Ruthenium anti-cancer drugs
Ruthenium anti-cancer drugs are coordination complexes of ruthenium complexes that have anticancer properties. They promise to provide alternatives to platinum-based drugs for anticancer therapy.[1][2] No ruthenium anti-cancer drug has been commercialized.
Since 1979, when Cisplatin entered clinical trials, there has been continuing interest in alternative metal-based drugs.[3] The leading ruthenium-based candidates are NAMI-A and KP1019. The first one to enter was NAMI-A. More ruthenium drugs are still under development. Ruthenium complexes as anticancer drugs are almost always designed to mimic platinum drugs, for targeting DNA.[4]
Properties of ruthenium complexes
Ruthenium has numerous properties that qualify it as an antineoplastic drug contender. While platinum-based compounds have served as very successful anti-cancer drugs, they have several limitations including their side effects, as well as ineffectiveness against certain types of cancer. Bergamo and Sava speculated in 2011 that some of these issues might be resolved with the use of a ruthenium substitute.[4] Though most ruthenium complexes are only in the beginning stages of the approval process for anti-cancer drugs, many of their properties may give them advantages over many platinum-based drugs now in use.[5]
Oxidation states and geometry
Ruthenium complexes typically adopt oxidation states II, III, and IV.[6] The geometry assumed by most ruthenium complexes is hexacoordinate, and octahedral, which differs from the square planar molecular geometry typical for platinum(II). The presence of six ligands allows for tuning of the complexes' electronic and steric properties.[1][7]
Ligand exchange rates
The rate of ligand exchange for ruthenium complexes is relatively slow in comparison with other transition metal complexes. The range of these exchange rates is around 10−2 to 10−4 s−1 which is on the scale of an average cell’s lifetime, giving the drug high kinetic stability and minimizing side reactions.[1] This allows the Ru complex to remain intact as it approaches the target as well as remain viable throughout its interaction with the cells. It is also possible through ligand variation to precisely tune the exchange kinetics, allowing a large degree of control over the complex’s stability.[8]
Activation by reduction
The theory is based on the understanding that Ru(II) complexes are generally more reactive than Ru(III) complexes. As cancer cells are generally growing and multiplying much more rapidly than normal healthy cells, this creates an environment that is less oxygen rich due to the raised metabolic rate. When this is paired with the tendency of cancerous cells to contain higher levels of glutathione and a lower pH, a chemically reducing environment is created.[1] This allows for ruthenium complexes to be administered as much less active, non-toxic Ru(III) compounds (as a prodrug), which can be activated solely at the site of the cancerous cells.[1] The reduction is thought to occur by mitochondrial proteins or microsomal single electron transfer proteins, though it may also occur by trans-membrane electron transport systems which reside outside the cell – implying that entry to the cancerous cells may not be required for the drug to be effective.[6] In theory it is also possible for the ruthenium compounds to be oxidized back to its inactive form if it leaves the cancerous environment. This phenomenon remains a theory, and while demonstrated in vitro, it has so far been difficult to prove experimentally in vivo.[4]
Transferrin transportation
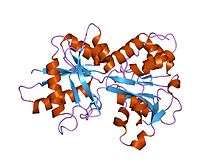
As ruthenium is in the same group as iron, they share many characteristics. Electronically, ruthenium molecules readily bond with nitrogen and sulphur donor molecules that are abundantly found in many proteins within the body.[1] For this reason ruthenium complexes are able to take advantage of the body’s ability to efficiently transport and uptake of iron. The ruthenium complexes can be transported by binding to serum albumin and transferrin proteins. These proteins allow for the efficient uptake of soluble iron for metabolic purposes.[4] As rapidly dividing cells have an increased demand for iron, the levels of transferrin receptors found on these cancerous cells are greatly increased. The receptor increase on cancerous cells has been document as two to twelve times that of healthy cells.[1] This greatly increases the selectivity of the drug as the majority of the dose is sequestered in cancerous tissues, bypassing most healthy cells. This effect contributes to the lower toxicity that is associated to the ruthenium drugs in comparison to platinum.[8] It is important to note that the ruthenium is not necessarily replacing the iron within these proteins, but that they are transported concurrently. It has been documented that transferrin-mediated ruthenium uptake is more efficient when the proteins are also saturated with iron.[1]
Current ruthenium anti-cancer drugs
NAMI
.jpg)
NAMI {Na[trans-RuCl4](DMSO)(imida)]} and NAMI-A {H2Im[trans-RuCl4(DMSO)HIm[imidH] are the most-stable ruthenium-based anti-cancer drugs.[5] NAMI-A is considered a pro-drug and is inactive at physiological pH of 7.4.[1] Cancer cells generally contain a lower oxygen concentration as well as higher levels of glutathione and a lower pH than normal tissues creating a reducing environment. Upon entering cancer cells NAMI-A is activated by the reduction of Ru(III) to Ru(II) to form the active anti-cancer agent.
KP1019
KP1019, a salt of trans-tetrachlorobis(indazole)ruthenate(III), has entered into clinical trials.[9] KP1019 has an octahedral structure with two trans N-donor indazole and four chloride ligands in the equatorial plane.[10][11][12] It has a low solubility in water, which makes it difficult to transport in the bloodstream. Instead KP1339 is used as a preparation of KP1019 in clinical trials, since it has a better solubility as a sodium salt.[12]
Proteins and other N-donors are good binding partners for KP1019.[13][14][15]
Especially transferrin and albumin are good binding partners.[16] The overall method of action for KP1019 needs to be supported further.
Tumor cells have a high requirement of iron, which results in a large concentration of transferrin. Ru(III) complexes bind to transferrin and proposed to interfere with iron uptake.[17][18]
RAPTA
RAPTA compounds are ruthenium–arene complexes bearing the 1,3,5-triaza-7-phosphatricyclo-[3.3.1.1]decane ligand.[19] The complex has a piano stool geometry. The PTA ligand confers water solubility, and the two chloride ligands are labile.[7] RAPTA compounds have low general toxicity that apparently reduces the side-effects associated with chemotherapy.[7]
RAED
Ruthenium diamine complexes are of interest as potential anticancer drugs.[20][21] RAED compounds are ruthenium–arene complexes bearing the 1,2-ethylenediamine ligand.
The ruthenium diamine complexes form adducts with guanine.[22] Methylation or substitution on en-NH, which prevent the hydrogen bonding, can lead to the loss of cytotoxic activity of the complex toward cancer cell.[23][24] The ethylenediamine ligand suppresses reactions of the complex with amino acid residues. The Ru(II) complexes have a higher affinity to DNA in the presence of protein than the Ru(III) compounds, such as NAMI-A.[25]
References
- Antonarakis, Emmanuel S.; Emadi, Ashkan (2010). "Ruthenium-based chemotherapeutics: Are they ready for prime time?". Cancer Chemotherapy and Pharmacology. 66 (1): 1–9. doi:10.1007/s00280-010-1293-1. PMC 4020437. PMID 20213076.
- Bergamo, A.; Gaiddon, C.; Schellens, J.H.M.; Beijnen, J.H.; Sava, G. (2012). "Approaching tumour therapy beyond platinum drugs". Journal of Inorganic Biochemistry. 106 (1): 90–9. doi:10.1016/j.jinorgbio.2011.09.030. PMID 22112845.
- Clarke, M.J.; Bitler, S.; Rennert, D.; Buchbinder, M.; Kelman, A.D. (1980). "Reduction and Subsequent Binding of Ruthenium Ions Catalyzed by Subcellular Components". Journal of Inorganic Biochemistry. 12 (1): 79–87. doi:10.1016/S0162-0134(00)80045-8. PMID 7373292.
- Bergamo, Alberta; Sava, Gianni (2011). "Ruthenium anticancer compounds: Myths and realities of the emerging metal-based drugs". Dalton Transactions. 40 (31): 7817–23. doi:10.1039/C0DT01816C. PMID 21629963.
- Amin, Amr; Buratovich, Michael (2009). "New Platinum and Ruthenium Complexes - the Latest Class of Potential Chemotherapeutic Drugs - a Review of Recent Developments in the Field". Mini-Reviews in Medicinal Chemistry. 9 (13): 1489–503. doi:10.2174/138955709790361566. PMID 20205631.
- Page, Simon (1 January 2012). "Ruthenium compounds as anticancer agents". Education in Chemistry. Vol. 49 no. 1. Royal Society of Chemistry. pp. 26–29.
- Ang, Wee Han; Casini, Angela; Sava, Gianni; Dyson, Paul J. (2011). "Organometallic ruthenium-based antitumor compounds with novel modes of action". Journal of Organometallic Chemistry. 696 (5): 989–98. doi:10.1016/j.jorganchem.2010.11.009.
- Bruijnincx, Pieter C.A.; Sadler, Peter J. (2009). Controlling platinum, ruthenium, and osmium reactivity for anticancer drug design. Advances in Inorganic Chemistry. 61. pp. 1–62. doi:10.1016/S0898-8838(09)00201-3. ISBN 9780123750334. PMC 3024542. PMID 21258628.
- Galanski, M.; Arion, V.; Jakupec, M.; Keppler, B. (2003). "Recent Developments in the Field of Tumor-Inhibiting Metal Complexes". Current Pharmaceutical Design. 9 (25): 2078–89. doi:10.2174/1381612033454180. PMID 14529417.
- Peti, Wolfgang; Pieper, Thomas; Sommer, Martina; Keppler, Bernhard K.; Giester, Gerald (1999). "Synthesis of Tumor-Inhibiting Complex Salts Containing the Anion trans-Tetrachlorobis(indazole)ruthenate(III) and Crystal Structure of the Tetraphenylphosphonium Salt". European Journal of Inorganic Chemistry. 1999 (9): 1551–5. doi:10.1002/(SICI)1099-0682(199909)1999:9<1551::AID-EJIC1551>3.0.CO;2-7.
- Jakupec, M. A.; Galanski, M.; Keppler, B. K. (2003). "Tumour-inhibiting platinum complexes—state of the art and future perspectives". Reviews of Physiology, Biochemistry and Pharmacology. Reviews of Physiology, Biochemistry and Pharmacology. 146. pp. 1–53. doi:10.1007/s10254-002-0001-x. ISBN 978-3-540-00228-4. PMID 12605304.
- Hartinger, Christian G.; Zorbas-Seifried, Stefanie; Jakupec, Michael A.; Kynast, Bernd; Zorbas, Haralabos; Keppler, Bernhard K. (2006). "From bench to bedside – preclinical and early clinical development of the anticancer agent indazolium trans-[tetrachlorobis(1H-indazole)ruthenate(III)] (KP1019 or FFC14A)". Journal of Inorganic Biochemistry. 100 (5–6): 891–904. doi:10.1016/j.jinorgbio.2006.02.013. PMID 16603249.
- Egger, Alexander; Arion, Vladimir B.; Reisner, Erwin; Cebrián-Losantos, Berta; Shova, Sergiu; Trettenhahn, Günter; Keppler, Bernhard K. (2005). "Reactions of Potent Antitumor Complex trans-[RuIIICl4(indazole)2]− with a DNA-Relevant Nucleobase and Thioethers: Insight into Biological Action". Inorganic Chemistry. 44 (1): 122–32. doi:10.1021/ic048967h. PMID 15627368.
- Smith, Clyde A.; Sutherland-Smith, Andrew J.; Kratz, Felix; Baker, E. N.; Keppler, B. H. (1996). "Binding of ruthenium(III) anti-tumor drugs to human lactoferrin probed by high resolution X-ray crystallographic structure analyses". Journal of Biological Inorganic Chemistry. 1 (5): 424–31. doi:10.1007/s007750050074.
- Trynda-Lemiesz, Lilianna; Karaczyn, Aldona; Keppler, Bernhard K; Kozlowski, Henryk (2000). "Studies on the interactions between human serum albumin and trans-indazolium (bisindazole) tetrachlororuthenate(III)". Journal of Inorganic Biochemistry. 78 (4): 341–6. doi:10.1016/S0162-0134(00)00062-3. PMID 10857915.
- Sulyok, M.; Hann, S.; Hartinger, C. G.; Keppler, B. K.; Stingeder, G.; Koellensperger, G. (2005). "Two dimensional separation schemes for investigation of the interaction of an anticancer ruthenium(iii) compound with plasma proteins". Journal of Analytical Atomic Spectrometry. 20 (9): 856–63. doi:10.1039/B508060F.
- Kratz, F; Hartmann, M; Keppler, B; Messori, L (1994). "The binding properties of two antitumor ruthenium(III) complexes to apotransferrin". The Journal of Biological Chemistry. 269 (4): 2581–8. PMID 8300587.
- Pongratz, Martina; Schluga, Petra; Jakupec, Michael A.; Arion, Vladimir B.; Hartinger, Christian G.; Allmaier, Günter; Keppler, Bernhard K. (2004). "Transferrin binding and transferrin-mediated cellular uptake of the ruthenium coordination compound KP1019, studied by means of AAS, ESI-MS and CD spectroscopy". Journal of Analytical Atomic Spectrometry. 19: 46–51. doi:10.1039/B309160K.
- Tan, Yu Qian; Dyson, Paul J.; Ang, Wee Han (2011). "Acetal-Functionalized RAPTA Complexes for Conjugation and Labeling". Organometallics. 30 (21): 5965–71. doi:10.1021/om200783r.
- Morris, Robert E.; Aird, Rhona E.; del Socorro Murdoch, Piedad; Chen, Haimei; Cummings, Jeff; Hughes, Nathan D.; Parsons, Simon; Parkin, Andrew; Boyd, Gary; Jodrell, Duncan I.; Sadler, Peter J. (2001). "Inhibition of Cancer Cell Growth by Ruthenium(II) Arene Complexes". Journal of Medicinal Chemistry. 44 (22): 3616–3621. doi:10.1021/jm010051m. ISSN 0022-2623.
- Chen H, Parkinson JA, Parsons S, Coxall RA, Gould RO, Sadler PJ (2002). "Organometallic ruthenium(II) diamine anticancer complexes: arene-nucleobase stacking and stereospecific hydrogen-bonding in guanine adducts". Journal of the American Chemical Society. 124 (12): 3064–82. doi:10.1021/ja017482e. PMID 11902898.CS1 maint: uses authors parameter (link)
- Chen H, Parkinson JA, Morris RE, Sadler PJ (2003). "Highly selective binding of organometallic ruthenium ethylenediamine complexes to nucleic acids: novel recognition mechanisms". Journal of the American Chemical Society. 125 (1): 173–86. doi:10.1021/ja027719m. PMID 12515520.CS1 maint: uses authors parameter (link)
- Bugarcic T, Habtemariam A, Deeth RJ, Fabbiani FP, Parsons S, Sadler PJ (2009). "Ruthenium(II) arene anticancer complexes with redox-active diamine ligands". Inorganic Chemistry. 48 (19): 9444–53. doi:10.1021/ic9013366. PMID 19780621.CS1 maint: uses authors parameter (link)
- Habtemariam A, Melchart M, Fernandez R, Parsons S, Oswald ID, Parkin A, Fabbiani FP, Davidson JE, Dawson A, Aird RE, Jodrell DI, Sadler PJ (2006). "Structure-activity relationships for cytotoxic ruthenium(II) arene complexes containing N,N-, N,O-, and O,O-chelating ligands". Journal of Medicinal Chemistry. 49 (23): 6858–68. doi:10.1021/jm060596m. PMID 17154516.CS1 maint: uses authors parameter (link)
- Wang F, Chen H, Parkinson JA, Murdoch Pdel S, Sadler PJ (2002). "Reactions of a ruthenium(II) arene antitumor complex with cysteine and methionine". Inorganic Chemistry. 41 (17): 4509–23. doi:10.1021/ic025538f. PMID 12184769.CS1 maint: uses authors parameter (link)