Heat shock response
The heat shock response (HSR) is a cellular response that increases the number of molecular chaperones to combat the negative effects on proteins caused by stressors such as increased temperatures, oxidative stress, and heavy metals.[1] In a normal cell, protein homeostasis (proteostasis) must be maintained because proteins are the main functional units of the cell.[2] Proteins take on a defined configuration in order to gain functionality. If these structures are altered, critical processes could be affected, leading to cell damage or death.[3] With the importance of proteins established, the heat shock response can be employed under stress to induce heat shock proteins (HSP), also known as molecular chaperones, that help prevent or reverse protein misfolding and provide an environment for proper folding.[4]
Protein folding is already challenging due to the crowded intracellular space where aberrant interactions can arise; it becomes more difficult when environmental stressors can denature proteins and cause even more non-native folding to occur.[5] If the work by molecular chaperones is not enough to prevent incorrect folding, the protein may be degraded by the proteasome or autophagy to remove any potentially toxic aggregates.[6] Misfolded proteins, if left unchecked, can lead to aggregation that prevents the protein from moving into its proper conformation and eventually leads to plaque formation, which may be seen in various diseases.[7] Heat shock proteins induced by the HSR can help prevent protein aggregation that can lead to common neurodegenerative diseases such as Alzheimer's, Huntington's, or Parkinson's Disease.[8]
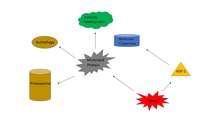
Induction of the heat shock response
With the introduction of environmental stressors, the cell must be able to maintain proteostasis. Acute or chronic subjection to these harmful conditions elicits a cytoprotective response to promote stability to the proteome.[9] HSPs (e.g. HSP70, HSP90, HSP60, etc.) are present under normal conditions but under heat stress, they are upregulated by the transcription factor heat shock factor 1 (HSF1).[10][11] There are four different transcription factors found in vertebrates (HSF 1-4) where the main regulator of HSPs is HSF1, while σ32 is the heat shock transcription factor in E. coli.[12][13] When not bound to DNA, HSF1 is in a monomeric state where it is inactive and negatively regulated by chaperones.[14] When a stress occurs, these chaperones are released due to the presence of denatured proteins and various conformational changes to HSF1 cause it to undergo nuclear localization where it becomes active through trimerization.[15][14] Newly trimerized HSF1 will bind to heat shock elements (HSE) located in promoter regions of different HSPs to activate transcription of HSP mRNA. The mRNA will eventually be transcribed and comprise the upregulated HSPs that can alleviate the stress at hand and restore proteostasis.[16] HSF1 will also regulate expression of HSPs through epigenetic modifications. The HSR will eventually attenuate as HSF1 returns to its monomeric form, negatively regulated through association with HSP70 and HSP90 along with additional post-translational modifications.[17] The HSR is not only involved with increasing transcription levels of HSPs; other facets include stress-induced mRNA stability preventing errors in mRNA and enhanced control during translation to thwart misfolding.[18]
Molecular chaperones
Molecular chaperones are typically referred to as proteins that associate with and help other proteins reach a native conformation while not being present in the end state.[19] Chaperones bind to their substrate (i.e. a misfolded protein) in an ATP-dependent manner to perform a specific function.[20] Exposed hydrophobic residues are a major problem with regards to protein aggregation because they can interact with one another and form hydrophobic interactions.[21] It is the job of chaperones to prevent this aggregation by binding to the residues or providing proteins a “safe” environment to fold properly.[22] Heat shock proteins are also believed to play a role in the presentation of pieces of proteins (or peptides) on the cell surface to help the immune system recognize diseased cells.[23] The major HSPs involved in the HSR include HSP70, HSP90, and HSP60.[5] Chaperones include the HSP70s and HSP90s while HSP60s are considered to be chaperonins.[18]
The HSP70 chaperone family is the main HSP system within cells, playing a key role in translation, post-translation, prevention of aggregates and refolding of aggregated proteins.[24] When a nascent protein is being translated, HSP70 is able to associate with the hydrophobic regions of the protein to prevent faulty interactions until translation is complete.[25] Post-translational protein folding occurs in a cycle where the protein becomes bound/released from the chaperone allowing burying hydrophobic groups and aiding in overcoming the energy needed to fold in a timely fashion.[26] HSP70 plays a part in de-aggregating proteins using the aforementioned mechanism; the chaperone will bind to exposed hydrophobic residues and either partially or fully disassemble the protein, allowing HSP70 to assist in the proper refolding.[27] When proteins are beyond the point of refolding, HSP70s can help direct these potentially toxic aggregates to be degraded by the proteasome or through autophagy.[28] HSP90s are parallel to HSP70s with respect to the refolding or proteins and use in protein clearance.[4] One difference between the two HSPs is HSP90s ability to keep proteins in an unfolded yet stable configuration until a signal causes the protein to translocate and complete its folding.[25]
Sometimes, HSP70 is unable to effectively aid a protein in reaching its final 3-D structure; The main reason being the thermodynamic barriers for folding are too high for the chaperone to meet.[24] Because the intracellular space is very crowded, sometimes proteins need an isolated space to prevent aberrant interactions between other proteins, which is provided by chaperonins or HSP60s .[7] HSP60s are barrel shaped and suited to bind to the hydrophobic residues of proteins.[29] Once a cap binds to the chaperonin, the protein is free within the barrel to undergo hydrophobic collapse and reach a stable conformation.[30] Once the cap is removed, the protein can either be correctly folded and move on to perform its function or return to a HSP if it is still not folded accurately.[31] These chaperones function to remove aggregation and significantly speed up protein folding.[21]
References
- Morimoto RI (March 1993). "Cells in stress: transcriptional activation of heat shock genes". Science. 259 (5100): 1409–10. doi:10.1126/science.8451637. PMID 8451637.
- Balchin D, Hayer-Hartl M, Hartl FU (July 2016). "In vivo aspects of protein folding and quality control". Science. 353 (6294): aac4354. doi:10.1126/science.aac4354. hdl:11858/00-001M-0000-002B-0856-C. PMID 27365453.
- Richter K, Haslbeck M, Buchner J (October 2010). "The heat shock response: life on the verge of death". Molecular Cell. 40 (2): 253–66. doi:10.1016/j.molcel.2010.10.006. PMID 20965420.
- Weibezahn J, Schlieker C, Tessarz P, Mogk A, Bukau B (August 2005). "Novel insights into the mechanism of chaperone-assisted protein disaggregation". Biological Chemistry. 386 (8): 739–44. doi:10.1515/BC.2005.086. PMID 16201868.
- Fink AL (April 1999). "Chaperone-mediated protein folding". Physiological Reviews. 79 (2): 425–49. doi:10.1152/physrev.1999.79.2.425. PMID 10221986.
- Cuervo AM, Wong E (January 2014). "Chaperone-mediated autophagy: roles in disease and aging". Cell Research. 24 (1): 92–104. doi:10.1038/cr.2013.153. PMC 3879702. PMID 24281265.
- Tower J (July 2009). "Hsps and aging". Trends in Endocrinology and Metabolism. 20 (5): 216–22. doi:10.1016/j.tem.2008.12.005. PMC 3835556. PMID 19394247.
- Wyttenbach A, Arrigo AP (2013). The Role of Heat Shock Proteins during Neurodegeneration in Alzheimer's, Parkinson's and Huntington's Disease. Landes Bioscience.
- Kaushik S, Cuervo AM (December 2015). "Proteostasis and aging". Nature Medicine. 21 (12): 1406–15. doi:10.1038/nm.4001. PMID 26646497.
- Abravaya K, Myers MP, Murphy SP, Morimoto RI (July 1992). "The human heat shock protein hsp70 interacts with HSF, the transcription factor that regulates heat shock gene expression". Genes & Development. 6 (7): 1153–64. doi:10.1101/gad.6.7.1153. PMID 1628823.
- Morimoto RI, Kline MP, Bimston DN, Cotto JJ (1997). "The heat-shock response: regulation and function of heat-shock proteins and molecular chaperones". Essays in Biochemistry. 32: 17–29. PMID 9493008.
- Akerfelt M, Trouillet D, Mezger V, Sistonen L (October 2007). "Heat shock factors at a crossroad between stress and development". Annals of the New York Academy of Sciences. 1113: 15–27. doi:10.1196/annals.1391.005. PMID 17483205.
- Guisbert E, Yura T, Rhodius VA, Gross CA (September 2008). "Convergence of molecular, modeling, and systems approaches for an understanding of the Escherichia coli heat shock response". Microbiology and Molecular Biology Reviews. 72 (3): 545–54. doi:10.1128/MMBR.00007-08. PMC 2546862. PMID 18772288.
- Morley JF, Morimoto RI (February 2004). "Regulation of longevity in Caenorhabditis elegans by heat shock factor and molecular chaperones". Molecular Biology of the Cell. 15 (2): 657–64. doi:10.1091/mbc.e03-07-0532. PMC 329286. PMID 14668486.
- Barna J, Csermely P, Vellai T (August 2018). "Roles of heat shock factor 1 beyond the heat shock response". Cellular and Molecular Life Sciences. 75 (16): 2897–2916. doi:10.1007/s00018-018-2836-6. PMID 29774376.
- Akerfelt M, Trouillet D, Mezger V, Sistonen L (October 2007). "Heat shock factors at a crossroad between stress and development". Annals of the New York Academy of Sciences. 1113: 15–27. doi:10.1196/annals.1391.005. PMID 17483205.
- Trinklein ND, Murray JI, Hartman SJ, Botstein D, Myers RM (March 2004). "The role of heat shock transcription factor 1 in the genome-wide regulation of the mammalian heat shock response". Molecular Biology of the Cell. 15 (3): 1254–61. doi:10.1091/mbc.e03-10-0738. PMC 363119. PMID 14668476.
- Taipale M, Tucker G, Peng J, Krykbaeva I, Lin ZY, Larsen B, Choi H, Berger B, Gingras AC, Lindquist S (July 2014). "A quantitative chaperone interaction network reveals the architecture of cellular protein homeostasis pathways". Cell. 158 (2): 434–448. doi:10.1016/j.cell.2014.05.039. PMC 4104544. PMID 25036637.
- Lindquist S, Craig EA (1988). "The heat-shock proteins". Annual Review of Genetics. 22: 631–77. doi:10.1146/annurev.ge.22.120188.003215. PMID 2853609.
- Priya S, Sharma SK, Goloubinoff P (June 2013). "Molecular chaperones as enzymes that catalytically unfold misfolded polypeptides". FEBS Letters. 587 (13): 1981–7. doi:10.1016/j.febslet.2013.05.014. PMID 23684649.
- Vabulas RM, Raychaudhuri S, Hayer-Hartl M, Hartl FU (December 2010). "Protein folding in the cytoplasm and the heat shock response". Cold Spring Harbor Perspectives in Biology. 2 (12): a004390. doi:10.1101/cshperspect.a004390. PMC 2982175. PMID 21123396.
- Naylor DJ, Hartl FU (2001). "Contribution of molecular chaperones to protein folding in the cytoplasm of prokaryotic and eukaryotic cells". Biochemical Society Symposium. 68 (68): 45–68. doi:10.1042/bss0680045. PMID 11573347.
- Tsan MF, Gao B (June 2009). "Heat shock proteins and immune system". Journal of Leukocyte Biology. 85 (6): 905–10. doi:10.1189/jlb.0109005. PMID 19276179.
- Hartl FU, Bracher A, Hayer-Hartl M (July 2011). "Molecular chaperones in protein folding and proteostasis". Nature. 475 (7356): 324–32. doi:10.1038/nature10317. PMID 21776078.
- Lackie RE, Maciejewski A, Ostapchenko VG, Marques-Lopes J, Choy WY, Duennwald ML, Prado VF, Prado MA (2017). "The Hsp70/Hsp90 Chaperone Machinery in Neurodegenerative Diseases". Frontiers in Neuroscience. 11: 254. doi:10.3389/fnins.2017.00254. PMC 5433227. PMID 28559789.
- Mayer MP, Bukau B (March 2005). "Hsp70 chaperones: cellular functions and molecular mechanism". Cellular and Molecular Life Sciences. 62 (6): 670–84. doi:10.1007/s00018-004-4464-6. PMC 2773841. PMID 15770419.
- Calderwood SK, Murshid A, Prince T (2009). "The shock of aging: molecular chaperones and the heat shock response in longevity and aging--a mini-review". Gerontology. 55 (5): 550–8. doi:10.1159/000225957. PMC 2754743. PMID 19546513.
- Dokladny K, Myers OB, Moseley PL (2015). "Heat shock response and autophagy--cooperation and control". Autophagy. 11 (2): 200–13. doi:10.1080/15548627.2015.1009776. PMC 4502786. PMID 25714619.
- Apetri AC, Horwich AL (November 2008). "Chaperonin chamber accelerates protein folding through passive action of preventing aggregation". Proceedings of the National Academy of Sciences of the United States of America. 105 (45): 17351–5. doi:10.1073/pnas.0809794105. PMC 2579888. PMID 18987317.
- Kmiecik S, Kolinski A (July 2011). "Simulation of chaperonin effect on protein folding: a shift from nucleation-condensation to framework mechanism". Journal of the American Chemical Society. 133 (26): 10283–9. doi:10.1021/ja203275f. PMC 3132998. PMID 21618995.
- Todd MJ, Lorimer GH, Thirumalai D (April 1996). "Chaperonin-facilitated protein folding: optimization of rate and yield by an iterative annealing mechanism". Proceedings of the National Academy of Sciences of the United States of America. 93 (9): 4030–5. doi:10.1073/pnas.93.9.4030. PMC 39481. PMID 8633011.