Synthesis-dependent strand annealing
In genetics, the initial processes involved in repair of a double-strand break by synthesis-dependent strand annealing (SDSA) are identical to those in the double Holliday junction model, and have been most extensively studied in yeast species Saccharomyces cerevisiae. Following a double-stranded break, a protein complex (MRX) binds to either end of the break, working with DNA nucleases to carry out resection, resulting in 5' end digest to produce 3' overhangs of single-stranded DNA (see Figure). These overhangs are then bound to form a nucleoprotein filament, which can then locate DNA sequences similar to one of the 3' overhangs, initiating a single-stranded strand invasion into the DNA duplex containing these sequences. Once strand invasion has occurred, a displacement loop, or D-loop, is formed, at which point either SDSA or a double Holliday junction occurs.[1]
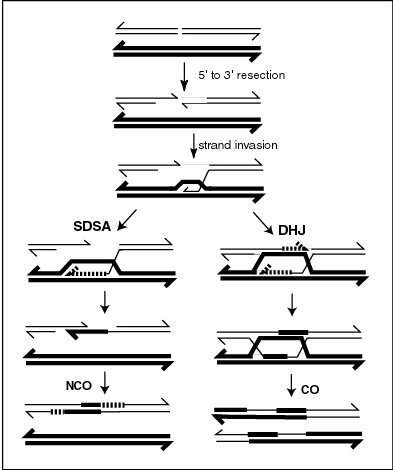
Homologous recombination via the SDSA pathway occurs in both mitotic and meiotic cells as an important mechanism of non-crossover recombination, and was first suggested as a model in 1976,[2] acquiring its current name in 1994.[3] As the double Holliday junction model was the first posited in order to explain this phenomenon,[4] various versions of the SDSA model were later proposed to explain heteroduplex DNA configurations that did not match predictions of the double Holliday junction model. Studies in S. cerevisiae found that non-crossover products appear earlier than double Holliday junctions or crossover products, which challenged the previous notion that both crossover and non-crossover products are produced by double Holliday junctions.[5]
In the SDSA model, repair of double-stranded breaks occurs without the formation of a double Holliday junction, so that the two processes of homologous recombination are identical until just after D-loop formation.[6] In yeast, the D-loop is formed by strand invasion with the help of proteins Rad51 and Rad52,[7] and is then acted on by DNA helicase Srs2 to prevent formation of the double Holliday junction in order for the SDSA pathway to occur.[8] The invading 3' strand is thus extended along the recipient homologous DNA duplex by DNA polymerase in the 5' to 3' direction, so that the D-loop physically translocates – a process referred to as bubble migration DNA synthesis.[9] The resulting single Holliday junction then slides down the DNA duplex in the same direction in a process called branch migration, displacing the extended strand from the template strand. This displaced strand pops up to form a 3' overhang in the original double-stranded break duplex, which can then anneal to the opposite end of the original break through complementary base pairing. Thus DNA synthesis fills in gaps left over from annealing, and extends both ends of the still present single stranded DNA break, ligating all remaining gaps to produce recombinant non-crossover DNA.[1]
SDSA is unique in that D-loop translocation results in conservative rather than semiconservative replication, as the first extended strand is displaced from its template strand, leaving the homologous duplex intact. Therefore, although SDSA produces non-crossover products because flanking markers of heteroduplex DNA are not exchanged, gene conversion does occur, wherein nonreciprocal genetic transfer takes place between two homologous sequences.[10]
Enzymes employed in SDSA during meiosis
Assembly of a nucleoprotein filament comprising single-stranded DNA (ssDNA) and the RecA homolog, Rad51, is a key step necessary for homology search during recombination. In the budding yeast Saccharomyces cerevisiae, Srs2 translocase dismantles Rad51 filaments during meiosis.[11] By directly interacting with Rad51, Srs2 dislodges Rad51 from nucleoprotein filaments thereby inhibiting Rad51-dependent formation of joint molecules and D-loop structures. This dismantling activity is specific for Rad51 since Srs2 does not dismantle DMC1 (a meiosis-specific Rad51 homolog), Rad52 (a Rad 51 mediator) or replication protein A (RPA, a single-stranded DNA binding protein). Srs2 promotes the non-crossover SDSA pathway, apparently by regulating RAD51 binding during strand exchange.[12]
The Sgs1(BLM) helicase is an ortholog of the human Bloom syndrome protein. It appears to be a central regulator of most of the recombination events that occur during S. cerevisiae meiosis.[13] Sgs1(BLM) may disassemble D-loop structures analogous to early strand invasion intermediates and thus promote NCO formation by SDSA.[13] The Sgs1 helicase forms a conserved complex with the topoisomerase III (Top3)-RMI1 heterodimer (that catalyzes DNA single strand passage). This complex, called STR (for its three components), promotes early formation of NCO recombinants by SDSA during meiosis.[14]
As reviewed by Uringa et al.[15] the RTEL1 helicase is required to regulate recombination during meiosis in the worm Caenorhabditis elegans. RTEL1 is a key protein in repair of DSBs. It disrupts D-loops and promotes NCO outcomes through SDSA.
The number of DSBs created during meiosis can substantially exceed the number of final CO events. In the plant Arabidopsis thaliana, only about 4% of DSBs are repaired by CO recombination,[16] suggesting that most DSBs are repaired by NCO recombination. Data based on tetrad analysis from several species of fungi show that only a minority (on average about 34%) of recombination events during meiosis are COs (see Whitehouse,[17] Tables 19 and 38 for summaries of data from S. cerevisiae, Podospora anserina, Sordaria fimicola and Sordaria brevicollis). In the fruit fly D. melanogaster during meiosis in females there is at least a 3:1 ratio of NCOs to COs.[18] These observations indicate that the majority of recombination events during meiosis are NCOs, and suggest that SDSA is the principal pathway for recombination during meiosis. The major function of SDSA during meiosis presumably is to repair DNA damages, particularly DSBs, in the genomes to be passed on to gametes (see Genetic recombination).
References
- Helleday T, Engelward BP (2007). "DNA double-strand break repair: From mechanistic understanding to cancer treatment". DNA Repair. 6 (7): 923–935. doi:10.1016/j.dnarep.2007.02.006. PMID 17363343.
- Resnick MA (1976). "Repair of double-strand breaks in DNA – model involving recombination". Journal of Theoretical Biology. 59 (1): 97–106. doi:10.1016/s0022-5193(76)80025-2. PMID 940351.
- Nassif N, Penney, Pal S, Engels WR, Gloor GB (1994). "Efficient copying of nonhomologous sequences from ectopic sites via P-element-induced gap repair". Molecular and Cellular Biology. 14 (3): 1613–25. doi:10.1128/mcb.14.3.1613. PMC 358520. PMID 8114699.
- Holliday R (1964). "The induction of mitotic recombination by mitomycin C in Ustilago and Saccharomyces". Genetics. 50 (3): 325–35. PMC 1210654. PMID 14207702.
- Allers T, Lichten M (2001). "Differential timing and control of noncrossover and crossover recombination during meiosis". Cell. 106 (1): 47–57. doi:10.1016/s0092-8674(01)00416-0. PMID 11461701.
- McMahill MS, Sham CW, Bishop DK (2007). "Synthesis-dependent strand annealing in meiosis". PLOS Biology. 5 (11): 2589–2601. doi:10.1371/journal.pbio.0050299. PMC 2062477. PMID 17988174.
- Dupaigne P, Le Breton C, Fabre F, Gangloff S, Le Carn E, Veaute X (2008). "The Srs2 helicase activity is stimulated by Rad51 filaments on dsDNA: Implications for crossover incidence during mitotic recombination". Molecular Cell. 29 (2): 243–254. doi:10.1016/j.molcel.2007.11.033. PMID 18243118.
- Miura T, Yamana Y, Usui T, Ogawa HI, Yamamoto MT, Kusano K (2012). "Homologous recombination via Synthesis-Dependent Strand Annealing in yeast requires the Irc20 and Srs2 DNA helicases". Genetics. 191 (1): 65–78. doi:10.1534/genetics.112.139105. PMC 3338270. PMID 22367032.
- Formosa T, Alberts BM (1986). "DNA-synthesis dependent on genetic-recombination – characterization of a reaction catalyzed by purified bacteriophage-T4 proteins". Cell. 47 (5): 793–806. doi:10.1016/0092-8674(86)90522-2. PMID 3022939.
- Maher RL, Branagan AM, Morrical SW (2011). "Coordination of DNA Replication and Recombination Activities in the Maintenance of Genome Stability". Journal of Cellular Biochemistry. 112 (10): 2672–2682. doi:10.1002/jcb.23211. PMC 3178728. PMID 21647941.
- Sasanuma H, Furihata Y, Shinohara M, Shinohara A (2013). "Remodeling of the Rad51 DNA strand-exchange protein by the Srs2 helicase". Genetics. 194 (4): 859–72. doi:10.1534/genetics.113.150615. PMC 3730916. PMID 23770697.
- Ira G, Malkova A, Liberi G, Foiani M, Haber JE (2003). "Srs2 and Sgs1-Top3 suppress crossovers during double-strand break repair in yeast". Cell. 115 (4): 401–11. doi:10.1016/s0092-8674(03)00886-9. PMC 4493758. PMID 14622595.
- De Muyt A, Jessop L, Kolar E, Sourirajan A, Chen J, Dayani Y, Lichten M (2012). "BLM helicase ortholog Sgs1 is a central regulator of meiotic recombination intermediate metabolism". Mol. Cell. 46 (1): 43–53. doi:10.1016/j.molcel.2012.02.020. PMC 3328772. PMID 22500736.
- Kaur H, De Muyt A, Lichten M (2015). "Top3-Rmi1 DNA single-strand decatenase is integral to the formation and resolution of meiotic recombination intermediates". Mol. Cell. 57 (4): 583–94. doi:10.1016/j.molcel.2015.01.020. PMC 4338413. PMID 25699707.
- Uringa EJ, Youds JL, Lisaingo K, Lansdorp PM, Boulton SJ (2011). "RTEL1: an essential helicase for telomere maintenance and the regulation of homologous recombination". Nucleic Acids Res. 39 (5): 1647–55. doi:10.1093/nar/gkq1045. PMC 3061057. PMID 21097466.
- Crismani W, Girard C, Froger N, Pradillo M, Santos JL, Chelysheva L, Copenhaver GP, Horlow C, Mercier R (2012). "FANCM limits meiotic crossovers". Science. 336 (6088): 1588–90. Bibcode:2012Sci...336.1588C. doi:10.1126/science.1220381. PMID 22723424.
- Whitehouse, HLK (1982). Genetic Recombination: understanding the mechanisms. Wiley. p. 321 & Table 38. ISBN 978-0471102052.
- Mehrotra S, McKim KS (2006). "Temporal analysis of meiotic DNA double-strand break formation and repair in Drosophila females". PLoS Genet. 2 (11): e200. doi:10.1371/journal.pgen.0020200. PMC 1657055. PMID 17166055.