Twistronics
Twistronics (from twist and electronics) is the study of how the angle (the twist) between layers of two-dimensional materials can change their electrical properties.[1][2] Materials such as bilayer graphene have been shown to have vastly different electronic behavior, ranging from non-conductive to superconductive, that depends sensitively on the angle between the layers.[3][4] The term was first introduced by the research group of Efthimios Kaxiras at Harvard University in their theoretical treatment of graphene superlattices.[1][5]
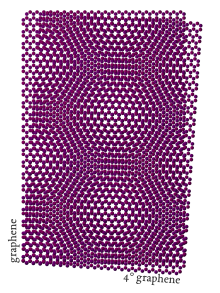
History
In 2007, National University of Singapore physicist Antonio Castro Neto hypothesized that pressing two misaligned graphene sheets together might yield new electrical properties, and separately proposed that graphene might offer a route to superconductivity, but he did not combine the two ideas.[4] In 2010 researchers from Universidad Técnica Federico Santa María in Chile found that for a certain angle close to 1 degree the band of the electronic structure of twisted bilayer graphene became completely flat[6], they suggested that collective behavior might be possible. In 2011 Allan MacDonald and Rafi Bistritzer using a simple theoretical model found that for the previously found "magic angle" the amount of energy a free electron would require to tunnel between two graphene sheets radically changes. In 2017, the research group of Efthimios Kaxiras at Harvard University used detailed quantum mechanics calculations to reduce uncertainty in the twist angle between two graphene layers that can induce extraordinary behavior of electrons in this two-dimensional system.[1]
Publication of these discoveries has generated a host of theoretical papers seeking to understand and explain the phenomena[7] as well as numerous experiments[3] using varying numbers of layers, twist angles and other materials.[4][8]
Characteristics
Superconduction and Insulation
The theoretical predictions of superconductivity were confirmed by Pablo Jarillo-Herrero and his student Yuan Cao of MIT and colleagues from Harvard University and the National Institute for Materials Science in Tsukuba, Japan. In 2018 they verified that superconductivity existed in bilayer graphene where one layer was rotated by an angle of 1.1° relative to the other, forming a moiré pattern, at a temperature of 1.7 K (−271.45 °C; −456.61 °F).[2][9][10] They created two bilayer devices that acted as an insulator instead of a conductor under a magnetic field. Increasing the field strength turned the second device into a superconductor.
A further advance in twistronics is the discovery of a method of turning the superconductive paths on and off by application of a small voltage differential.[11]
Heterostructures
Experiments have also been done using combinations of graphene layers with other materials that form heterostructures in the form of atomically thin sheets that are held together by the weak Van der Waals force.[12] For example, a study published in Science in July 2019 found that with the addition of a boron nitride lattice between two graphene sheets, unique orbital ferromagnetic effects were produced at a 1.17° angle, which could be used to implement memory in quantum computers.[13] Further spectroscopic studies of twisted bilayer graphene revealed strong electron-electron correlations at the magic angle.[14]
Electron Puddling
Between 2-D layers for bismuth selenide and a dichalcogenide, researchers at the Northeastern University in Boston, discovered that at a specific degrees of twist a new lattice layer, consisting of only pure electrons, would develop between the two 2-D elemental layers.[15] The quantum and physical effects of the alignment between the two layers appears to create "puddle" regions which trap electrons into a stable lattice. Because this stable lattice consists only of electrons, it is the first non-atomic lattice observed and suggests new opportunities to confine, control, measure, and transport electrons.
Ferromagnetism
A three layer construction, consisting of two layers of graphene with a 2-D layer of boron nitride, has been shown to exhibit superconductivity, insulation and ferromagnetism.[16]
References
- Carr, Stephen; Massatt, Daniel; Fang, Shiang; Cazeaux, Paul; Luskin, Mitchell; Kaxiras, Efthimios (2017-02-17). "Twistronics: Manipulating the Electronic Properties of Two-dimensional Layered Structures through their Twist Angle". Physical Review B. 95 (7): 075420. arXiv:1611.00649. doi:10.1103/PhysRevB.95.075420. ISSN 2469-9950.
- Jarillo-Herrero, Pablo; Kaxiras, Efthimios; Taniguchi, Takashi; Watanabe, Kenji; Fang, Shiang; Fatemi, Valla; Cao, Yuan (2018-03-06). "Magic-angle graphene superlattices: a new platform for unconventional superconductivity". Nature. 556 (7699): 43–50. arXiv:1803.02342. doi:10.1038/nature26160. PMID 29512651.
- Gibney, Elizabeth (2019-01-02). "How 'magic angle' graphene is stirring up physics". Nature. 565 (7737): 15–18. Bibcode:2019Natur.565...15G. doi:10.1038/d41586-018-07848-2. PMID 30602751.
- Freedman, David H. (2019-04-30). "How Twisted Graphene Became the Big Thing in Physics". Quanta Magazine. Retrieved 2019-05-05.
- Tritsaris, Georgios A.; Carr, Stephen; Zhu, Ziyan; Xie, Yiqi; Torrisi, Steven B.; Tang, Jing; Mattheakis, Marios; Larson, Daniel; Kaxiras, Efthimios (2020-01-30). "Electronic structure calculations of twisted multi-layer graphene superlattices". arXiv:2001.11633 [cond-mat.mes-hall].doi:10.1088/2053-1583/ab8f62
- Suárez Morell, E.; Correa, J. D.; Vargas, P.; Pacheco, M.; Barticevic, Z. (2010-09-13). "Flat bands in slightly twisted bilayer graphene: Tight-binding calculations". Physical Review B. 82 (12): 121407. doi:10.1103/PhysRevB.82.121407. ISSN 1098-0121.
- Freedman, David H. (2019-05-28). "What's the Magic Behind Graphene's 'Magic' Angle?". Quanta Magazine. Retrieved 2019-05-28.
- "Experiments explore the mysteries of 'magic' angle superconductors". phys.org. 2019-07-31. Retrieved 2019-07-31.
- Cao, Yuan; Fatemi, Valla; Demir, Ahmet; Fang, Shiang; Tomarken, Spencer L.; Luo, Jason Y.; Sanchez-Yamagishi, Javier D.; Watanabe, Kenji; Taniguchi, Takashi (2018-04-01). "Correlated insulator behaviour at half-filling in magic-angle graphene superlattices". Nature. 556 (7699): 80–84. arXiv:1802.00553. Bibcode:2018Natur.556...80C. doi:10.1038/nature26154. ISSN 0028-0836. PMID 29512654.
- Wang, Brian (2018-03-07). "Graphene superlattices could be used for superconducting transistors". NextBigFuture.com. Retrieved 2019-05-03.
- "Twisted physics: Magic angle graphene produces switchable patterns of superconductivity". phys.org. October 30, 2019. Retrieved 2020-02-06.
- University of Sheffield (March 6, 2019). "1 + 1 does not equal 2 for graphene-like 2-D materials". phys.org. Retrieved 2019-08-01.
- Than, Ker (2019-07-26). "Physicists discover new quantum trick for graphene: magnetism". phys.org. Retrieved 2019-07-27.
- Scheurer, Mathias S. (2019-07-31). "Spectroscopy of graphene with a magic twist". Nature. 572 (7767): 40–41. Bibcode:2019Natur.572...40S. doi:10.1038/d41586-019-02285-1.
- "Physicists may have accidentally discovered a new state of matter". phys.org. Retrieved 2020-02-27.
- "A talented 2-D material gets a new gig". phys.org. Retrieved 2020-03-04.