Mammatus cloud
Mammatus (mamma[1] or mammatocumulus), meaning "mammary cloud", is a cellular pattern of pouches hanging underneath the base of a cloud, typically cumulonimbus rainclouds, although they may be attached to other classes of parent clouds. The name mammatus is derived from the Latin mamma (meaning "udder" or "breast"). According to the WMO International Cloud Atlas, mamma is a cloud supplementary feature rather than a genus, species or variety of cloud. They are formed by cold air sinking down to form the pockets contrary to the puffs of clouds rising through the convection of warm air. These formations were first described in 1894 by William Clement Ley.[1][2][3]
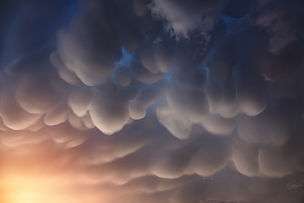
Characteristics
Mammatus are most often associated with anvil clouds and also severe thunderstorms. They often extend from the base of a cumulonimbus, but may also be found under altostratus, and cirrus clouds, as well as volcanic ash clouds.[4] When occurring in cumulonimbus, mammatus are often indicative of a particularly strong storm. Due to the intensely sheared environment in which mammatus form, aviators are strongly cautioned to avoid cumulonimbus with mammatus as they indicate convectively induced turbulence.[5] Contrails may also produce lobes but these are incorrectly termed as mammatus.[1]
Mammatus may appear as smooth, ragged or lumpy lobes and may be opaque or translucent. Because mammatus occur as a grouping of lobes, the way they clump together can vary from an isolated cluster to a field of mammae that spread over hundreds of kilometers to being organized along a line, and may be composed of unequal or similarly-sized lobes. The individual mammatus lobe average diameters of 1–3 kilometres (0.6–1.9 mi) and lengths on average of 1⁄2 kilometre (0.3 mi). A lobe can last an average of 10 minutes, but a whole cluster of mamma can range from 15 minutes to a few hours. They are usually composed of ice, but also can be a mixture of ice and liquid water or be composed of almost entirely liquid water.
True to their ominous appearance, mammatus clouds are often harbingers of a coming storm or other extreme weather system. Typically composed primarily of ice, they can extend for hundreds of miles in each direction and individual formations can remain visibly static for ten to fifteen minutes at a time. While they may appear foreboding they are merely the messengers - appearing around, before or even after severe weather.
Hypothesized formation mechanisms
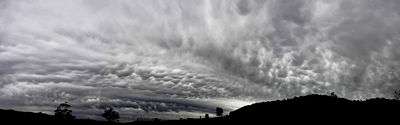
The existence of many different types of mammatus clouds, each with distinct properties and occurring in distinct environments, has given rise to multiple hypotheses on their formation, which are also relevant to other cloud forms.[4][6]
One environmental trend is shared by all of the formation mechanisms hypothesized for mammatus clouds: sharp gradients in temperature, moisture and momentum (wind shear) across the anvil cloud/sub-cloud air boundary, which strongly influence interactions therein. The following are the proposed mechanisms, each described with its shortcomings:
- The anvil of a cumulonimbus cloud gradually subsides as it spreads out from its source cloud. As air descends, it warms. However, the cloudy air will warm more slowly (at the moist adiabatic lapse rate) than the sub-cloud, dry air (at the dry adiabatic lapse rate). Because of the differential warming, the cloud/sub-cloud layer destabilizes and convective overturning can occur, creating a lumpy cloud-base. The problems with this theory are that there are observations of mammatus lobes that do not support the presence of strong subsidence in the lobes, and that it is difficult to separate the processes of hydrometeor fallout and cloud-base subsidence, thus rendering it unclear as to whether either process is occurring.
- Cooling due to hydrometeor fallout is a second proposed formation mechanism. As hydrometeors fall into the dry sub-cloud air, the air containing the precipitation cools due to evaporation or sublimation. Being now cooler than the environmental air and unstable, they descend until in static equilibrium, at which point a restoring force curves the edges of the fallout back up, creating the lobed appearance. One problem with this theory is that observations show that cloud-base evaporation does not always produce mammatus. This mechanism could be responsible for the earliest stage of development, but other processes (namely process 1, above) may come into play as the lobes are formed and mature.
- There may also be destabilization at cloud base due to melting. If the cloud base exists near the freezing line, then the cooling in the immediate air caused by melting can lead to convective overturning, just as in the processes above. However, this strict temperature environment is not always present.
- The above processes specifically relied on the destabilization of the sub-cloud layer due to adiabatic or latent heating effects. Discounting the thermodynamical effects of hydrometeor fallout, another mechanism proposes that dynamics of the fallout alone are enough to create the lobes. Inhomogeneities in the masses of the hydrometeors along the cloud-base may cause inhomogeneous descent along the base. Frictional drag and associated eddy-like structures create the lobed appearance of the fallout. The main shortcoming of this theory is that vertical velocities in the lobes have been observed to be greater than the fall speeds of the hydrometeors within them; thus, there should be a dynamical downward forcing, as well.
- Another method, that was first proposed by Kerry Emanuel, is called cloud-base detrainment instability (CDI), which acts very much like convective cloud-top entrainment. In CDI, cloudy air is mixed into the dry sub-cloud air rather than precipitating into it. The cloudy layer destabilizes due to evaporative cooling and mammatus are formed.
- Clouds undergo thermal reorganization due to radiative effects as they evolve. There are a couple of ideas as to how radiation can cause mammatus to form. One is that, because clouds radiatively cool (Stefan-Boltzmann law) very efficiently at their tops, entire pockets of cool, negatively buoyant cloud can penetrate downward through the entire layer and emerge as mammatus at cloud-base. Another idea is that as the cloud-base warms due to radiative heating from land surface's longwave emission, the base destabilizes and overturns. This method is valid for only optically thick clouds. However, the nature of anvil clouds is that they are largely made up of ice, and are therefore relatively optically thin.
- Gravity waves are proposed to be the formation mechanism of linearly organized mammatus clouds. Indeed, wave patterns have been observed in the mammatus environment, but this is mostly due to gravity wave creation as a response to a convective updraft impinging upon the tropopause and spreading out in wave form over the entirety of the anvil. Therefore, this method does not explain the prevalence of mammatus clouds in one part of the anvil versus another. Furthermore, time and size scales for gravity waves and mammatus do not match up entirely. Gravity wave trains may be responsible for organizing the mammatus rather than forming them.[7]
- Kelvin–Helmholtz (K-H) instability is prevalent along cloud boundaries and results in the formation of wave-like protrusions (called Kelvin-Helmholtz billows) from a cloud boundary. Mammatus are not in the form of K-H billows, thus, it is proposed that the instability can trigger the formation of the protrusions, but that another process must form the protrusions into lobes. Still, the main downfall with this theory is that K-H instability occurs in a stably stratified environment, and the mammatus environment is usually at least somewhat turbulent.
- Rayleigh–Taylor instability is the name given to the instability that exists between two fluids of differing densities, when the denser of the two is atop the less dense fluid. Along a cloud-base/sub-cloud interface, the denser, hydrometeor-laden air could cause mixing with the less-dense sub-cloud air. This mixing would take the form of mammatus clouds. The physical problem with this proposed method is that an instability existing along a static interface cannot necessarily be applied to the interface between two sheared atmospheric flows.
- The last proposed formation mechanism is that mammatus arise from Rayleigh-Bénard convection, where differential heating (cooling at the top and heating at the bottom) of a layer causes convective overturning. However, in this case of mammatus, the base is cooled by thermodynamical mechanisms mentioned above. As the cloud base descends, it happens on the scale of mammatus lobes, while adjacent to the lobes, there is a compensating ascent. This method has not proven to be observationally sound and is viewed as generally insubstantial.
This plenitude of proposed formation mechanisms shows, if nothing else, that the mammatus cloud is generally poorly understood.[1][8]
Gallery
- Mammatus clouds over U.S. Air Force Academy, 2004
- Mammatus clouds over Croatia
- Mammatus clouds over New York City, 2009
- Mammatus clouds in Tulsa, Oklahoma, 1973
- Mammatus clouds over San Antonio, Texas, 2009
- Mammatus Clouds over the Pacific Coast San Francisco
- Mammatus clouds over Squaw Valley Ski Resort, Olympic Valley, California
- Mammatus clouds in Milan, Italy, in July 2005 on a very hot, humid day without wind
- Mammatus Clouds in San Francisco, California
- Mammatus clouds forming in Minnesota in 2005
- Mammatus clouds and crepuscular rays over San Francisco Bay
- Aerial photo of mammatus clouds over central New South Wales, Australia,
- Mammatus Clouds Over Sierras de Córdoba Mountains, Argentina
- Mammatus clouds over Santa Catarina, Brazil, following Cyclone Catarina.
- Cumulus Mammatus clouds between Hamilton and Missoula, Montana
- Mammatus clouds over the University of Central Florida in Orlando, Florida, 2011.
- Just before a large thunderstorm in Altomuenster, Bavaria, Germany
- Mammatus clouds over Bingley, UK, following a thunderstorm on 2 November 2013
- Mammatus Clouds over Hoshiarpur May 20, 2016, Punjab, India
- Mammatus Clouds observed in Norwich Norfolk, UK, 2015/05/24 just before sunset in the west moving east. Duration 17 minutes. Calm weather. No rain, 19 Celsius.
- Strikingly regular mammatus clouds on June 26, 2012 in Regina, Saskatchewan, following a severe storm warning and tornado watch.
- Mammatus clouds in Lithuania, 2016
References
- Schultz, David M.; Hancock, Y. (2016). "Contrail lobes or mamma? The importance of correct terminology" (PDF). Weather. 71 (8): 203. Bibcode:2016Wthr...71..203S. doi:10.1002/wea.2765.
- Anonymous (1975). International Cloud Atlas. Volume I. Manual on the observation of clouds and other Meteors (PDF). World Meteorological Organization. Archived from the original (PDF) on 2017-07-08. Retrieved 2017-05-13.
- Ley, William Clement, Cloudland: A study on the structure and characters of clouds (London, England: Edward Stanford, 1894), pp. 104–105.
- Schultz, David M.; Kanak, Katharine M.; Straka, Jerry M.; Trapp, Robert J.; Gordon, Brent A.; Zrnić, Dusan S.; Bryan, George H.; Durant, Adam J.; Garrett, Timothy J.; Klein, Petra M.; Lilly, Douglas K. (2006). "The Mysteries of Mammatus Clouds: Observations and Formation Mechanisms". Journal of the Atmospheric Sciences. 63 (10): 2409. Bibcode:2006JAtS...63.2409S. doi:10.1175/JAS3758.1.
- Lane, Todd P.; Sharman, Robert D.; Trier, Stanley B.; Fovell, Robert G.; Williams, John K. (2012). "Recent Advances in the Understanding of Near-Cloud Turbulence". Bulletin of the American Meteorological Society. 93 (4): 499. Bibcode:2012BAMS...93..499L. doi:10.1175/BAMS-D-11-00062.1.
- Garrett, Timothy J.; Schmidt, Clinton T.; Kihlgren, Stina; Cornet, Céline (2010). "Mammatus Clouds as a Response to Cloud-Base Radiative Heating". Journal of the Atmospheric Sciences. 67 (12): 3891. Bibcode:2010JAtS...67.3891G. doi:10.1175/2010JAS3513.1.
- Winstead, Nathaniel S.; Verlinde, J.; Arthur, S. Tracy; Jaskiewicz, Francine; Jensen, Michael; Miles, Natasha; Nicosia, David (2001). <0159:HRAROO>2.0.CO;2 "High-Resolution Airborne Radar Observations of Mammatus". Monthly Weather Review. 129 (1): 159–166. Bibcode:2001MWRv..129..159W. doi:10.1175/1520-0493(2001)129<0159:HRAROO>2.0.CO;2.
- Kanak, Katharine M.; Straka, Jerry M.; Schultz, David M. (2008). "Numerical Simulation of Mammatus". Journal of the Atmospheric Sciences. 65 (5): 1606. Bibcode:2008JAtS...65.1606K. CiteSeerX 10.1.1.720.2477. doi:10.1175/2007JAS2469.1.
External links
![]() |
Wikimedia Commons has media related to Mammatus clouds. |
- Forming Mammatus Clouds Time Lapse Video
- Mammatus clouds over Hastings, Nebraska
- Mammatus Clouds sagging pouch-like structures
- NASA Astronomy Picture of the Day: Mammatus Clouds Over Mexico (30 December 2007)
- Mammatus Clouds over St Albans, Hertfordshire, UK on 12 August 2008 at the BBC News web site. 21 August 2008
???