Dog coat genetics
Modern dog breeds have a wide range of coat colors, patterns, textures and lengths. Knowledge of the genetics of canine coat coloring and patterning[1] and coat texturing and length[2] has improved a great deal in recent years.
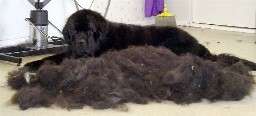
Dog coat color is governed by how genes are passed from dogs to their puppies and how those genes are expressed in each dog. Dogs have about 19,000 genes in their genome but only a handful affect the physical variations in their coats. And the usual rules apply—most genes come in pairs, one from the dog’s mother and one from its father. Genes of interest have more than one version, or allele. Usually only one or a small number of alleles exist for each gene. So, at any one gene locus a dog will either be homozygous, that is, the gene is made of two identical alleles (one from its mother and one its father) or heterozygous, that is, the gene is made of two different alleles (again, one inherited from each parent).
To understand why a dog’s coat looks the way it does based on its genes requires an understanding of a handful of particular dog coat genes and their alleles. For example, if you wanted to find out how a black and white greyhound that seems to have wavy hair got its coat, you would want to look into the dominant black gene with its K and k alleles, the (white) spotting gene with its multiple alleles, and the R and r alleles of the curl gene.
Genes associated with coat color
Each hair follicle is surrounded by many melanocytes (pigment cells), which make and transfer the pigment melanin into a developing hair. Dog fur is colored by two types of melanin: eumelanin (brownish-black) and phaeomelanin (reddish-yellow). A melanocyte can be signaled to produce either color of melanin.
The various dog coat colors are from patterns of:
- Eumelanin — black, chocolate brown, grey or taupe pigment;
- Phaeomelanin — tan pigment, including all shades of red, gold and cream pigment; and/or
- Lack of melanin — white (no pigment).
By 2020, more than eight genes in the canine genome have been verified to determine coat color. Each of these has at least two known alleles. Together these genes account for the variation in coat color seen in dogs. Each gene has a unique, fixed location, known as a locus, within the dog genome.
Some of the loci associated with canine coat color are:
Pigment shade
Several loci can be grouped as affecting the shade of color: the Brown (B), Dilution (D), and Intensity (I) loci.
B (brown) locus
The gene at the B locus is known as tyrosinase related protein 1 (TYRP1). This gene affects the color of the eumelanin pigment produced, making it either black or brown. TYRP1 is an enzyme involved in the synthesis of eumelanin. Each of the known mutations appears to eliminate or significantly reduce TYRP1 enzymatic activity.[3] This modifies the shape of the final eumelanin molecule, changing the pigment from a black to a brown color. Color is affected in coat and skin (including the nose and paw pads).[4]
There are four known alleles that occur at the B locus:
- B = Black eumelanin. An animal that has at least one copy of the B allele will have a black nose, paw pads and eye rims and (usually) dark brown eyes.
- b = Brown eumelanin - such as chocolate or liver (includes several alleles - bs, bd and bc). An animal with any matched or unmatched pair of the b alleles will have brown, rather than black, hair, a liver nose, paw pads and eye rims, and hazel eyes. Phaeomelanin color is unaffected.[3] Only one of the alleles is present in the English Setter (bs), Doberman Pinscher (bd) and Italian Greyhound (bc), but in most breeds with any brown allele 2 or all 3 are present.[5] It is unknown whether the different brown alleles cause specific shades or hues of brown.
B is dominant to b.
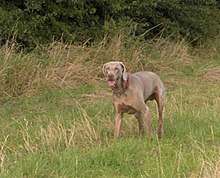
D (dilute) locus
The melanophilin gene (MLPH) at the D locus causes a dilution of both eumelanin and phaeomelanin and determines the intensity of pigmentation.[7] MLPH codes for a protein involved in the distribution of melanin - it is part of the melanosome transport complex. Defective MLPH prevents normal pigment distribution, resulting in a paler colored coat.[8]
There are two common alleles: D (normal, wild-type MLPH), and d (defective MLPH) that occur in many breeds. But recently the research group of Tosso Leeb has identified additional alleles in other breeds.
- D = Not diluted. Black or brown eumelanin (as determined by Brown locus), reddish or orangish tan phaeomelanin.
- d = Diluted. Diluted fur color: black eumelanin (B/-) diluted to bluish grey (ranging from light blue-grey to dark steel); brown eumelanin (b/b) diluted to taupe or "Isabella". Phaeomelanin is diluted from red to yellowish tan; this phaeomelanin dilution is not as dramatic as the eumelanin color shift.[9] Slight to moderate dilution of the paw pads and eye rims towards bluish grey if B/- or taupe if b/b, and slight to moderate reduction of eye color from brown towards amber in a B/- animal, or from hazel towards light amber in a b/b animal.
D is completely dominant to d.
Homozygosity of d is sometimes accompanied by hair loss and recurrent skin inflammation, a condition referred to as either color dilution alopecia (CDA) or black hair follicular dysplasia (BHFD) depending upon the breed of dog.[7]
Color gene interactions
COLOUR GENE INTERACTIONS[10] |
Not Dilute (D/D or D/d) |
Dilute (d/d) |
Black B/B or B/b |
Black eumelanin Red* phaeomelanin |
Blue-grey eumelanin Yellow phaeomelanin |
Brown b/b |
Chocolate-brown eumelanin Red* phaeomelanin[3] |
Taupe or "Isabella" eumelanin Yellow phaeomelanin |
* Note that phaeomelanin is frequently diluted by intensity factor of theoretical I locus. |
I (intensity) locus
The alleles at the theoretical I locus are thought to affect phaeomelanin expression. Two alleles are theorised to occur at the I locus:
- I = Intense red, not diluted
- i = Not intense red
It is thought that I and i interact with semi-dominance, so that there are three distinct phenotypes. I/i heterozygotes are paler than I/I animals but darker than i/i animals.
- i results in light-coloured phaeomelanin such as gold, yellow, buff and apricot. This gene is the most common cause of lighter tans, and unlike d/d, it allows the skin and eyes to remain dark.
This gene was found to be MFSD12 in 2019 [11]. It occurs in many different breeds and causes dogs to be cream instead of red. It can also affect only the areas of a dog that would have been reddish and not affect the black areas, i.e. leaving a cream Afghan with a very black mask.
Pigment type
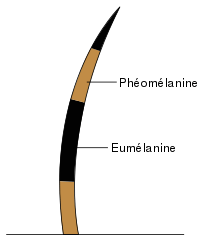
Several loci can be grouped as controlling when and where on a dog eumelanin (blacks-browns) or phaeomelanin (reds-yellows) are produced: the Agouti (A), Extension (E) and Black (K) loci. [3] Intercellular signaling pathways tell a melanocyte which type of melanin to produce. Time-dependent pigment switching can lead to the production of a single hair with bands of eumelanin and phaeomelanin.[12] Spatial-dependent signaling results in parts of the body with different levels of each pigment.
MC1R (the E locus) is a receptor on the surface of melanocytes. When active, it causes the melanocyte to synthesize eumelanin; when inactive, the melanocyte produces phaeomelanin instead. ASIP (the A locus) binds to and inactivates MC1R, thereby causing phaeomelanin synthesis. DEFB103 (the K locus) in turn prevents ASIP from inhibiting MC1R, thereby increasing eumelanin synthesis. [12]
A (agouti) locus
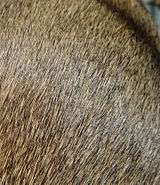
The alleles at the A locus are related to the production of agouti signalling protein (ASIP) and determine whether an animal expresses an agouti appearance, and, by controlling the distribution of pigment in individual hairs, what type of agouti. There are four known alleles that occur at the A locus:
- Ay = Fawn or sable. Tan with black whiskers and varying amounts of black-tipped and/or all-black hairs dispersed throughout. Fawn typically referring to dogs with clearer tan and sable to those with more black shading.
- aw = Wild-type agouti. Each hair with 3-6 bands alternating black and tan. Also called wolf sable.
- at = Tan point. Black with tan patches on the face and underside - including saddle tan (tan with a black saddle or blanket).[13] Phaeomelanin production is limited to tan points; dark portions of the dog are solid eumelanin hairs. [14]
- a = Recessive black. Solid black, inhibition of phaeomelanin.
- ayt = Recombinant fawn (black with tan patches on the face and underside) has been identified in numerous Tibetan Spaniels and individuals in other breeds, including the Dingo. Its hierarchical position is not yet understood.[15][16]
Most texts suggest that the dominance hierarchy for the A locus alleles appears to be as follows: Ay > aw > at > a; however, research suggests the existence of pairwise dominance/recessiveness relationships in different families and not the existence of a single hierarchy in one family.[19]
- Ay is incompletely dominant to at, so that heterozygous individuals have more black sabling, especially as puppies and Ayat can resemble the awaw phenotype. Other genes also affect how much black is in the coat.
- aw is the only allele present in many Nordic spitzes, and is not present in most other breeds.
- at includes tan point and saddle tan, both of which look tan point at birth. Modifier genes in saddle tan puppies cause a gradual reduction of the black area until the saddle tan pattern is achieved.
- a is only present in a handful of breeds. Most black dogs are black due to the K locus allele KB for dominant black.[20]
E (extension) locus
The alleles at the E locus (the melanocortin receptor one gene or MC1R) determine whether an animal expresses a melanistic mask, as well as determining whether an animal can produce eumelanin in its coat. There are three known, plus two more theorized, alleles that occur at the E locus:
- Em = Mask (a eumelanin mask is added to the face). The distribution of the pigments on the rest of the face and on the body is determined by the agouti locus.
- EG = Grizzle (if atat and not KB/-, tan underparts with a dark overlay covering the top and sides of the body, head and tail, and the outside of the limbs) - also called domino.
- E = Normal extension (pattern expressed as per alleles present at A and K loci).
- eh = Cocker sable (if KB/- and may require atat, tan with a dark overlay covering the top and sides of the body, head and tail, and the outside of the limbs).
- e = Recessive or clear fawn (tan, inhibition of eumelanin).[21]
- Em allows the production of black and chocolate brown eumelanin in the fur and causes the melanistic mask.
- Dogs with Genotype EE or Ee can produce black or chocolate brown eumelanin for the fur.
- Dogs with Genotype ee can only store pheomelanin in the fur. BB or Bb on the B locus still allows a black nose.
- Homozygous ee causes red or yellow fur. Eumelanin can be in nose, eye lids and paw pads but not in the fur.
- Genotyp ee and bb for brown eumelanin causes red fur and liver-nose.
- In dogs with recessive red the Merle factor can be hidden, as they don't have eumelanin in the fur.
The dominance hierarchy for the E locus alleles appears to be as follows: Em > EG > E > eh > e.
- E allows normal expression of eumelanin and/or phaeomelanin according to the alleles present at the A and K loci.
- Em allows similar pattern expression to E except any tan (phaeomelanin) areas on the mask area are replaced with eumelanin (black/etc.) The mask can vary from the muzzle, to the face and ears, to a larger area with shading on the front and sides as in the Belgian Tervuren. The mask Em is unaffected by the greying gene G and will remain dark in a G/- animal while the rest of the dog pales, such as in Kerry Blue Terriers. Some puppies are born with a mask which fades away within a few weeks of birth: these puppies do not have the Em allele and their temporary mask is due to sabling.
- An animal that is homozygous for e will express a red to yellow coat regardless of most alleles at other loci. Eumelanin is inhibited, so there can be no black hairs anywhere, even the whiskers. Pigment on the nose leather can be lost at the middle (Dudley nose). In combination with a/a (phaeomelanin inhibitor), an e/e dog will be white to off-white; in combination with U/U or U/u, an e/e dog will be off-white or cream.[22]
- The Grizzle allele has been studied only in Salukis and Afghan Hounds, the latter in which it is referred to as "Domino", but also occurs in the Borzoi. Its placement in the dominance hierarchy has not been solidified. Black with fawn-tan points (at/at E/-) is instead dark-sable with extended clear-tan points (at/at EG/-). Brindle affects fawn and sable areas, resulting in black with bridled-tan points (at/at E/- Kbr/-) or brindle with clear-tan points (at/at EG/- Kbr/-). Expression of EG is dependent upon the animal being homozygous for at and not possessing Em or KB.[23] EG is theorized to have no effect on the phenotype of non-at/- nor KB dogs and to be allelic to Em and e.
- The eh sable extension allele has been studied only in English Cocker Spaniels and produces sable in the presence of dominant black KB and tan point at/at. Its expression is dependent upon the animal not possessing Em nor E nor being homozygous for e. eh is theorized to be on the E locus and to have no effect on ky/ky dogs. All cocker spaniels are homozygous for at, so it is unknown how the gene may function in the presence of other A-series alleles.
K (dominant black) locus
The alleles at the K locus (the β-Defensin 103 gene or DEFB103) determine the coloring pattern of an animal's coat.[24] There are three known alleles that occur at the K locus:
- KB = Dominant black (black)
- kbr = Brindle (black stripes added to tan areas)
- ky = Phaeomelanin permitted (pattern expressed as per alleles present at A and E loci)
The dominance hierarchy for the K locus alleles appears to be as follows: KB > kbr > ky.
- KB causes a solid eumelanin coat (black, brown, grey or taupe) except when combined with e/e (tan or white), Eh/- (Cocker sable) or Em/- G/- and appropriate coat type (light eumelanin with dark eumelanin mask)
- kbr causes the addition of eumelanin stripes to all tan areas of a dog except when combined with e/e (no effect) or EG/- atat non-KB/- (eumelanin and sabled areas become striped, tan areas remain tan)
- ky is wild-type allowing full expression of other genes.
Interactions of some genes with brindle
Alleles at the Agouti (A), Extension (E) and Black (K) loci determine the presence or absence of brindle and its location:
Brindle INTERACTIONS[10] |
Fawn or Sable Ay/- |
Wolf Sable aw/aw, aw/at or aw/a |
Tan Point at/at or at/a |
Rec. Black a/a | |
Dom. Black KB/- |
Mask Em/- |
black (with mask)* |
black (with mask)* |
black (with mask)* |
black (with mask)* |
wildtype E E/E or E/e |
black | black | black | black | |
Cocker Sable† eh/eh or eh/e |
? | ? | cocker sable | ? | |
Brindle Kbr/Kbr or Kbr/ky |
Mask Em/- |
brindle with mask |
brindle with mask |
black & brindled tan with mask |
black (with mask)* |
wildtype E E/E or E/e |
brindle | brindle | black & brindled tan | black | |
Grizzle/Domino† EG/EG, EG/E or EG/e |
brindle (Afghan) | n/a | brindle points (Afghan) | n/a | |
wildtype K ky/ky |
Mask Em/- |
fawn or sable with mask |
wolf sable with mask |
black & tan with mask |
black (with mask)* |
wildtype E E/E or E/e |
fawn or sable | wolf sable | black & tan | black | |
Grizzle/Domino† EG/EG, EG/E or EG/e |
fawn | n/a | grizzle | n/a | |
any K -/- |
Clear Fawn e/e |
tan | tan | tan | white (Samoyed) |
† eh and EG are only included in the table where their interactions are known. |
Patches and white spotting
The Merle (M), Harlequin (H), and Spotting (S) loci contribute to patching, spotting, and white markings. Alleles present at the Merle (M) and Harlequin (H) loci cause patchy reduction of melanin to half (merle), zero (harlequin) or both (double merle). Alleles present at the Spotting (S), Ticking (T) and Flecking (F) loci determine white markings.
H (harlequin) locus
DNA studies have isolated a missense mutation in the 20S proteasome β2 subunit at the H locus.[25] The H locus is a modifier locus (of the M locus) and the alleles at the H locus will determine if an animal expresses a harlequin vs merle pattern. There are two alleles that occur at the H locus:
- H = Harlequin (if M/-, patches of full colour and white)
- h = Non-harlequin (if M/-, normal expression of merle)
H/h heterozygotes are harlequin and h/h homozygotes are non-harlequin. Breeding data suggests that homozygous H/H is embryonic lethal and that therefore all harlequins are H/h.[26]
- The Harlequin allele is specific to Great Danes. Harlequin dogs (H/h M/m) have the same pattern of patches as merle (h/h M/m) dogs, but the patches are white and harlequin affects eumelanin and phaeomelanin equally. H has no effect on non-merle m/m dogs.
M (merle) locus
The alleles at the M locus (the silver locus protein homolog gene or SILV, aka premelanosome protein gene or PMEL) determine whether an animal expresses a merle pattern to its coat. There are two alleles that occur at the M locus:
- M = Merle (patches of full colour and reduced colour)
- m = Non-merle (normal expression)
M and m show a relationship of both co-dominance and no dominance.
- On heterozygous M/m merles, black is reduced to silver on ~50% of the animal in semi-random patches with rough edges like torn paper. The fraction of the dog covered by merle patches is random such that some animals may be predominantly black and others predominantly silver. The merle gene is “faulty” with many merle animals having one odd patch of a third shade of grey, brown or tan.
- On homozygous M/M “double merles”, black is replaced with ~25% black, ~50% silver and ~25% white, again with random variation, such that some animals have more black or more white.
- Eumelanin (black/etc.) is significantly reduced by M/m, but phaeomelanin is barely affected such that there will be little to no evidence of the merle gene on any tan areas or on an e/e dog. However, the white patches caused by M/M affect both pigments equally, such that a fawn double merle would be, on average, ~75% tan and ~25% white.
- The merle gene also affects the skin, eye colour, eyesight and development of the eye and inner ear. Merle M/m puppies develop their skin pigmentation (nose, paws, belly) with speckled-edged progression, equally evident in e/e merles except when extensive white markings cause pink skin to remain in these areas. Blue and part-blue eyes are common.
- Both heterozygosity and homozygosity of the merle gene (i.e., M/m and M/M) are linked to a range of auditory and ophthalmologic abnormalities.[27] Most M/m merles have normal-sized eyes and acceptably functional eyesight and hearing; most M/M double merles suffer from microphthalmia and/or partial to complete deafness.[28]
S (spotting) locus
The alleles at the S locus (the microphthalmia-associated transcription factor gene or MITF) determine the degree and distribution of white spotting on an animal's coat.[29] There is disagreement as to the number of alleles that occur at the S locus, with researchers sometimes postulating a conservative two[30] or, commonly, four[31] alleles. The alleles postulated are:
- S = Solid color/no white (very small areas of white may still appear; a diamond or medallion on the chest, a few toe tips/toes, or a tail tip)
- si = Irish-spotting (white on muzzle, forehead, feet, legs, chest, neck and tail)
- sp = Piebald (varies from coloured with Irish spotting plus at least one white marking on the top or sides of the body or hips, to mostly white which generally retains patches of colour around the eyes, ears and tail base)
- sw = Extreme piebald spotting (extremely large areas of white, almost completely white)
S is incomplete dominant (towards co-dominant) to sp. DNA studies have not yet confirmed the existence of all four alleles, with some research suggesting the existence of at least two alleles (S and sp)[29] and other research suggesting the possible existence of a third allele (si).[32]
- S/sp heterozygotes usually have some white at birth on the chest and toes, which may be covered by ticking as the puppy grows. Animals of this genotype also commonly display pseudo-Irish spotting; in fact most Irish-spotted dogs are so due to heterozygosity for solid and piebald.
- A few breeds (e.g., Boston Terrier) are fixed for Irish spotting and therefore theorized to possess a different allele on the S locus (si) or an allele on a completely separate gene.
- It has been suggested that what appears to be the result of an sw allele is in fact the result of plus and minus modifiers acting on one of the other alleles.[29] It is thought that the spotting that occurs in Dalmatians is the result of the interaction of three loci (the S locus, the T locus and F locus) giving them a unique spotting pattern not found in any other breed.[33]
- White spotting also affects skin, causing pink patches.
- White spotting can cause blue eyes, microphthalmia, blindness and deafness; however, because pigmentation is generally retained around the eye/ear area, this is rare except among sw/sw dogs (or extreme versions of sp/sp if sw does not exist).
In 2014, a study found that a simple repeat polymorphism in the MITF-M Promoter is a key regulator of white spotting and that white color had been selected for by humans.[34][35]
Albinism
C (colored) locus
Various people have postulated several alleles at the C locus and suggested some/all determine the degree to which an animal expresses phaeomelanin, a red-brown protein related to the production of melanin, in its coat and skin. Five alleles have been theorised to occur at the C locus:
- C = Full color (animal expresses phaeomelanin)
- cch = Chinchilla (partial inhibition of phaeomelanin resulting in decreased red pigment)
- ce = Extreme dilution (inhibition of phaeomelanin resulting in extremely reduced red pigment)
- cb, cp = Blue-eyed albino/Platinum (almost total inhibition of phaeomelanin resulting in near albino appearance)
- ca = Albino (complete inhibition of phaeomelanin production, resulting in complete inhibition of melanin production)
However, the C locus is now considered to be the gene SLC45A2 [36] based on publications about albinism in Doberman Pinschers originally, and later in other small breeds [37]. Please see also http://munster.sasktelwebsite.net/DogColor/white.html
Theoretical genes for color and pattern
There are additional theoretical loci thought to be associated with coat color in dogs. DNA studies are yet to confirm the existence of these genes or alleles but their existence is theorised based on breeding data:[38]
F (flecking) locus
The alleles at the theoretical F locus are thought to determine whether an animal displays small, isolated regions of white in otherwise pigmented regions (not apparent on white animals). Two alleles are theorised to occur at the F locus:
- F = Flecked
- f = Not flecked
(Please see ticking below, which may be another name for the flecking described here)
It is thought that F is dominant to f.[33]
G (progressive greying) locus
The alleles at the theoretical G locus are thought to determine if progressive greying of the animal's coat will occur. Two alleles are theorised to occur at the G locus:
- G = Progressive greying (melanin lost from hairs over time)
- g = No progressive greying
It is thought that G is dominant to g.
- The greying gene affects both eumelanin, and to a lesser extent phaeomelanin. In the presence of Em/- the eumelanin mask will be unaffected and remain dark. Grey dogs are born fully coloured and develop the greying effect over several months. New hairs are grown fully coloured but their colour fades over time towards white. Greying is most evident in continuous-growing coats (long + wire + curly) as individual hairs remain on the dog long enough for the colour to be lost. In short-haired dogs, hairs are shed out and re-grown before the colour has a chance to change.
- Premature greying, in which the face/etc. greys at a young age is not caused by G and has not been proven to be genetic.
T (ticking) locus
The alleles at the theoretical T locus are thought to determine whether an animal displays small, isolated regions of pigment in otherwise s-spotted white regions. Two alleles are theorised to occur at the T locus:
- T = Ticked
- t = Not ticked
It is thought that T is dominant to t. Ticking may be caused by several genes rather than just one. Patterns of medium-sized individual spots, smaller individual spots, and tiny spots that completely cover all white areas leaving a roan-like or merle-like appearance (reserving the term large spots for the variation exclusive to the Dalmatian) can each occur separately or in any combination.
- The effect of the ticking gene(s) is to add back little coloured spots to areas made white by piebald spotting (-/s) or the limited white markings of S/S animals. It does not affect white areas that were caused by a/a e/e or M/M or M/m H/h. The colour of the tick marks will be as expected or one shade darker. Tick marks are semi-random, so that they vary from one dog to the next and can overlap, but are generally present on the lower legs and heavily present on the nose.
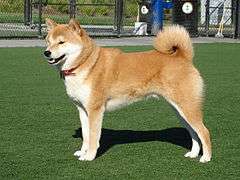
U (urajiro) locus
The alleles at the theoretical U locus are thought to limit phaeomelanin production on the cheeks and underside.[39] Two alleles are theorised to occur at the U locus:
- U = Urajiro
- u = Not urajiro
It is thought that U is dominant to u but incomplete with homozygosity required for complete dilution to off-white and heterozygotes displaying a darker cream. The urajiro pattern is expressed in the tan (phaeomelanin) areas of any dog who is not e/e. In e/e dogs, the urajiro gene causes dilution of the entire dog to off-white or cream.
Miscolours in dog breeds
Miscolours occur quite rarely in dog breeds, because genetic carriers of the recessive alleles causing fur colours that don't correspond to the breed standard are very rare in the gene pool of a breed and there is an extremely low probability that one carrier will be mated with another. In case two carriers have offspring, according to the law of segregation an average of 25% of the puppies are homozygous and express the off-colour in the phenotype, 50% become carriers and 25% are homozygous for the standard colour. Usually off-coloured individuals are excluded from breeding, but that doesn't stop the inheritance of the recessive allele from carriers mated with standard-coloured dogs to new carriers.
In the breed Boxer large white markings in heterzygous carriers with genotype S si or S sw belong to the standard colours, therefore extreme white Boxers are born regularly, some of them with health problems.[40] The cream-white colour of the Shiba Inu is not caused by any spotting gene but by strong dilution of pheomelanin. [41] Melanocytes are present in the whole skin and in the embryonic tissue for the auditory organs and eyes, therefore this colour is not associated with any health issues.
- Punnett square: Inheritance with one carrier of a recessive gene
- Litter of a Boxer Genotype S si mated with another si carrier.
- Punnett square: Inheritance with two genetic carriers
- Shiba Inu: According to the AKC cream-white is a non-standard colour[42] but is accepted by the British Kennel Club.[43]
- For normal Yorkshire Terriers Piebald spotting sp sp is not allowed. Tricolor Yorkies became a separate breed.
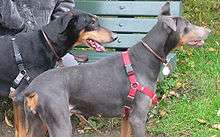
The occurrence of a dominant coat colour gene not belonging to the standard colours is a suspicion for crossbreeding with another breed. For example the dilute gen D in the suddenly appeared variety "silver coloured" Labrador Retriever might probably come from a Weimaraner.[45] The same applies for Dobermann Pinschers suffering from Blue dog syndrome.[46][47][48]
Genes associated with hair length, growth and texture
Every hair in the dog coat grows from a hair follicle, which has a three phase cycle, as in most other mammals. These phases are:
- anagen, growth of normal hair;
- catagen, growth slows, and hair shaft thins; and
- telogen, hair growth stops, the follicle rests, and the old hair falls off—is shed. At the end of the telogen phase, the follicle begins the cycle again.[49]
Most dogs have a double coat, each hair follicle containing 1-2 primary hairs and several secondary hairs. The primary hairs are longer, thicker and stiffer, and called guard hairs or outer coat. Each follicle also holds a variety of silky- to wiry-textured secondary hairs (undercoat) all of which are wavy, and smaller and softer than the primary hair. The ratio of primary to secondary hairs varies at least six-fold, and varies between dogs according to coat type, and on the same dog in accordance with seasonal and other hormonal influences.[50] Puppies are born with a single coat, with more hair follicles per unit area, but each hair follicle contains only a single hair of fine, silky texture. Development of the adult coat begins around 3 months of age, and is completed around 12 months.
Research indicates that the majority of variation in coat growth pattern, length and curl can be attributed to mutations in four genes, the R-spondin-2 gene or RSPO2, the fibroblast growth factor-5 gene or FGF5, the keratin-71 gene or KRT71[2] and the melanocortin 5 receptor gene (MC5R). The wild-type coat in dogs is short, double and straight.
L (length) locus
The alleles at the L locus (the fibroblast growth factor-5 gene or FGF5) determine the length of the animal's coat.[51] There are two known alleles that occur at the L locus:
- L = Short coat
- l = Long coat
L is dominant to l. A long coat is demonstrated when a dog has pair of recessive l alleles at this locus. The dominance of L > l is incomplete, and L/l dogs have a small but noticeable increase in length and finer texture than closely related L/L individuals. However, between breeds there is significant overlap between the shortest L/L and the longest L/l phenotypes. In certain breeds (German Shepherd, Alaskan Malamute, Cardigan Welsh Corgi), the coat is often of medium length and many dogs of these breeds are also heterozygous at the L locus (L/l).
W (wired) locus

The alleles at the W locus (the R-spondin-2 gene or RSPO2) determine the coarseness and the presence of "facial furnishings" (e.g. beard, moustache, eyebrows).[2] There are two known alleles that occur at the W locus:
- W = Wire (hair is coarse and facial furnishings present)
- w = Non-wire (hair is not coarse and facial furnishings are not present)

W is dominant to w, but the dominance of W > w is incomplete. W/W dogs have coarse hair, prominent furnishings and greatly-reduced shedding. W/w dogs have the harsh wire texture, but decreased furnishings, and overall coat length and shedding similar to non-wire animals.[52]
Animals that are homozygous for long coat (i.e., l/l) and possess at least one copy of W will have long, soft coats with furnishings, rather than wirey coats.[2]
R (curl) locus
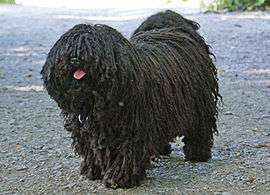
The R (curl) Locus[note 1] The alleles at the R locus (the keratin-71 gene or KRT71) determine whether an animal's coat is straight or curly.[2] There are two known alleles that occur at the R locus:
- R = Straight
- r = Curly
The relationship of R to r is one of no dominance. Heterozygotes (R/r) have wavy hair that is easily distinguishable from either homozygote. Wavy hair is considered desirable in several breeds, but because it is heterozygous, these breeds do not breed true for coat type.
Corded coats, like those of the Puli and Komondor are thought to be the result of continuously growing curly coats (long + wire + curly) with double coats, though the genetic code of corded dogs has not yet been studied. Corded coats will form naturally, but can be messy and uneven if not "groomed to cord" while the puppy's coat is lengthening.
Interaction of length and texture genes
These three genes responsible for the length and texture of an animal's coat interact to produce eight different (homozygous) phenotypes:[2]
COAT TYPE GENE INTERACTIONS[10] |
Straight R/R |
Wavy R/r |
Curly r/r | |
Non-wire w/w |
Short L/L or L/l |
Short (e.g., Akita, Greyhound) |
Short wavy (e.g., Chesapeake Bay Retriever) |
Short curly (Curly Coated Retriever? (unproven)) |
Long l/l |
Long (e.g., Pomeranian, Cocker Spaniel) |
Long wavy (e.g., Boykin Spaniel) |
Long curly (e.g., Irish Water Spaniel) | |
Wire W/W or W/w |
Long l/l |
Shaggy (e.g., Shih Tzu, Bearded Collie) |
Poofy (e.g., Bichon Frise, Portuguese Water Dog, SCWT) |
Long curly with furnishings or Corded (e.g., Poodle, Puli, Komondor) |
Short L/L or L/l |
Wire (e.g., Border Terrier, Scottish Terrier) |
Wavy wire (e.g., Wire Fox Terrier) |
Curly-wire (e.g., Wirehaired Pointing Griffon) |
Breed exceptions to coat type
Breeds in which coat type Is not explained by FgF5, RSPO2 and KRT71 genes:[10]
- Yorkshire Terrier, Silky Terrier
- Afghan Hound
Genotypes of dogs of these 3 breeds are usually L/L or L/l, which does not match with their long-haired phenotype. The Yorkshire and Silky Terriers share common ancestry and likely share an unidentified gene responsible for their long hair. The Afghan Hound has a unique patterned coat that is long with short patches on the chest, face, back and tail. The Irish Water Spaniel may share the same pattern gene, although unlike the Afghan Hound, the IWS is otherwise genetically a long-haired (fixed for l/l) breed.
Other related genes
Shedding gene
The alleles on the melanocortin 5 receptor gene (MC5R)[53] determine whether an animal will have neotenous retention of a puppy-like coat type. The locus has not been assigned a common name or letter, but has been called the shedding gene or single coat gene. There are two known alleles that occur at this locus:
- The mutant allele (reduced shedding, single coat or minimal undercoat, reduced length)
- The wildtype allele (normal shedding, double coat, normal length)
The mutant allele is incomplete dominant. With respect to coat texture, shedding, follicle density and number of secondary hairs per follicle, heterozygotes closely resemble animals homozygous for the mutant allele, with minor differences. With respect to coat length and the prominence of fringing and furnishings, the relationship between the two alleles is more complex and dependent on the alleles present at the L and W loci:
- In short-haired dogs (L/- w/w), this gene causes the smooth coat type that is common in hounds and pointers. Coat length is significantly reduced in animals homozygous for the smooth-coat allele, and of intermediate length in heterozygotes. Heterozygosity for long coat (L/l) dulls the effect on coat length. Typically, the undercoat is completely absent. Very few breeds (e.g., Chihuahua) have both smooth and non-smooth coat types.
- In long-haired dogs (l/l w/w), this gene causes fringed coats (e.g., Saluki, long-haired pointers). Coat length is reduced on the body, but lengthened on the feathering. Fringed coats may have an unbristled undercoat. An overall long single coat (e.g., Papillon, Japanese Chin) requires additional lengthening modifier genes.
- In wire-haired dogs (L/- W/-), this gene causes short-wire coats (e.g., Border Terrier, Wire-haired Dachshund, German Wirehaired Pointer) only when homozygous, and has no effect on length when heterozygous. Short-wire coats may have a bristled undercoat.
- In shaggy-haired dogs (l/l W/-), this gene causes a soft single coat (e.g., Coton de Tulear, Soft Coated Wheaten Terrier, Dachshunds of mixed longhair/wirehair parentage) which varies by breed from cottony to silky. The minimal undercoat of fringed and short-wire coats originates from a different subset of secondary hairs, and is lost when a dog has the alleles for both long and wire hair.
- In dogs with long curly coats with furnishings (l/l W/- R/R), this gene causes a single long curly coat with furnishings that will not cord (e.g., most Poodles), as proper formation of cords requires a double coat.
Remaining influences of length (e.g., setter vs Cocker Spaniel), texture (e.g., setter vs mountain dog vs spitz or Bearded Collie vs Old English Sheepdog) and abundance of undercoat (e.g., Labrador Retriever vs Keeshond) are likely polygenic.
Hairlessness gene
Some breeds of dog do not grow hair on parts of their bodies and may be referred to as "hairless". Examples of "hairless" dogs are the Xoloitzcuintli (Mexican Hairless Dog), the Peruvian Inca Orchid (Peruvian Hairless Dog) and the Chinese Crested. Research suggests that hairlessness is caused by a dominant allele of the forkhead box transcription factor (FOXI3) gene, which is homozygous lethal.[54] There are coated homozygous dogs in all hairless breeds, because this type of inheritance prevents the coat type from breeding true. The hairlessness gene permits hair growth on the head, legs and tail. Hair is sparse on the body, but present and typically enhanced by shaving, at least in the Chinese Crested, whose coat type is shaggy (long + wire). Teeth are affected as well, and hairless dogs have incomplete dentition.
The American Hairless Terrier is unrelated to the other hairless breeds and displays a different hairlessness gene. Unlike the other hairless breeds, the AHT is born fully coated, and loses its hair within a few months. The AHT gene, serum/glucocorticoid regulated kinase family member 3 gene (SGK3), is recessive and does not result in missing teeth. Because the breed is new and rare, outcrossing to the parent breed (the Rat Terrier) is permitted to increase genetic diversity. These crosses are fully coated and heterozygous for AHT-hairlessness.
Ridgeback
Some breeds (e.g., Rhodesian Ridgeback, Thai Ridgeback) have an area of hair along the spine between the withers and hips that leans in the opposite direction (cranially) to the surrounding coat. The ridge is caused by a duplication of several genes (FGF3, FGF4, FGF 19, ORAOV1 and sometimes SNP), and ridge is dominant to non-ridged.[55]
Genetic testing and phenotype prediction
In recent years genetic testing for the alleles of some genes has become available.[56] Software is also available to assist breeders in determining the likely outcome of matings.[57]
Characteristics linked to coat colour
The genes responsible for the determination of coat colour also affect other melanin-dependent development, including skin colour, eye colour, eyesight, eye formation and hearing. In most cases, eye colour is directly related to coat colour, but blue eyes in the Siberian Husky and related breeds, and copper eyes in some herding dogs are not known to be related to coat colour.
The development of coat colour, skin colour, iris colour, pigmentation in back of eye and melanin-containing cellular elements of the auditory system occur independently, as does development of each element on the left vs right side of the animal. This means that in semi-random genes (M merle, s spotting and T ticking), the expression of each element is independent. For example, skin spots on a piebald-spotted dog will not match up with the spots in the dog's coat; and a merle dog with one blue eye can just as likely have better eyesight in its blue eye than in its brown eye.
Loci for coat colour, type and length
All known genes are on separate chromosomes, and therefore no gene linkage has yet been described among coat genes. However, they do share chromosomes with other major conformational genes, and in at least one case, breeding records have shown an indication of genes passed on together.
Gene | Chromosome (in Dogs) [58][10] |
Symbol | Locus Name |
Description | Share Chr[59][60] |
---|---|---|---|---|---|
ASIP | 24 | Ay, aw, at, a | Agouti | Sable, wolf-sable, tan point, recessive black; as disproven | |
TYRP1 | 11 | B, bs, bd, bc | Brown | Black, 3 x chocolate / liver | |
SLC45A2 | 4 | C, caZ,caL | Colour | C = full color, 2 recessive alleles for types of albinism [61] | STC2, GHR(1) & GHR(2) size |
MLPH | 25 | D, d | Dilution | Black/chocolate, blue/isabella | |
MC1R | 5 | Em, Eg, E, eh, e | Extension | Black mask, grizzle, normal extension, cocker-sable, recessive red | |
PSMB7 | 9 | H, h | Harlequin | Harlequin, non-harlequin | |
DEFB103 | 16 | KB, Kbr, ky | blacK | Dominant black, brindle, fawn/sable/banded hairs | |
FgF5 | 32 | L, l | Longcoat | Short coat, long coat | |
PMEL | 10 | M, m | Merle | Double merle, merle, non-merle | HMGA2 size |
KRT71 | 27 | R, r | cuRlycoat | Straight coat, curly coat | |
MITF | 20 | S, si, sp | Spotting | Solid, Irish spotting, piebald spotting; sw not proven to exist | |
RSPO2 | 13 | W, w | Wirecoat | Wire coat, non-wire coat | |
MC5R | 1 | n/a | Shedding | Single coat/minimal shedding, double coat/regular shedding | C189G bobtail |
FOXI3 | 17 | n/a | Hairless | Hairless, coated | |
SGK3 | 29 | n/a | AHT | Coated, AHT-hairless | |
n/a | 18 | n/a | Ridgeback | Ridgeback, non-ridgeback | |
-- | 3 | - | - | No coat genes yet identified here. | IGF1R size |
-- | 7 | - | - | No coat genes yet identified here. | SMAD2 size |
-- | 15 | - | - | No coat genes yet identified here. | IGF1 size |
There are size genes on all 39 chromosomes, 17 classified as "major" genes.[62] 7 of those are identified as being of key importance and each results in ~2x difference in body weight.[63] IGF1 (Insulin-like growth factor 1), SMAD2 (Mothers against decapentaplegic homolog 2), STC2 (Stanniocalcin-2) and GHR(1) (Growth hormone receptor one) are dose-dependent with compact dwarfs vs leaner large dogs and heterozygotes of intermediate size and shape. IGF1R (Insulin-like growth factor 1 receptor) and HMGA2 (High-mobility group AT-hook 2) are incomplete dominant with delicate dwarfs vs compact large dogs and heterozygotes closer to the homozygous dwarfed phenotypes. GHR(2) (Growth hormone receptor two) is completely dominant, homozygous and heterozygous dwarfs equally small, larger dogs with a broader flatter skull and larger muzzle.[64] It is believed that the PMEL/SILV merle gene is linked to the HMGA2 size gene, meaning that alleles are most often inherited together, accounting for size differences in merle vs non-merle litter mates, such as in the Chihuahua (merles usually larger) and Shetland Sheepdog (merles frequently smaller).
See also
- Labrador Retriever coat colour genetics
- Cat coat genetics
- Equine coat color genetics
- Farm-Fox Experiment
Notes
- Researchers have not yet assigned a letter to this locus and "R" has been selected based on the use of the term "Rex" for curled hair in domestic cats.
References
- Schmutz, S. M.; Berryere, T. G. (December 2007). "Genes affecting coat color and pattern in domestic dogs: a review". Animal Genetics. 38 (6): 539–549. doi:10.1111/j.1365-2052.2007.01664.x. PMID 18052939. S2CID 28968274.
- Edouard Cadieu; Mark W. Neff; Pascale Quignon; Kari Walsh; Kevin Chase; Heidi G. Parker; Bridgett M. VonHoldt; Alison Rhue; Adam Boyko; Alexandra Byers; Aaron Wong; Dana S. Mosher; Abdel G. Elkahloun; Tyrone C. Spady; Catherine André; K. Gordon Lark; Michelle Cargill; Carlos D. Bustamante; Robert K. Wayne; Elaine A. Ostrander (October 2009). "Coat Variation in the Domestic Dog Is Governed by Variants in Three Genes". Science. 326 (5949): 150–153. Bibcode:2009Sci...326..150C. doi:10.1126/science.1177808. PMC 2897713. PMID 19713490.
- Kaelin, Christopher B.; Barsh, Gregory S. (January 2013). "Genetics of Pigmentation in Dogs and Cats". Annual Review of Animal Biosciences. 1 (1): 125–156. doi:10.1146/annurev-animal-031412-103659. ISSN 2165-8102. PMID 25387014.
- Schmutz, Sheila M.; Berryere, Tom G.; Goldfinch, Angela D. (2002-07-01). "TYRP1 and MC1R genotypes and their effects on coat color in dogs". Mammalian Genome. 13 (7): 380–387. doi:10.1007/s00335-001-2147-2. ISSN 0938-8990. PMID 12140685.
- https://http://munster.sasktelwebsite.net/DogColor/dogbrown.html
- Silver Labrador Retriever Facts And Controversy
- Ute Philipp; Henning Hamann; Lars Mecklenburg; Seiji Nishino; Emmanuel Mignot; Anne-Rose Günzel-Apel; Sheila M Schmutz; Tosso Leeb (June 2005). "Polymorphisms within the canine MLPH gene are associated with dilute coat color in dogs". BMC Genetics. 6 (34): 34. doi:10.1186/1471-2156-6-34. ISSN 1471-2156. PMC 1183202. PMID 15960853.
- Drögemüller, Cord; Philipp, Ute; Haase, Bianca; Günzel-Apel, Anne-Rose; Leeb, Tosso (2007-07-01). "A Noncoding Melanophilin Gene (MLPH) SNP at the Splice Donor of Exon 1 Represents a Candidate Causal Mutation for Coat Color Dilution in Dogs". Journal of Heredity. 98 (5): 468–473. doi:10.1093/jhered/esm021. ISSN 0022-1503. PMID 17519392.
- Welle, M.; Philipp, U.; Rüfenacht, S.; Roosje, P.; Scharfenstein, M.; Schütz, E.; Brenig, B.; Linek, M.; Mecklenburg, L.; Grest, P.; Drögemüller, M. (2009-07-01). "MLPH Genotype—Melanin Phenotype Correlation in Dilute Dogs". Journal of Heredity. 100 (suppl_1): S75–S79. doi:10.1093/jhered/esp010. ISSN 0022-1503.
- Cadieu, E.; Neff, M. W.; Quignon, P.; Walsh, K.; Chase, K.; Parker, H. G.; Vonholdt, B. M.; Rhue, A.; Boyko, A.; Byers, A.; Wong, A.; Mosher, D. S.; Elkahloun, A. G.; Spady, T. C.; André, C.; Lark, K. G.; Cargill, M.; Bustamante, C. D.; Wayne, R. K.; Ostrander, E. A. (2009). "Coat Variation in the Domestic Dog is Governed by Variants in Three Genes". Science. 326 (5949): 150–153. Bibcode:2009Sci...326..150C. doi:10.1126/science.1177808. PMC 2897713. PMID 19713490.
- Hédan B, Cadieu E, Botherel N, Dufaure de Citres C, Letko A, Rimbault M, Drogemüller C, Jagannathan V, Derrien T, Schmutz S, Leeb T and André C. Identification of a Missense Variant in MFSD12 Involved in Dilution of Phaeomelanin Leading to White or Cream Coat Color in Dogs Genes 2019, 10, 386; doi:10.3390/genes10050386
- Kaelin, Christopher B.; Barsh, Gregory S. (January 2013). "Genetics of Pigmentation in Dogs and Cats". Annual Review of Animal Biosciences. 1 (1): 125–156. doi:10.1146/annurev-animal-031412-103659. ISSN 2165-8102. PMID 25387014.
- Dreger DL, Parker H, Ostrander E, Schmutz SM. The involvement of RALY in a complex gene interaction producing the saddle tan phenotype in dogs. A presentation at Advances in Canine and Feline Genomics and Inherited Diseases 2012 Conference, Visby, Sweden. June 1, 2012.
- Dreger, Dayna L.; Schmutz, Sheila M. (2011). "A SINE Insertion Causes the Black-and-Tan and Saddle Tan Phenotypes in Domestic Dogs". Journal of Heredity. 102 (Suppl 1): S11–S18. doi:10.1093/jhered/esr042. PMID 21846741.
- Dayna L Dreger; et al. (May 29, 2019). "True Colors: commercially-acquired morphological genotypes reveal hidden allele variation among dog breeds, informing both trait ancestry and breed potential". PLOS ONE. 14 (10): e0223995. doi:10.1371/journal.pone.0223995. PMC 6816562. PMID 31658272. Retrieved September 23, 2019.
- Agouti Series
- Banded hairs on agouti wolf back
- Agouti Wolf Grey
- Julie A. Kerns; J. Newton; Tom G. Berryere; Edward M. Rubin; Jan-Fang Cheng; Sheila M. Schmutz; Gregory S. Barsh (October 2004). "Characterization of the dog Agouti gene and a nonagouti mutation in German Shepherd Dogs". Mammalian Genome. 15 (10): 798–808. doi:10.1007/s00335-004-2377-1. ISSN 0938-8990. PMID 15520882.
- Sheila Schmutz: A locus
- Sheila Schmutz: The E Locus in Dogs
- https://asas.org/docs/default-source/wcgalp-proceedings-oral/278_paper_10239_manuscript_1637_0.pdf?sfvrsn=2
- Dayna L. Dreger; Sheila M. Schmutz (Jun 2010). "A New Mutation in MC1R Explains a Coat Color Phenotype in 2 Old Breeds: Saluki and Afghan Hound". Journal of Heredity. 101 (5): 644–649. doi:10.1093/jhered/esq061. PMID 20525767.
- Sophie I. Candille; Christopher B. Kaelin; Bruce M. Cattanach; Bin Yu; Darren A. Thompson; Matthew A. Nix; Julie A. Kerns; Sheila M. Schmutz; Glenn L. Millhauser; Gregory S. Barsh (November 2007). "A β-Defensin Mutation Causes Black Coat Color in Domestic Dogs". Science. 318 (5855): 1418–1423. Bibcode:2007Sci...318.1418C. doi:10.1126/science.1147880. PMC 2906624. PMID 17947548.
- Clark, LA; Tsai, KL; Starr, AN; Nowend, KL; Murphy, KE (2011). "A missense mutation in the 20S proteasome β2 subunit of Great Danes having harlequin coat patterning". Genomics. 97 (4): 244–248. doi:10.1016/j.ygeno.2011.01.003. PMID 21256207.
- Leigh Anne Clark; Alison N. Starr; Kate L. Tsai; Keith E. Murphy (July 2008). "Genome-wide linkage scan localizes the harlequin locus in the Great Dane to chromosome 9". Gene. 418 (1–2): 49–52. doi:10.1016/j.gene.2008.04.006. PMID 18513894.
- Leigh Anne Clark; Jacquelyn M. Wahl; Christine A. Rees; Keith E. Murphy (January 2006). "Retrotransposon insertion in SILV is responsible for merle patterning of the domestic dog". PNAS. 103 (5): 1376–1381. doi:10.1073/pnas.0506940103. ISSN 0273-1134. PMC 1360527. PMID 16407134.
- "George Strain on Merle" (PDF). Merle Poms. Retrieved 27 October 2011.
- Sheila M. Schmutz; Tom G. Berryere; Dayna L. Dreger (June 2009). "MITF and White Spotting in Dogs: A Population Study". Journal of Heredity. 100 (Supplement 1): 566–574. doi:10.1093/jhered/esp029.
- Winge, Ojvind (1950). Inheritance in Dogs: With Special Reference to the Hunting Breeds. Catherine Roberts (translator). Ithaca, N.Y.: Comstock Publishing. p. 194.
- Little, Clarence Cook (1957). The Inheritance of Coat Color in Dogs. New York: Comstock Publishing. p. 194. ISBN 978-0-87605-621-9.
- Karlsson E. K.; Baranowska I.; Wade C. M.; Salmon Hillbertz N. H.; Zody M. C.; Anderson N.; Biagi T. M.; Patterson N.; Pielberg G. R.; Kulbokas E. J. III; Comstock K. E.; Keller E. T.; Mesirov J. P.; von Euler H.; Kämpe O.; Hedhammar A.; Lander E. S.; Andersson G.; Andersson L.; Lindblad-Toh K. (November 2007). "Efficient mapping of mendelian traits in dogs through genome-wide association". Nature Genetics. 39 (11): 1304–1306. doi:10.1038/ng.2007.10. PMID 17906626.
- Edward J. Cargill1, Thomas R. Famula, Robert D. Schnabel, George M. Strain & Keith E. Murphy (July 2005). The color of a Dalmatian's spots: Linkage evidence to support the TYRP1 gene. BMC Veterinary Research. 1. p. 1. doi:10.1186/1746-6148-1-1. ISBN 978-1-74661-481-2. PMC 1192828. PMID 16045797.CS1 maint: multiple names: authors list (link)
- Why white dogs are white Uppsala University 2014
- Baranowska Körberg, Izabella; Sundström, Elisabeth; Meadows, Jennifer R. S.; Rosengren Pielberg, Gerli; Gustafson, Ulla; Hedhammar, Åke; Karlsson, Elinor K.; Seddon, Jennifer; Söderberg, Arne; Vilà, Carles; Zhang, Xiaolan; Åkesson, Mikael; Lindblad-Toh, Kerstin; Andersson, Göran; Andersson, Leif (2014). "A Simple Repeat Polymorphism in the MITF-M Promoter is a Key Regulator of White Spotting in Dogs". PLOS ONE. 9 (8): e104363. Bibcode:2014PLoSO...9j4363B. doi:10.1371/journal.pone.0104363. PMC 4130573. PMID 25116146.
- Winkler, Paige A.; Gornik, Kara R.; Ramsey, David T.; Dubielzig, Richard R.; Venta, Patrick J.; Petersen-Jones, Simon M.; Bartoe, Joshua T. (2014). "A Partial Gene Deletion of SLC45A2 Causes Oculocutaneous Albinism in Doberman Pinscher Dogs". PLOS ONE. 9 (3): e92127. Bibcode:2014PLoSO...992127W. doi:10.1371/journal.pone.0092127. PMC 3960214. PMID 24647637.
- Wijesena, H.R. and Schmutz, S.M. 2015. A Missense Mutation in SLC45A2 is Associated with Albinism in Small Long Haired Dogs. Journal of Heredity 106:285-8.
- Sheila M. Schmutz (December 27, 2008). "Coat Color Alleles in Dogs". Retrieved September 12, 2010.
- http://www.doggenetics.co.uk/albino.html
- Boxer markings
- I locus - dilution of pheomelanin only
- FCI standard No 257
- Japanese Shiba Inu
- FCI Standard No 161 Beagle
- Silver Labrador Retrievers Facts And Controversy
- FCI Standard No 143 Dobermann
- Health problems linked to colour
- Gutachten zur Auslegung von § 11b des Tierschutzgesetzes (Verbot von Qualzüchtungen) page 15
- Evans, Howard E.; de Lahunta, Alexander (August 7, 2013). Miller's Anatomy of the Dog (Fourth ed.). Saunders. pp. 71–73. ISBN 978-1437708127.
- Miller's Anatomy of the Dog
- D. J. E. Housley; P. J. Venta (August 2006). "The long and the short of it: evidence that FGF5 is a major determinant of canine 'hair'-itability". Animal Genetics. 37 (4): 309–315. doi:10.1111/j.1365-2052.2006.01448.x. PMID 16879338.
- Hayward, J. J.; et al. (2016). "Complex disease and phenotype mapping in the domestic dog. '". Nat. Commun. 7 (1): 10460. doi:10.1038/ncomms10460. PMC 4735900. PMID 26795439.
- Hayward JJ et al. 2016 Complex disease and phenotype mapping in the domestic dog. Nat. Commun. 7, 10460
- Droegemueller, C; Karlsson, EK; Hytšnen, MK; Perloski, M; Dolf, G; Sainio, K; Lohi, H; Lindblad-Toh, K; Leeb, T (2008). "A mutation in hairless dogs implicates FOXI3 in ectodermal development". Science. 321 (5895): 1462. Bibcode:2008Sci...321.1462D. doi:10.1126/science.1162525. PMID 18787161.
- Salmon Hillbertz N H C, Isaksson M, Karlsson E K, Hellmen E, Rosengren Pielberg G, Savolainen P, Wad C M, von Euler E, Gustafson U, Hedhammar A, Nilsson M, Lindblad-Toh K, Andersson L, Andersson G (2007): "Duplication of FGF3, FGF4, FGF 19 and ORAOV1 causes hair ridge and predisposition to dermoid sinus in, Ridgeback dogs" in Nature Genetics, Vol. 39, Number 11, November 2007.
- "Vet Gen". Veterinary Genetic Services. 2010. Retrieved September 12, 2010.
- "Breeders Assistant". Premier Pedigree Software. 2009. Retrieved September 12, 2010.
- https://homepage.usask.ca/~schmutz/mapping.html#loci
- Hayward, J. J.; et al. (2016). "Complex disease and phenotype mapping in the domestic dog". Nat. Commun. 7 (1): 10460. doi:10.1038/ncomms10460.
- Hytönen; et al. (2008). "Ancestral T-Box mutation is present in many, but not all, short-tailed dog breeds". Journal of Heredity. 100 (2): 236–240. doi:10.1093/jhered/esn085. PMID 18854372.
- http://munster.sasktelwebsite.net/white.html
- https://www.nature.com/article-assets/npg/ncomms/2016/160122/ncomms10460/extref/ncomms10460-s1.pdf
- http://genome.cshlp.org/content/suppl/2013/10/22/gr.157339.113.DC1/Supplemental_FigureS4.pdf
- Rimbault, M; Beale, HC; Schoenebeck, JJ; Hoopes, BC; Allen, JJ; Kilroy-Glynn, P; et al. (2013). "Derived variants at six genes explain nearly half of size reduction in dog breeds". Genome Res. 23 (12): 1985–1995. doi:10.1101/gr.157339.113. PMC 3847769. PMID 24026177.
External links
- Schmutz, Sheila M. (March 4, 2010). "Dog Coat Color Genetics". Sheila Schmutz. Retrieved July 24, 2020.