Beta-lactam
A beta-lactam (β-lactam) ring is a four-membered lactam.[1] A lactam is a cyclic amide, and beta-lactams are named so because the nitrogen atom is attached to the β-carbon atom relative to the carbonyl. The simplest β-lactam possible is 2-azetidinone. β-lactams are significant structural units of medicines.[2]
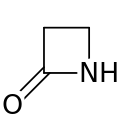
Clinical significance
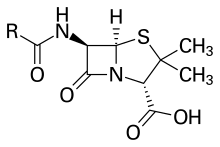
The β-lactam ring is part of the core structure of several antibiotic families, the principal ones being the penicillins, cephalosporins, carbapenems, and monobactams, which are, therefore, also called β-lactam antibiotics. Nearly all of these antibiotics work by inhibiting bacterial cell wall biosynthesis. This has a lethal effect on bacteria, although any given bacteria population will typically contain a subgroup that is resistant to β-lactam antibiotics. Bacterial resistance occurs as a result of the expression of one of many genes for the production of β-lactamases, a class of enzymes that break open the β-lactam ring. More than 1,800 different β-lactamase enzymes have been documented in various species of bacteria.[3] These enzymes vary widely in their chemical structure and catalytic efficiencies.[4] When bacterial populations have these resistant subgroups, treatment with β-lactam can result in the resistant strain becoming more prevalent and therefore more virulent. β-lactam derived antibiotics can be considered as one of the most important antibiotic classes but prone to clinical resistance. β-lactam exhibits its antibiotic properties by imitating the naturally occurring d-Ala-d-Ala substrate for the group of enzymes known as penicillin binding proteins (PBP), which have as function to cross-link the peptidoglycan part of the cell wall of the bacteria.[5]
History
The first synthetic β-lactam was prepared by Hermann Staudinger in 1907 by reaction of the Schiff base of aniline and benzaldehyde with diphenylketene[6][7] in a [2+2] cycloaddition (Ph indicates a phenyl functional group):
Up to 1970, most β-lactam research was concerned with the penicillin and cephalosporin groups, but since then, a wide variety of structures have been described.[8][9]
Synthesis and reactivity
Many methods have been developed for the synthesis of β-lactams.[10]
Breckpot synthesis: The synthesis of substituted β-lactams from the cyclization of beta amino acid esters using the Grignard reagent.[11]
Due to ring strain, β-lactams are more readily hydrolyzed than linear amides or larger lactams. This strain is further increased by fusion to a second ring, as found in most β-lactam antibiotics. This trend is due to the amide character of the β-lactam being reduced by the aplanarity of the system. The nitrogen atom of an ideal amide is sp2-hybridized due to resonance, and sp2-hybridized atoms have trigonal planar bond geometry. As a pyramidal bond geometry is forced upon the nitrogen atom by the ring strain, the resonance of the amide bond is reduced, and the carbonyl becomes more ketone-like. Nobel laureate Robert Burns Woodward described a parameter h as a measure of the height of the trigonal pyramid defined by the nitrogen (as the apex) and its three adjacent atoms. h corresponds to the strength of the β-lactam bond with lower numbers (more planar; more like ideal amides) being stronger and less reactive.[12] Monobactams have h values between 0.05 and 0.10 angstroms (Å). Cephems have h values in of 0.20–0.25 Å. Penams have values in the range 0.40–0.50 Å, while carbapenems and clavams have values of 0.50–0.60 Å, being the most reactive of the β-lactams toward hydrolysis.[13]
Other applications
A new study has suggested that β-lactams can undergo ring-opening polymerization to form amide bonds, to become nylon-3 polymers. The backbones of these polymers are identical to peptides, which offer them biofunctionality. These nylon-3 polymers can either mimic host defense peptides or act as signals to stimulate 3T3 stem cell function.[13]
Antiproliferative agents that target tubulin with β-lactams in their structure have also been reported.[14][15]
References
- Gilchrist T (1987). Heterocyclic Chemistry. Harlow: Longman Scientific. ISBN 978-0-582-01421-3.
- Fisher, J. F.; Meroueh, S. O.; Mobashery, S. (2005). "Bacterial resistance to β-lactam antibiotics: compelling opportunism, compelling opportunity". Chemical Reviews. 105 (2): 395–424. doi:10.1021/cr030102i. PMID 15700950.
- Brandt C, Braun SD, Stein C, Slickers P, Ehricht R, Pletz MW, Makarewicz O (February 2017). "In silico serine β-lactamases analysis reveals a huge potential resistome in environmental and pathogenic species". Scientific Reports. 7: 43232. Bibcode:2017NatSR...743232B. doi:10.1038/srep43232. PMC 5324141. PMID 28233789.
- Ehmann DE, Jahić H, Ross PL, Gu RF, Hu J, Kern G, Walkup GK, Fisher SL (July 2012). "Avibactam is a covalent, reversible, non-β-lactam β-lactamase inhibitor". Proceedings of the National Academy of Sciences of the United States of America. 109 (29): 11663–8. Bibcode:2012PNAS..10911663E. doi:10.1073/pnas.1205073109. PMC 3406822. PMID 22753474.
- Tipper DJ, Strominger JL (October 1965). "Mechanism of action of penicillins: a proposal based on their structural similarity to acyl-D-alanyl-D-alanine". Proceedings of the National Academy of Sciences of the United States of America. 54 (4): 1133–41. Bibcode:1965PNAS...54.1133T. doi:10.1073/pnas.54.4.1133. PMC 219812. PMID 5219821.
- Tidwell TT (2008). "Hugo (Ugo) Schiff, Schiff bases, and a century of beta-lactam synthesis". Angewandte Chemie. 47 (6): 1016–20. doi:10.1002/anie.200702965. PMID 18022986.
- Staudinger H (1907). "Zur Kenntniss der Ketene. Diphenylketen". Justus Liebigs Ann. Chem. 356 (1–2): 51–123. doi:10.1002/jlac.19073560106.
- Flynn EH (1972). Cephalosporins and Penicillins : Chemistry and Biology. New York and London: Academic Press.
- Hosseyni S, Jarrahpour A (October 2018). "Recent advances in β-lactam synthesis". Organic & Biomolecular Chemistry. 16 (38): 6840–6852. doi:10.1039/c8ob01833b. PMID 30209477.
- Alcaide, Benito; Almendros, Pedro; Aragoncillo, Cristina (2007). "Β-Lactams: Versatile Building Blocks for the Stereoselective Synthesis of Non-β-Lactam Products". Chemical Reviews. 107 (11): 4437–4492. doi:10.1021/cr0307300. PMID 17649981.
- Bogdanov B, Zdravkovski Z, Hristovski K. "Breckpot Synthesis". Institute of Chemistry Skopje.
- Woodward RB (May 1980). "Penems and related substances". Philosophical Transactions of the Royal Society of London. Series B, Biological Sciences. 289 (1036): 239–50. Bibcode:1980RSPTB.289..239W. doi:10.1098/rstb.1980.0042. PMID 6109320.
- Nangia A, Biradha K, Desiraju GR (1996). "Correlation of biological activity in β-lactam antibiotics with Woodward and Cohen structural parameters: A Cambridge database study". J. Chem. Soc. Perkin Trans. 2 (5): 943–53. doi:10.1039/p29960000943.
- O'Boyle NM, Carr M, Greene LM, Bergin O, Nathwani SM, McCabe T, Lloyd DG, Zisterer DM, Meegan MJ (December 2010). "Synthesis and evaluation of azetidinone analogues of combretastatin A-4 as tubulin targeting agents". Journal of Medicinal Chemistry. 53 (24): 8569–84. doi:10.1021/jm101115u. hdl:2262/81779. PMID 21080725.
- O'Boyle NM, Greene LM, Bergin O, Fichet JB, McCabe T, Lloyd DG, Zisterer DM, Meegan MJ (April 2011). "Synthesis, evaluation and structural studies of antiproliferative tubulin-targeting azetidin-2-ones" (PDF). Bioorganic & Medicinal Chemistry. 19 (7): 2306–25. doi:10.1016/j.bmc.2011.02.022. hdl:2262/54923. PMID 21397510.