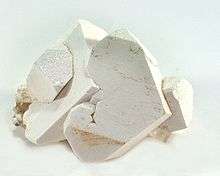
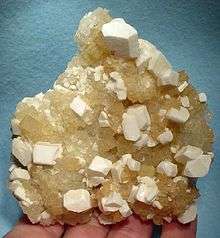
Crystal structure
In the mineral family of leonite, the lattice contains sulfate tetrahedrons, a divalent element in an octahedral position surrounded by oxygen, and water and univalent metal (potassium) linking these other components together. One sulfate group is disordered at room temperature. The disordered sulfate becomes fixed in position as temperature is lowered. The crystal form also changes at lower temperatures, so two other crystalline forms of leonite exist at lower temperatures.[5]
The divalent metal cation (magnesium) is embedded in oxygen octahedra, four from water around the equator, and two from sulfate ions at the opposite poles. In the crystal there are two different octahedral environments. Each of these octahedra are joined together by potassium ions and hydrogen bonds.[6]
Phase changes
The sulfate occurs in layers parallel to the (001) surface. In the room temperature form, the sequence is ODODODODOD with O=ordered, and D=disordered. In the next form at lower temperatures, the disordered sulfate appears in two different orientations giving the sequence OAOBOAOBOAOBOAOB. At the lowest temperatures, the sequence simplifies to OAOAOAOAOAO.[7]
The first phase transition happens at -4 °C.[8] At 170 K (−103 °C), the crystals have space group I2/a, lattice parameters a = 11.780 Å, b = 9.486 Å, c = 19.730 Å, β = 95.23°, 8 formula per unit cell, and a cell volume of V = 2195.6 Å3.[5] The c dimension and unit cell volume are doubled due to the presence of four sulfate layers rather than two as in the other forms.[7] The next phase change happens at -153 °C.[8] At 100 K (−173 °C), the space group is P21/a, a = 11.778 Å, b = 9.469 Å, c = 9.851 Å, β = 95.26°, 4 formula per unit cell, and a cell volume of V = 1094.01 Å3.[5]
Temperature effects
As temperature increases, the cell volume gradually increases for the I2/a and C2/m phases; however, the a dimension decreases with increasing temperature. The change in a dimension is −11×10−6 K−1.[8] Birefringence drops as temperature rises. It varies from 0.0076 at −150 °C down to 0.0067 at 0 °C and 0.0061 at 100 °C.[8] At the lower phase transition, birefringence steps down as the temperature drops; for the upper phase transition, it is continuous but not constant.[8]
At the upper phase transition, −4 °C, latent heat is released, and the heat capacity changes. This transition has a fair bit of hysteresis. At the lower phase transition, heat capacity stays the same, but latent heat is released.[8]
Leonite starts to lose water at 130 °C, but only really breaks down at 200 °C:[4]
- K2Mg(SO4)2·4H2O(s) → K2Mg(SO4)2·2H2O(s) + 2H2O(g).
At even higher temperatures, langbeinite and arcanite (anhydrous potassium sulfate) and steam are all that remain:[4][9]
- 2K2Mg(SO4)2·4H2O(s) → K2Mg2(SO4)3(s) + K2SO4(s) + 8H2O(g).
Other physical properties
The logarithmic solubility product Ksp for leonite is −9.562 at 25 °C.[10] The equilibrium constant log K at 25 °C is −3.979.[11] The chemical potential of leonite is μj°/RT = −1403.97.[12]
Thermodynamic properties include ΔfGok = −3480.79 kJ mol−1; ΔfHok = −3942.55 kJ mol−1; and ΔCop,k = 191.32 J K−1 mol−1.[13]
The infrared spectrum of sulfate stretching modes shows peaks in absorption at 1005, 1080, 1102, 1134 and 1209 cm−1. Sulfate bending mode causes a peak at 720, and lesser peaks at 750 and 840 cm−1. An OH stretching mode absorbs at 3238 cm−1. When temperatures reduce, the peaks move and/or narrow, and additional peaks may appear at phase transitions.[6]
When leonite is stored for exhibition, it must not be in a place with too much humidity, otherwise it hydrates more.[14]
Formation
Starting in 1897, Jacobus Henricus van 't Hoff investigated how different salts were formed as sea water evaporated in different conditions. His purpose was to discover how salt deposits are formed. His research formed the basis for the studies of the conditions in which leonite is formed.[15]
Leonite can form when a water solution of potassium sulfate and magnesium sulfate is concentrated between the temperature range of 320–350 K (47–77 °C). Above this temperature range, langbeinite (K2Mg2(SO4)3) is formed. Below 320 K (47 °C), picromerite (K2Mg(SO4)2·6H2O) crystallises.[16] For solutions with more than 90% proportion MgSO4, hexahydrite (MgSO4·6H2O) crystallises preferentially, and below 60%, arcanite (K2SO4) forms.[16]
In mixtures of potassium chloride, potassium sulfate, magnesium chloride and magnesium sulfate at 35 °C in water, leonite can crystallise out in a certain composition range. The plot of the system forms boundaries of leonite with potassium chloride, potassium sulfate, and picromerite. As magnesium is enriched, a quadruple point with kainite exists.[17]
In salt (NaCl) saturated brine, leonite can be deposited from magnesium and potassium sulfate mixtures as low as 25 °C. The 25 °C isotherm of the system has leonite surrounded by sylvine, picromerite, astrakanite, epsomite, and kainite. Sodium chloride saturated brines are formed by seawater evaporation, though seawater does not contain enough potassium to deposite leonite this way.[18]
Leonite is precipitated in series solar ponds at the Great Salt Lake.[19]
When picromerite is heated to between 85 and 128 °C, it gives off steam to give leonite:[20][21]
- K2Mg(SO4)2·6H2O(s) → K2Mg(SO4)2·4H2O(s) + 2H2O(g).
Reactions
When leonite is dissolved in nitric acid and then crystallised, an acid potassium magnesium double sulfate is formed: KHMg(SO4)2·2H2O.[22]
Leonite heated with hydrated magnesium sulfate in an equimolar ratio at 350 °C produces langbeinite:[23]
- K2Mg(SO4)2·4H2O(s) + MgSO4·xH2O(s) → K2Mg2(SO4)3(s) + (4 + x)H2O(g).
Potassium chloride solution can convert leonite to solid potassium sulfate:[24]
- 2KCl(aq) + K2Mg(SO4)2·4H2O(s) → 2K2SO4(s) + MgCl2(aq).
More potassium sulfate can be precipitated by adding ethylene glycol.[25]
Fluorosilicic acid in water reacts with leonite to produce insoluble potassium fluorosilicate and a solution of magnesium sulfate and sulfuric acid:[26]
- H2SiF6(aq) + K2Mg(SO4)2·4H2O(s) → K2SiF6(s) + MgSO4(aq) + H2SO4(aq).
Between 15 and 30 °C, a 22% magnesium chloride solution will react with leonite or picromerite to yield solid potassium chloride and hydrated magnesium sulfate.[27]
Natural occurrence
Leonite can form during the dehydration of seawater or lakewater. Leonite can be a minor primary constituent of evaporite potash deposits, or a secondary mineral.[28] In order to form leonite from seawater, the brine must separate from the deposited solids so that reactions do not happen with earlier deposited salts, and the temperature must be around 32 °C. Below 25° or above 40°, the content of the brine will not be suitable to deposit leonite.[28] At this temperature, blodite deposits first, and then leonite, constituting only 3.2% of the bittern salts.[28]
Secondary reactions can produce or consume leonite in evaporite deposits. Leonite can convert to polyhalite, and kieserite can be changed to leonite,[28] Groundwater penetrating bittern salt deposits can convert some to leonite, particularly in the cap regions of salt domes.[28]
Leonite was first found in nature in the Stassfurt Potash deposit, Westeregeln, Egeln, Saxony-Anhalt, Germany.[1] The Stassfurt salt deposits are from the Permian period. They are under the Magdeburg-Halberstadt region in central Germany. The leonite occurs in the salt clay and carnallite beds, which are up to 50 meters thick.[29] Other locations in Germany are the Neuhof-Ellers Potash Works in Neuhof, Fulda, Hesse; the Riedel Potash Works in Riedel-Hänigsen, Celle, Lower Saxony; Aschersleben; Vienenburg; and Leopoldshall.[1] Outside Germany, it is found at Vesuvius, Italy; Stebnyk, Ukraine; and the Carlsbad potash district, Eddy County, New Mexico, US. It is found in crystalline speleothems in Tăuşoare Cave in Romania; here it occurs with konyaite (K2Mg(SO4)2·5H2O), syngenite (K2Ca(SO4)2·H2O), thenardite (Na2SO4), and mirabilite (Na2SO4·10H2O).[30] Leonite also occurs in Wooltana Cave, Flinders Ranges, South Australia.[31]
Soil in the Gusev Crater on Mars contains leonite as well as many other hydrated sulfates.[32] On Europa, leonite is predicted to be stable, with a vapour pressure 10−13 that of ice. It is stable at pressures up to 10−7, above which a more hydrated salt exists. It should form up to 2% of the salts near the surface.[33]
Weathering of potassium-rich medieval glass forms a weathering crust that can contain leonite.[34]
Use
Leonite can be used directly as a fertilizer, contributing potassium and magnesium. It can be refined to K2SO4 for fertilizer use.[35] The process to convert leonite to potassium sulfate involves mixing it with a potassium chloride (a cheaper chemical) solution. The desired product, potassium sulfate, is less soluble and is filtered off. Magnesium chloride is very soluble in water. The filtrate is concentrated by evaporation, where more leonite crystallises, which is then recycled to the start of the process, adding more langbeinite or picromerite.[24]
Leonite may have been used in an alchemical formula to make "potable gold" around 300 AD in China. This was likely to be a liquid colloid of gold.[36]
Related
Leonite is isotypic with the mineral mereiterite (K2Fe(SO4)2·4H2O), and with artificial Mn-leonite (K2Mn(SO4)2·4H2O). Others with the same crystal structure include:
- K2Cd(SO4)2·4H2O
- (NH4)2Mg(SO4)2·4H2O
- (NH4)2Mn(SO4)2·4H2O
- (NH4)2Fe(SO4)2·4H2O
- (NH4)2Co(SO4)2·4H2O and
- K2Mg(SeO4)2·4H2O.[37]
Myron Stein suggested using the name "leonite" for element 96, naming it after the constellation Leo. This name was not accepted and curium was the name assigned.[38]
References
- Mindat.org
- Leonite Webmineral data
- "Leonite" (PDF). Mineral Data Publishing. 2005.
- Bilonizhka, P. (2003). "Leonite in Pre-Carpathian Evaporites and its Transformation Under Increased Temperatures" (PDF). Acta Mineralogica-Petrographica. 1: 14. Retrieved 17 November 2015.
- Hertweck, Birgit; Giester, Gerald; Libowitzky, Eugen (October 2001). "The crystal structures of the low-temperature phases of leonite-type compounds, K2 Me(SO4)2 ·4H2O (Me = Mg, Mn, Fe)". American Mineralogist. 86 (10): 1282–1292. Bibcode:2001AmMin..86.1282H. doi:10.2138/am-2001-1016.
- Hertweck, Birgit; Libowitzky, Eugen (1 December 2002). "Vibrational spectroscopy of phase transitions in leonite-type minerals". European Journal of Mineralogy. 14 (6): 1009–1017. Bibcode:2002EJMin..14.1009H. doi:10.1127/0935-1221/2002/0014-1009.
- Libowitzky, Eugen (2006). "Crystal Structure Dynamics: Evidence by Diffraction and Spectroscopy". Croatica Chemica Acta. 29 (2): 299–309.
- Hertweck, B.; Armbruster, T.; Libowitzky, E. (1 July 2002). "Multiple phase transitions of leonite-type compounds: optical, calorimetric, and X-ray data". Mineralogy and Petrology. 75 (3–4): 245–259. Bibcode:2002MinPe..75..245H. doi:10.1007/s007100200027.
- Balić-Žunić, Tonči; Birkedal, Renie; Katerinopoulou, Anna; Comodi, Paola (20 September 2015). "Dehydration of blödite, Na2Mg(SO4)2(H2O)4, and leonite, K2Mg(SO4)2(H2O)4". European Journal of Mineralogy. 28 (1): 33–42. Bibcode:2016EJMin..28...33B. doi:10.1127/ejm/2015/0027-2487.
- Kwok, Kui S.; Ng, Ka M.; Taboada, Maria E.; Cisternas, Luis A. (March 2008). "Thermodynamics of salt lake system: Representation, experiments, and visualization" (PDF). AIChE Journal. 54 (3): 706–727. doi:10.1002/aic.11421. table 7 on page 716
- Plummer, L. N.; Parkhurst, D. L.; Fleming, G. W.; Dunkle, S. A. (1988). "A Computer Program Incorporating Pitzer's Equations for Calculation of Geochemical Reactions in Brines" (PDF). Water-Resources Investigation Report (88–4153): 8. Retrieved 28 November 2015.
- Harvie, Charles E.; Weare, John H. (July 1980). "The prediction of mineral solubilities in natural waters: the Na-K-Mg-Ca-Cl-SO4-H2O system from zero to high concentration at 25 °C". Geochimica et Cosmochimica Acta. 44 (7): 981–997. Bibcode:1980GeCoA..44..981H. doi:10.1016/0016-7037(80)90287-2.
- Bhattacharia, Sanjoy K.; Tanveer, Sheik; Hossain, Nazir; Chen, Chau-Chyun (October 2015). "Thermodynamic modeling of aqueous Na+–K+–Mg2+–SO42− quaternary system". Fluid Phase Equilibria. 404: 141–149. doi:10.1016/j.fluid.2015.07.002.
- Thompson, John M.A. (1992). Manual of curatorship : a guide to museum practice (2nd ed.). Oxford: Butterworth-Heinemann. p. 431. ISBN 978-0750603515. Retrieved 24 November 2015.
- Whetham, William Cecil Dampier (1902). A Treatise on the Theory of Solutions. Cambridge Natural Science Manuals. Cambridge: The University Press. pp. 403–406. Retrieved 23 November 2015.
- Wollmann, Georgia; Voigt, Wolfgang (May 2010). "Solid–liquid phase equilibria in the system K2SO4–MgSO4–H2O at 318K". Fluid Phase Equilibria. 291 (2): 151–153. doi:10.1016/j.fluid.2009.12.005.
- Susarla, V. R. K. S.; Seshadri, K. (August 1982). "Equilibria in the system containing chloride and sulphates of potassium and magnesium". Proceedings of the Indian Academy of Sciences - Chemical Sciences. 91 (4): 315–320. doi:10.1007/BF02842643 (inactive 2020-04-29).
- M'nif, A.; Rokbani, R. (January 2004). "Minerals successions crystallisation related to tunisian natural brines". Crystal Research and Technology. 39 (1): 40–49. doi:10.1002/crat.200310147.
- Butts, D.S. (June 1980). "Chemistry of Great Salt Lake Brines in Solar Ponds". In Wallace Gwynn, J. (ed.). Great Salt Lake, a Scientific, Historical, and Economic Overview. Utah Geological Survey. p. 172. ISBN 9781557910837.
- Dhandapani, M.; Thyagu, L.; Prakash, P. Arun; Amirthaganesan, G.; Kandhaswamy, M. A.; Srinivasan, V. (April 2006). "Synthesis and characterization of potassium magnesium sulphate hexahydrate crystals". Crystal Research and Technology. 41 (4): 328–331. doi:10.1002/crat.200510582.
- Song, Yuehua; Xia, Shupin; Wang, Haidong; Gao, Shiyang (July 1995). "Thermal behavior of double salt schoenite". Journal of Thermal Analysis. 45 (1–2): 311–316. doi:10.1007/bf02548695.
- Meyerhoffer, Wilhelm; Cottrell, F. G. (1901). "An Acid Triple Salt". Journal of the Chemical Society, Abstracts. 80: 552. doi:10.1039/CA9018005548. Originally in Zeit. Anorg. Chem. 1901, 27, 442-444.
- US 3726965, F. Andreasen & U. Neitzel, "Production of langbeinite from a potassium magnesium sulfate salt and magnesium sulfate", published 10 April 1973
- Kirk, Raymond Eller; Othmer, Donald Frederick (1995). Kirk-Othmer Encyclopedia of Chemical Technology Volume 19 Pigments to Powders, Handling (4th ed.). John Wiley. p. 531.
- US 4195070, Ronald J. Allain; David G. Braithwaite & Joseph P. Maniscalco, "Preparation of a MgCl2 solution for Nalco's MgCl2 process from MgSO4 and other MgSO4 salts", published 25 March 1980
- US 3082061, Raymond L. Barry & Woodrow W. Richardson, "Production of potassium fluosilicate", published 19 March 1960
- US 3533735, Jerome A. Lukes, "Production of potassium chloride from schoenite and from brines containing potassium, magnesium, chloride and sulfate", published 13 October 1970
- Stewart, Frederick H. (1963). "Y. Marine Evaporites" (PDF). In Fleischer, Michael (ed.). Data of Geochemistry (6 ed.). Washington: United States Government Printing Office. p. Y10–Y25.
- Iglesrud, Iver (June 1932). "Formation of Oceanic Salt Deposits". Physics of the Earth V Oceanography. Washington DC: National Research Council of the National Academy of Sciences. pp. 184–195.
- Onac, B. P.; White, W. B.; Viehmann, I. (February 2001). "Leonite [K2Mg(SO4)2·4H2O], konyaite [Na2Mg(SO4)2·5H2O] and syngenite [K2Ca(SO4)2·H2O] from Tausoare Cave, Rodnei Mts, Romania". Mineralogical Magazine. 65 (1): 103–109. Bibcode:2001MinM...65..103O. doi:10.1180/002646101550154.
- Snow, Michael; Pring, Allan; Allen, Nicole (November 2014). "Minerals of the Wooltana Cave, Flinders Ranges, South Australia". Transactions of the Royal Society of South Australia. 138 (2): 214–230. doi:10.1080/03721426.2014.11649009.
- Lane, M. D.; Bishop, J. L.; Darby Dyar, M.; King, P. L.; Parente, M.; Hyde, B. C. (1 May 2008). "Mineralogy of the Paso Robles soils on Mars". American Mineralogist. 93 (5–6): 728–739. Bibcode:2008AmMin..93..728L. doi:10.2138/am.2008.2757. Retrieved 14 November 2015.
- Zolotov, M. Yu.; Shock, E. L. (2000). "Thermodynamic Stability of Hydrated Salts on the Surface of Europa" (PDF). Lunar and Planetary Science. XXXI: 1843. Bibcode:2000LPI....31.1843Z.
- Woisetschläger, Gebhard; Dutz, Myriam; Paul, Sabine; Schreiner, Manfred (27 November 2000). "Weathering Phenomena on Naturally Weathered Potash-Lime-Silica-Glass with Medieval Composition Studied by Secondary Electron Microscopy and Energy Dispersive Microanalysis". Microchimica Acta. 135 (3–4): 121–130. doi:10.1007/s006040070001.
- Foot, D. G.; Huiatt, J. L.; Froisland, L. J. (1984). Potash Recovery from Process and Waste Brines by Solar Evaporation and Flotation (PDF). Bureah of Mines, United States Department of Interior. p. 2.
- Ping-Yü, Ho; Gwei-Djen, Lu; Needham, Joseph (1976). Science and civilisation in China (Reprinted ed.). Cambridge: Cambridge University Press. pp. 75–98. ISBN 978-0521210287.
- Giester, Gerald; Rieck, Branko (19 May 1995). "Mereiterite, K2Fe[SO4]2·4H2O, a new leonite-type mineral from the Lavrion Mining District, Greece". European Journal of Mineralogy. 7 (3): 559–566. Bibcode:1995EJMin...7..559G. doi:10.1127/ejm/7/3/0559.
- Seaborg, Glenn T. (1994). "Terminology of the transuranium elements". Terminology. 1 (2): 229–252. doi:10.1075/term.1.2.02sea.
External links
![]() |
Wikimedia Commons has media related to Leonite. |
- "Aqueous Salt Solutions The MgSO4-K2SO4-H2O system".
- Starrs, B. A.; Storch, H. H. (January 1929). "The Ternary System: Potassium Sulphate-Magnesium Sulphate-Water". The Journal of Physical Chemistry. 34 (10): 2367–2374. doi:10.1021/j150316a019. public domain but paywalled
- Madsen, Beth M. (1966). "Loweite, Vanthoffite, Bloedite, and Leonite from Southeastern New Mexico". Geological Survey Professional Paper. 550 (2): B125–B129. Retrieved 14 November 2015.
- Eberhard, Usdowski; Bach, Martin F. (1998). Atlas and Data of Solid-Solution Equilibria of Marine Evaporites. Springer Science & Business Media. p. 263. doi:10.1007/9783642602849 (inactive 2020-04-29). ISBN 9783642643354. includes 3D diagram of temperature vs Mg/K and Cl/SO4 with leonite showing up as a lozenge shaped cylinder