Dual systems model
The dual systems model, also known as the maturational imbalance model,[1] is a theory arising from developmental cognitive neuroscience which posits that increased risk-taking during adolescence is a result of a combination of heightened reward sensitivity and immature impulse control.[2][3] In other words, the appreciation for the benefits arising from the success of an endeavor is heightened, but the appreciation of the risks of failure lags behind.
The dual systems model hypothesizes that early maturation of the socioemotional system (including brain regions like the striatum) increases adolescents' attraction for exciting, pleasurable, and novel activities during a time when cognitive control systems (including brain regions like the prefrontal cortex) are not fully developed and thus cannot regulate these appetitive, and potentially hazardous, impulses. The temporal gap in the development of the socioemotional and cognitive control systems creates a period of heightened vulnerability to risk-taking during mid-adolescence. In the dual systems model, "reward sensitivity" and "cognitive control" refer to neurobiological constructs that are measured in studies of brain structure and function. Other models similar to the dual systems model are the maturational imbalance model,[4] the driven dual systems model,[5] and the triadic model.[6]
Historical perspective
The dual systems model arose out of evidence from developmental cognitive neuroscience providing insight into how patterns of brain development could explain aspects of adolescent decision-making. In 2008, Laurence Steinberg's laboratory at Temple University and BJ Casey's laboratory at Cornell separately proposed similar dual systems theories of adolescent risky decision-making.[4][7][8] Casey et al. termed their model the maturational imbalance model.
Models
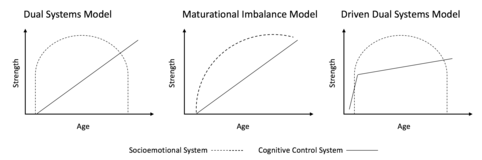
Maturational imbalance model
Both the dual systems model and the maturational imbalance model conceive of a slower developing cognitive control system that matures through late adolescence. The dual systems model proposes an inverted-U shape development of the socioemotional system, such that reward responsivity increases in early adolescence and declines thereafter. The maturational imbalance model portrays a socioemotional system that reaches its peak around mid-adolescence and then plateaus into adulthood. Further, the dual systems model proposes that the development of the cognitive control and socioemotional systems is independent whereas the maturational imbalance proposes that the maturation of the cognitive control system leads to dampening of socioemotional responsivity.[1][2]
Driven dual systems model
Recently, another variation of the dual systems model was proposed called the "driven dual systems model".[5] This model proposes an inverted-U shaped trajectory of socioemotional system responsivity, similar to the dual systems model, but hypothesizes a cognitive control trajectory that plateaus in mid-adolescence. This cognitive control trajectory differs from that proposed by the dual systems model and maturational imbalance model which continues to increase into the early 20s. Similar to the driven dual systems model, a model has been proposed including a hyperactive socioemotional system that undermines the regulatory ability of the cognitive control system.[9] These later models hypothesize that cognitive control development is completed by mid-adolescence and attribute increased risk-taking during adolescence to the hyperarousal of the socioemotional system. The dual systems model and maturational imbalance model propose that cognitive control development continues into early adulthood and that increased risk-taking in adolescence is attributable to a developmental imbalance where the socioemotional system is at its peak of development but the cognitive control system developmental trajectory lags behind.[10]
Triadic model
The "triadic model", which includes a third brain system responsible for emotion processing and primarily implicating the amygdala.[11] The triadic model proposes that this emotion system increases impulsivity during adolescence by increasing the perceived cost of delaying decision-making. This model posits that impulsivity and risk seeking in adolescence is due to a combination of hyperactive reward systems causing adolescents to approach appetitive stimuli, emotion processing systems causing adolescents to enhance perceived costs of delaying behaviors and reduce avoidance of potentially negative stimuli, and an underdeveloped cognitive control system that is unable to regulate reward-seeking behaviors.[11]
Adolescent risk-taking
Risk-taking in certain, but not all, domains peaks during adolescence. Most notably, mortality and morbidity rates increase significantly from childhood to adolescence[12][13] despite the fact that physical and mental capabilities increase during this period. The primary cause for this increase in mortality/morbidity among adolescents is preventable injury. According to the Center for Disease Control, in 2014 about 40% of all adolescent deaths (ages 15–19 years) were caused by unintentional accidents.[14] From 1999 to 2006, almost one-half of all adolescent deaths (ages 12–19 years) were due to accidental injury.[15] Of these unintentional injuries, about 2/3 are due to motor vehicle accidents, followed by unintentional poisoning, unintentional drowning, other land transportation accidents, and unintentional discharge of firearms.[15]
The dual systems model proposes that mid-adolescence is the time of highest biological propensity for risk-taking, but that older adolescents may exhibit higher levels of real-world risk-taking (e.g., binge drinking is most common during the early 20s)[16][17] not due to greater propensity for risk-taking but due to greater opportunity.[10] For example, individuals in their early 20s compared to mid-adolescence have less adult supervision, greater financial resources, and greater legal privileges. The dual systems model looks to experimental paradigms in developmental neuroscience for evidence of this greater biological propensity for risk-taking.[2]
There is also a consistent relation between age and crime with adolescents and young adults being more likely to engage in violent and non-violent crime.[18] These findings are linked to increases in sensation-seeking, which is the tendency to seek out novel, exciting, and rewarding stimuli, during adolescence, and continued development of impulse control, which is the ability to regulate one's behavior. The dual systems model points to brain development as a mechanism for this association.[2]
Reward seeking
Across many species including humans, rodents, and nonhuman primates, adolescents demonstrate peaks in reward-seeking behaviors.[19][2] For example, adolescent rats are more sensitive than adult rats to rewarding stimuli[20] and show enhanced behavioral responses to novelty and peers.[21][22] Adolescent humans show peaks in self-reported sensation-seeking,[23] increased neural activation to monetary and social rewards,[24][25] greater temporal discounting of delayed rewards,[26] and heightened preferences for primary rewards (e.g., sweet substances).[27]
Sensation-seeking is a type of reward seeking involving the tendency to seek out novel, exciting, and rewarding stimuli. Sensation-seeking has been found to increase in preadolescence, peak in mid-adolescence, and decline in early adulthood.[28]
Impulsivity
Impulsivity has been found to exhibit a different developmental trajectory than reward or sensation seeking.[28] Impulsivity gradually declines with age in a linear fashion.[29] Around mid-adolescence when impulsivity and sensation-seeking are at their peak is the theoretical peak age for risk-taking according to the dual systems model.[2]
Social influence
Adolescent risk-taking is more likely to occur in the presence of peers compared to adults.[16][30] Animal studies have found that adolescent mice, but not adult mice, consume more alcohol in the presence of peers than when alone.[31] In humans, the presence of peers has been found to result in increased activation in the striatum and orbitofrontal cortex risk-taking, and activation in these regions predicted subsequent risk-taking among adolescents but not adults.[32] Age differences in activation of the striatum and frontal cortex have been interpreted to suggest heightened risk-taking in the presence of peers is due to the influence of peers on reward processing rather than the influence of peers on cognitive control.[32]
Socioemotional system
The term socioemotional brain network or system (also known as the ventral affective system) refers to the striatum as well as the medial and orbital prefrontal cortices.[33]
Dopamine
Evidence from rodent studies indicates the dopaminergic system, the pathway connecting the ventral tegmental area to the nucleus accumbens and olfactory tubercle, plays a critical role in the brain's reward circuitry and the dopamine-rich striatum has been implicated as a key contributor to reward sensitivity in the brain.[34][35]
During puberty, the dopaminergic system undergoes significant reorganization.[36] Increased dopamine projections from mesolimbic areas (e.g., the striatum) to the prefrontal cortex[37][38] have been observed during mid- and late-adolescence. These projections are pruned/decline in early adulthood.[39] Adolescent-specific peaks in dopamine receptors in the striatum have been observed in humans and rodents.[40] Additionally, dopamine concentrations projecting to the prefrontal cortex increase into adolescence as do the dopamine projections from the prefrontal cortex to the striatum (namely the nucleus accumbens).[41]
Hyper- versus hypo-sensitivity to reward
The striatum has been linked to reward processing, learning, and motivation.[47]
Hyperactivity
Neuroimaging studies using functional magnetic resonance imaging (fMRI) have shown that the ventral striatum is more active among adolescents compared to children and adults when receiving monetary rewards,[43][48] primary rewards,[27] and social rewards.[32][49] Peaks in striatal activity as associated with increased self-reported risk-taking.[29]
Hypoactivity
Some studies have found that striatum activity is blunted compared to children and adults when anticipating rewards,[50] which has been linked to greater risk-taking behaviors.[51] The theory linking this hypoactivation to greater risk-taking is that adolescents experience less gratifying experience from anticipating rewards and they are therefore motivated to seek out more reward-inducing experiences to achieve the same level of reward sensation as other age groups.[36]
Current consensus
Although evidence exists for both adolescent hyper-responsiveness to rewards and hypo-responsiveness to rewards, the field of developmental neuroscience has generally converged on the view of hyper-responsiveness.[52] In other words, that is, that adolescents are motivated, in part, to engage in greater reward-seeking behaviors because of developmental changes in the striatum that contribute to hypersensitivity to reward.[6]
Cognitive control system
The cognitive control system refers to the lateral prefrontal, lateral parietal, and anterior cingulate cortices. The most commonly investigated region is the prefrontal cortex which undergoes substantial development throughout adolescence.[43] The development of the prefrontal cortex has been implicated in the ability to regulate behavior and engage in inhibitory control.[53]
As a result of synaptic pruning and myelination of the prefrontal cortex, improvements in executive functions have been observed during adolescence.[53]
Synaptic pruning
During development, the brain undergoes overproduction of neurons and their synaptic connections and then prunes those that are unnecessary for optimal functioning.[54] This developmental process results in grey matter reduction over development. During adolescence, this pruning process is specialized with some areas losing approximately half of their synaptic connections but others showing little change.[55] Total grey matter volume undergoes substantial pruning starting around puberty. The process of grey matter loss (i.e., maturation) occurs differentially in different brain regions with frontal and occipital poles losing grey matter early, but the prefrontal cortex losing grey matter only at the end of adolescence.[55]
Myelination
In addition to synaptic pruning, the brain undergoes myelination, which influences the speed of information flow across brain regions. Myelination involves neuronal axons connecting certain brain areas to become insulated with a white, fatty substance called myelin that increases the speed and efficiency of transmission along axons. Myelination increases dramatically during adolescence.[56] Myelination contributes to the developmental thinning or reduction in grey matter in the prefrontal cortex during adolescence.[57]
Links to inhibitory control
Evidence supporting the dual systems model theory of delayed maturation of the cognitive control system is supported by evidence of structural changes like cortical thinning[55] as well as less diffuse activation of frontal regions during inhibitory control tasks from adolescence to adulthood.[58][59] Regardless of age, increased activation of the prefrontal cortex is related to better performance on response inhibition tasks.[60]
Experimental paradigms
Reward tasks
Three primary experimental paradigms are used to study reward behavior in adolescents (1) passive receipt of reward, (2) reward conditional on task performance, and (3) decision-making selecting different types of reward options.
Passive exposure tasks
Passive exposure tasks generally involve exposing the participant to pleasant stimuli (e.g., monetary reward, attractive faces). These paradigms also involve exposure to negative stimuli for the purposes of comparison (e.g., monetary loss, angry faces). Although these tasks are more commonly used to investigate emotion processing rather than reward, some studies have used a slot-machine passive task[61] to target reward circuitry in the brain. Faces have also been used as reward for motivational paradigms.[62] Passive exposure tasks have been found to activate the striatum and orbitofrontal cortex, with striatal activation greater in adolescents in response to rewarding stimuli but orbitofrontal activation greater in adults in response to negative stimuli.[61]
Performance contingent tasks
Reward tied to task performance typically involves participants being asked to complete a task in order to obtain a reward (and sometimes to avoid losing a reward). Task performance is not necessarily directly related to the reward. Examples of this type of task are the Pirate's paradigm,[43] monetary incentive delay (MID) task,[63] Iowa Gambling Task,[64] Balloon Analogue Risk Task (BART),[65] and Columbia Card Task,[66] among others. Differences in activation to anticipation of reward versus preparation to try and achieve reward have been reported on performance related reward tasks.[44][50]
Decision-making tasks
Reward decision-making tasks involve participants being asked to choose among different options of reward. Sometimes the rewards differ on probability, magnitude, or type of reward (e.g., social versus monetary). These tasks are typically conceived to not have a correct or incorrect response, but rather to have decision-making based on the participants' preference. Examples of decision making tasks include delay discounting tasks[67] and the Driving Game.[32] During feedback on decision-making tasks, greater striatal activation to rewarding outcomes has been observed in adolescents compared to adults.[25][61]
Response inhibition tasks
Common response inhibition tasks are the Go/No-Go, Flanker, Stroop, Stop Signal, and anti-saccade tasks. Individuals who perform well on these tasks generally activate the prefrontal cortex to a greater extent than individuals who perform poorly on these tasks.[59][68][69] Performance on these tasks improves with age.
Go/No-Go task
The Go/No-Go task requires participants to respond, usually by pressing a button or a key on a computer keyboard, to a designated cue or withhold a response, by not pressing the button/key, to a different designated cue. Variants of this task include alphabet letters, shapes, and faces.[70][71]
Flanker task
The Flanker task typically involves presentation of a target flanked by non-target stimuli that is either in the same direction as the target (congruent) or in the opposite direction of a target (incongruent) or neither direction (neutral). Participants have to respond to the direction of the target ignoring the non-target stimuli.[72]
Stroop tasks
Stroop tasks require participants to respond to one facet of the presented stimuli (e.g., read the word) but ignore another competing facet (e.g., ignore a contradictory color).[73]
Stop signal task
The Stop Signal task is similar to the Go/No-Go task in that participants see a cue indicating a go trial. For stop trials, participants see the go cue but then are presented with the stop signal (typically a sound) indicating they should not respond to the go trial. Presenting the stop signal after the go cue makes this task more difficult than traditional Go/No-Go tasks.[74]
Anti-saccade task
Anti-saccade tasks typically require participants to fixate on a motionless target. A stimulus is then presented on one side of the target and the participant is asked to make a saccade (either move their eyes or respond with a button press) in the direction away from the stimulus.[75]
Legal relevance
Adolescent developmental immaturity and culpability were central to three US Supreme Court cases: Roper v. Simmons,[76] Graham v. Florida,[77] and Miller v. Alabama.[78] Prior to Roper in 2005, the Supreme Court had relied on common sense standards to determine adolescent culpability. For example, in Thompson v. Oklahoma,[79] the Court prohibited capital punishment for individuals under the age of 16 stating that "Contemporary standards of decency confirm our judgment that such a young person is not capable of acting with the degree of culpability that can justify the ultimate penalty."[79] In Roper, however, the Court looked to developmental science as rationale for abolishing capital punishment for juveniles. In 2010, the Court ruled life without parole was unconstitutional for juveniles in Graham and in 2012 the Court ruled that States could not mandate life without parole for juveniles even in the case of homicide in Miller. In Miller, the Court stated "It is increasingly clear that adolescent brains are not yet fully mature in regions and systems related to higher-order executive functions such as impulse control, planning ahead, and risk avoidance."[78]
Criticism
Others have criticized the triadic model for a lack of evidence implicating the emotion processing systems (e.g., the amygdala) in risk-taking during adolescence.[10]
References
- Casey, B. J.; Jones, Rebecca M.; Somerville, Leah H. (2011-03-01). "Braking and Accelerating of the Adolescent Brain". Journal of Research on Adolescence. 21 (1): 21–33. doi:10.1111/j.1532-7795.2010.00712.x. ISSN 1532-7795. PMC 3070306. PMID 21475613.
- Steinberg, Laurence (2010-04-01). "A dual systems model of adolescent risk-taking". Developmental Psychobiology. 52 (3): 216–224. doi:10.1002/dev.20445. ISSN 1098-2302. PMID 20213754.
- Somerville, Leah H.; Jones, Rebecca M.; Casey, B.J. (2009). "A time of change: Behavioral and neural correlates of adolescent sensitivity to appetitive and aversive environmental cues". Brain and Cognition. 72 (1): 124–133. doi:10.1016/j.bandc.2009.07.003. PMC 2814936. PMID 19695759.
- Casey, B.j.; Jones, Rebecca M.; Hare, Todd A. (2008-03-01). "The Adolescent Brain". Annals of the New York Academy of Sciences. 1124 (1): 111–126. Bibcode:2008NYASA1124..111C. doi:10.1196/annals.1440.010. ISSN 1749-6632. PMC 2475802. PMID 18400927.
- Luna, Beatriz; Wright, Catherine (2016). APA handbook of psychology and juvenile justice. pp. 91–116. doi:10.1037/14643-005. ISBN 978-1-4338-1967-4.
- Ernst, Monique (2014). "The triadic model perspective for the study of adolescent motivated behavior". Brain and Cognition. 89: 104–111. doi:10.1016/j.bandc.2014.01.006. PMC 4248307. PMID 24556507.
- Steinberg, Laurence (2008). "A Social Neuroscience Perspective on Adolescent Risk-Taking". Developmental Review : DR. 28 (1): 78–106. doi:10.1016/j.dr.2007.08.002. ISSN 0273-2297. PMC 2396566. PMID 18509515.
- Steinberg, Laurence; Albert, Dustin; Cauffman, Elizabeth; Banich, Marie; Graham, Sandra; Woolard, Jennifer (2008). "Age differences in sensation seeking and impulsivity as indexed by behavior and self-report: Evidence for a dual systems model". Developmental Psychology. 44 (6): 1764–1778. doi:10.1037/a0012955. PMID 18999337.
- Luciana, Monica; Collins, Paul F. (2012-12-01). "Incentive Motivation, Cognitive Control, and the Adolescent Brain: Is It Time for a Paradigm Shift?". Child Development Perspectives. 6 (4): 392–399. doi:10.1111/j.1750-8606.2012.00252.x. ISSN 1750-8592. PMC 3607661. PMID 23543860.
- Shulman, Elizabeth P.; Smith, Ashley R.; Silva, Karol; Icenogle, Grace; Duell, Natasha; Chein, Jason; Steinberg, Laurence (2016). "The dual systems model: Review, reappraisal, and reaffirmation". Developmental Cognitive Neuroscience. 17: 103–117. doi:10.1016/j.dcn.2015.12.010. PMID 26774291.
- ERNST, MONIQUE; PINE, DANIEL S.; HARDIN, MICHAEL (2006). "Triadic model of the neurobiology of motivated behavior in adolescence". Psychological Medicine. 36 (3): 299–312. doi:10.1017/S0033291705005891. ISSN 0033-2917. PMC 2733162. PMID 16472412.
- Dahl, Ronald E. (June 2004). "Adolescent brain development: a period of vulnerabilities and opportunities. Keynote address". Annals of the New York Academy of Sciences. 1021 (1): 1–22. Bibcode:2004NYASA1021....1D. doi:10.1196/annals.1308.001. ISSN 0077-8923. PMID 15251869.
- Casey, B. J.; Caudle, Kristina (2013-04-16). "The Teenage Brain". Current Directions in Psychological Science. 22 (2): 82–87. doi:10.1177/0963721413480170. PMC 4182916. PMID 25284961.
- Centers for Disease Control and Prevention (June 30, 2016). "Deaths: Leading Causes for 2014" (PDF). www.cdc.gov.
- Centers for Disease Control and Prevention (May 2010). "Mortality Among Teenagers Aged 12-19 Years: United States, 1999-2006". www.cdc.gov.
- Chassin, Laurie; Hussong, Andrea; Beltran, Iris (2009). Handbook of Adolescent Psychology. John Wiley & Sons, Inc. doi:10.1002/9780470479193.adlpsy001022. ISBN 9780470479193.
- Willoughby, Teena; Good, Marie; Adachi, Paul J.C.; Hamza, Chloe; Tavernier, Royette (2013). "Examining the link between adolescent brain development and risk taking from a social–developmental perspective". Brain and Cognition. 83 (3): 315–323. doi:10.1016/j.bandc.2013.09.008. PMID 24128659.
- Steinberg, L (2013). "The influence of neuroscience on US Supreme Court decisions about adolescents' criminal culpability". Nat. Rev. Neurosci. 14 (7): 513–518. doi:10.1038/nrn3509. PMID 23756633.
- Spear, Linda Patia (2011-10-01). "Rewards, aversions and affect in adolescence: Emerging convergences across laboratory animal and human data". Developmental Cognitive Neuroscience. 1 (4): 390–403. doi:10.1016/j.dcn.2011.08.001. ISSN 1878-9293. PMC 3170768. PMID 21918675.
- Simon, Nicholas W.; Moghaddam, Bita (2015). "Neural processing of reward in adolescent rodents". Developmental Cognitive Neuroscience. 11: 145–154. doi:10.1016/j.dcn.2014.11.001. PMC 4597598. PMID 25524828.
- Douglas, Lewis A.; Varlinskaya, Elena I.; Spear, Linda P. (November 2003). "Novel-object place conditioning in adolescent and adult male and female rats: effects of social isolation". Physiology & Behavior. 80 (2–3): 317–325. doi:10.1016/j.physbeh.2003.08.003. ISSN 0031-9384. PMID 14637231.
- VARLINSKAYA, E (2008). "Social interactions in adolescent and adult Sprague–Dawley rats: Impact of social deprivation and test context familiarity". Behavioural Brain Research. 188 (2): 398–405. doi:10.1016/j.bbr.2007.11.024. PMC 3057041. PMID 18242726.
- Steinberg, Laurence; Graham, Sandra; O'Brien, Lia; Woolard, Jennifer; Cauffman, Elizabeth; Banich, Marie (2009-01-01). "Age Differences in Future Orientation and Delay Discounting". Child Development. 80 (1): 28–44. CiteSeerX 10.1.1.537.1994. doi:10.1111/j.1467-8624.2008.01244.x. ISSN 1467-8624. PMID 19236391.
- Ethridge, Paige; Kujawa, Autumn; Dirks, Melanie A.; Arfer, Kodi B.; Kessel, Ellen M.; Klein, Daniel N.; Weinberg, Anna (2017). "Neural responses to social and monetary reward in early adolescence and emerging adulthood". Psychophysiology. 54 (12): 1786–1799. doi:10.1111/psyp.12957. ISSN 1469-8986. PMC 5757310. PMID 28700084.
- Ernst, Monique; Nelson, Eric E.; Jazbec, Sandra; McClure, Erin B.; Monk, Christopher S.; Leibenluft, Ellen; Blair, James; Pine, Daniel S. (2005-05-01). "Amygdala and nucleus accumbens in responses to receipt and omission of gains in adults and adolescents". NeuroImage. 25 (4): 1279–1291. doi:10.1016/j.neuroimage.2004.12.038. ISSN 1053-8119. PMID 15850746.
- Whelan, Robert; McHugh, Louise A. (2009-04-01). "Temporal Discounting of Hypothetical Monetary Rewards by Adolescents, Adults, and Older Adults". The Psychological Record. 59 (2): 247–258. doi:10.1007/bf03395661. ISSN 0033-2933.
- Galván, Adriana; McGlennen, Kristine M. (2012-11-19). "Enhanced Striatal Sensitivity to Aversive Reinforcement in Adolescents versus Adults". Journal of Cognitive Neuroscience. 25 (2): 284–296. doi:10.1162/jocn_a_00326. ISSN 0898-929X. PMID 23163417.
- Harden, K. Paige; Tucker-Drob, Elliot M. (2011). "Individual differences in the development of sensation seeking and impulsivity during adolescence: Further evidence for a dual systems model". Developmental Psychology. 47 (3): 739–746. doi:10.1037/a0023279. PMID 21534657.
- Galvan, Adriana; Hare, Todd; Voss, Henning; Glover, Gary; Casey, B. J. (March 2007). "Risk-taking and the adolescent brain: who is at risk?". Developmental Science. 10 (2): F8–F14. doi:10.1111/j.1467-7687.2006.00579.x. ISSN 1363-755X. PMID 17286837.
- Simons-Morton, Bruce; Lerner, Neil; Singer, Jeremiah (November 2005). "The observed effects of teenage passengers on the risky driving behavior of teenage drivers". Accident Analysis and Prevention. 37 (6): 973–982. doi:10.1016/j.aap.2005.04.014. ISSN 0001-4575. PMID 15921652.
- Logue, Sheree; Chein, Jason; Gould, Thomas; Holliday, Erica; Steinberg, Laurence (January 2014). "Adolescent Mice, Unlike Adults, Consume More Alcohol in the Presence of Peers than Alone". Developmental Science. 17 (1): 79–85. doi:10.1111/desc.12101. ISSN 1363-755X. PMC 3869041. PMID 24341974.
- Chein, Jason; Albert, Dustin; O'Brien, Lia; Uckert, Kaitlyn; Steinberg, Laurence (2011-03-01). "Peers increase adolescent risk taking by enhancing activity in the brain's reward circuitry". Developmental Science. 14 (2): F1–F10. doi:10.1111/j.1467-7687.2010.01035.x. ISSN 1467-7687. PMC 3075496. PMID 21499511.
- Pfeifer, Jennifer H.; Peake, Shannon J. (2012). "Self-development: Integrating cognitive, socioemotional, and neuroimaging perspectives". Developmental Cognitive Neuroscience. 2 (1): 55–69. doi:10.1016/j.dcn.2011.07.012. ISSN 1878-9293. PMID 22682728.
- Schultz, W. (July 1998). "Predictive reward signal of dopamine neurons". Journal of Neurophysiology. 80 (1): 1–27. doi:10.1152/jn.1998.80.1.1. ISSN 0022-3077. PMID 9658025.
- Montague, P. Read; Hyman, Steven E.; Cohen, Jonathan D. (2004-10-14). "Computational roles for dopamine in behavioural control". Nature. 431 (7010): 760–767. Bibcode:2004Natur.431..760M. doi:10.1038/nature03015. ISSN 1476-4687. PMID 15483596.
- Spear, L. P. (June 2000). "The adolescent brain and age-related behavioral manifestations". Neuroscience and Biobehavioral Reviews. 24 (4): 417–463. doi:10.1016/s0149-7634(00)00014-2. ISSN 0149-7634. PMID 10817843.
- Teicher, M. H.; Andersen, S. L.; Hostetter, J. C. (1995-11-21). "Evidence for dopamine receptor pruning between adolescence and adulthood in striatum but not nucleus accumbens". Brain Research. Developmental Brain Research. 89 (2): 167–172. doi:10.1016/0165-3806(95)00109-q. ISSN 0165-3806. PMID 8612321.
- McCutcheon, James E.; Ebner, Stephanie R.; Loriaux, Amy L.; Roitman, Mitchell F. (2012-09-25). "Encoding of Aversion by Dopamine and the Nucleus Accumbens". Frontiers in Neuroscience. 6: 137. doi:10.3389/fnins.2012.00137. ISSN 1662-4548. PMC 3457027. PMID 23055953.
- Doremus-Fitzwater, Tamara L.; Varlinskaya, Elena I.; Spear, Linda P. (February 2010). "Motivational systems in adolescence: possible implications for age differences in substance abuse and other risk-taking behaviors". Brain and Cognition. 72 (1): 114–123. doi:10.1016/j.bandc.2009.08.008. ISSN 1090-2147. PMC 2814912. PMID 19762139.
- Wahlstrom, Dustin; White, Tonya; Luciana, Monica (April 2010). "Neurobehavioral evidence for changes in dopamine system activity during adolescence". Neuroscience and Biobehavioral Reviews. 34 (5): 631–648. doi:10.1016/j.neubiorev.2009.12.007. ISSN 1873-7528. PMC 2845533. PMID 20026110.
- Brenhouse, Heather C.; Andersen, Susan L. (August 2011). "Developmental trajectories during adolescence in males and females: a cross-species understanding of underlying brain changes". Neuroscience and Biobehavioral Reviews. 35 (8): 1687–1703. doi:10.1016/j.neubiorev.2011.04.013. ISSN 0149-7634. PMC 3134153. PMID 21600919.
- Ernst, Monique; Pine, Daniel S.; Hardin, Michael (March 2006). "Triadic model of the neurobiology of motivated behavior in adolescence". Psychological Medicine. 36 (3): 299–312. doi:10.1017/s0033291705005891. ISSN 1469-8978. PMC 2733162. PMID 16472412.
- Galvan, Adriana; Hare, Todd A.; Parra, Cindy E.; Penn, Jackie; Voss, Henning; Glover, Gary; Casey, B. J. (2006-06-21). "Earlier development of the accumbens relative to orbitofrontal cortex might underlie risk-taking behavior in adolescents". The Journal of Neuroscience. 26 (25): 6885–6892. doi:10.1523/JNEUROSCI.1062-06.2006. ISSN 1529-2401. PMID 16793895.
- Geier, C. F.; Terwilliger, R.; Teslovich, T.; Velanova, K.; Luna, B. (July 2010). "Immaturities in reward processing and its influence on inhibitory control in adolescence". Cerebral Cortex. 20 (7): 1613–1629. doi:10.1093/cercor/bhp225. ISSN 1460-2199. PMC 2882823. PMID 19875675.
- Kimchi, Eyal Y.; Torregrossa, Mary M.; Taylor, Jane R.; Laubach, Mark (July 2009). "Neuronal correlates of instrumental learning in the dorsal striatum". Journal of Neurophysiology. 102 (1): 475–489. doi:10.1152/jn.00262.2009. ISSN 0022-3077. PMC 2712266. PMID 19439679.
- Hart, Genevra; Leung, Beatrice K.; Balleine, Bernard W. (February 2014). "Dorsal and ventral streams: The distinct role of striatal subregions in the acquisition and performance of goal-directed actions". Neurobiology of Learning and Memory. 108: 104–118. doi:10.1016/j.nlm.2013.11.003. ISSN 1074-7427. PMC 4661143. PMID 24231424.
- [42][43][44][45][46]
- Van Leijenhorst, Linda; Zanolie, Kiki; Van Meel, Catharina S.; Westenberg, P. Michiel; Rombouts, Serge A. R. B.; Crone, Eveline A. (January 2010). "What motivates the adolescent? Brain regions mediating reward sensitivity across adolescence". Cerebral Cortex. 20 (1): 61–69. doi:10.1093/cercor/bhp078. ISSN 1460-2199. PMID 19406906.
- Guyer, Amanda E.; McClure-Tone, Erin B.; Shiffrin, Nina D.; Pine, Daniel S.; Nelson, Eric E. (July 2009). "Probing the neural correlates of anticipated peer evaluation in adolescence". Child Development. 80 (4): 1000–1015. doi:10.1111/j.1467-8624.2009.01313.x. ISSN 1467-8624. PMC 2791675. PMID 19630890.
- Bjork, James M.; Knutson, Brian; Fong, Grace W.; Caggiano, Daniel M.; Bennett, Shannon M.; Hommer, Daniel W. (2004-02-25). "Incentive-elicited brain activation in adolescents: similarities and differences from young adults". The Journal of Neuroscience. 24 (8): 1793–1802. doi:10.1523/JNEUROSCI.4862-03.2004. ISSN 1529-2401. PMID 14985419.
- Schneider, Sophia; Peters, Jan; Bromberg, Uli; Brassen, Stefanie; Miedl, Stephan F.; Banaschewski, Tobias; Barker, Gareth J.; Conrod, Patricia; Flor, Herta (January 2012). "Risk taking and the adolescent reward system: a potential common link to substance abuse". The American Journal of Psychiatry. 169 (1): 39–46. doi:10.1176/appi.ajp.2011.11030489. ISSN 1535-7228. PMID 21955931.
- Galvan, Adriana (2010-02-12). "Adolescent Development of the Reward System". Frontiers in Human Neuroscience. 4: 6. doi:10.3389/neuro.09.006.2010. ISSN 1662-5161. PMC 2826184. PMID 20179786.
- Paus, Tomás (February 2005). "Mapping brain maturation and cognitive development during adolescence". Trends in Cognitive Sciences. 9 (2): 60–68. doi:10.1016/j.tics.2004.12.008. ISSN 1364-6613. PMID 15668098.
- Huttenlocher, P. R.; Dabholkar, A. S. (1997-10-20). "Regional differences in synaptogenesis in human cerebral cortex". The Journal of Comparative Neurology. 387 (2): 167–178. doi:10.1002/(SICI)1096-9861(19971020)387:2<167::AID-CNE1>3.0.CO;2-Z. ISSN 0021-9967. PMID 9336221.
- Gogtay, Nitin; Giedd, Jay N.; Lusk, Leslie; Hayashi, Kiralee M.; Greenstein, Deanna; Vaituzis, A. Catherine; Nugent, Tom F.; Herman, David H.; Clasen, Liv S. (2004-05-25). "Dynamic mapping of human cortical development during childhood through early adulthood". Proceedings of the National Academy of Sciences of the United States of America. 101 (21): 8174–8179. Bibcode:2004PNAS..101.8174G. doi:10.1073/pnas.0402680101. ISSN 0027-8424. PMC 419576. PMID 15148381.
- Paus, Tomáš (2010). "Growth of white matter in the adolescent brain: Myelin or axon?". Brain and Cognition. 72 (1): 26–35. doi:10.1016/j.bandc.2009.06.002. PMID 19595493.
- Tau, Gregory Z.; Peterson, Bradley S. (January 2010). "Normal development of brain circuits". Neuropsychopharmacology. 35 (1): 147–168. doi:10.1038/npp.2009.115. ISSN 1740-634X. PMC 3055433. PMID 19794405.
- Casey, B. J.; Trainor, R. J.; Orendi, J. L.; Schubert, A. B.; Nystrom, L. E.; Giedd, J. N.; Castellanos, F. X.; Haxby, J. V.; Noll, D. C. (November 1997). "A Developmental Functional MRI Study of Prefrontal Activation during Performance of a Go-No-Go Task". Journal of Cognitive Neuroscience. 9 (6): 835–847. doi:10.1162/jocn.1997.9.6.835. ISSN 0898-929X. PMID 23964603.
- Durston, Sarah; Davidson, Matthew C.; Tottenham, Nim; Galvan, Adriana; Spicer, Julie; Fossella, John A.; Casey, B. J. (January 2006). "A shift from diffuse to focal cortical activity with development". Developmental Science. 9 (1): 1–8. doi:10.1111/j.1467-7687.2005.00454.x. ISSN 1363-755X. PMID 16445387.
- Ridderinkhof, K. Richard; Wildenberg, Wery P.M. van den; Segalowitz, Sidney J.; Carter, Cameron S. (2004). "Neurocognitive mechanisms of cognitive control: The role of prefrontal cortex in action selection, response inhibition, performance monitoring, and reward-based learning". Brain and Cognition. 56 (2): 129–140. doi:10.1016/j.bandc.2004.09.016. PMID 15518930.
- Van Leijenhorst, Linda; Moor, Bregtje Gunther; Macks, Zdeňa A. Op de; Rombouts, Serge A.R.B.; Westenberg, P. Michiel; Crone, Eveline A. (2010). "Adolescent risky decision-making: Neurocognitive development of reward and control regions". NeuroImage. 51 (1): 345–355. doi:10.1016/j.neuroimage.2010.02.038. PMID 20188198.
- Kohls, Gregor; Schulte-Rüther, Martin; Nehrkorn, Barbara; Müller, Kristin; Fink, Gereon R.; Kamp-Becker, Inge; Herpertz-Dahlmann, Beate; Schultz, Robert T.; Konrad, Kerstin (June 2013). "Reward system dysfunction in autism spectrum disorders". Social Cognitive and Affective Neuroscience. 8 (5): 565–572. doi:10.1093/scan/nss033. ISSN 1749-5016. PMC 3682440. PMID 22419119.
- Knutson, B.; Adams, C. M.; Fong, G. W.; Hommer, D. (2001-08-15). "Anticipation of increasing monetary reward selectively recruits nucleus accumbens". The Journal of Neuroscience. 21 (16): RC159. doi:10.1523/jneurosci.21-16-j0002.2001. ISSN 1529-2401. PMC 6763187. PMID 11459880.
- Bechara, A.; Damasio, A. R.; Damasio, H.; Anderson, S. W. (April 1994). "Insensitivity to future consequences following damage to human prefrontal cortex". Cognition. 50 (1–3): 7–15. doi:10.1016/0010-0277(94)90018-3. ISSN 0010-0277. PMID 8039375.
- Lejuez, C. W.; Read, Jennifer P.; Kahler, Christopher W.; Richards, Jerry B.; Ramsey, Susan E.; Stuart, Gregory L.; Strong, David R.; Brown, Richard A. (June 2002). "Evaluation of a behavioral measure of risk taking: the Balloon Analogue Risk Task (BART)". Journal of Experimental Psychology: Applied. 8 (2): 75–84. doi:10.1037/1076-898x.8.2.75. ISSN 1076-898X. PMID 12075692.
- Figner, Voelki (2004). "Risky decision making in a computer card game: An information integration experiment". Polish Psychological Bulletin. 35: 135–139.
- Christakou, Anastasia; Brammer, Mick; Rubia, Katya (2011-01-15). "Maturation of limbic corticostriatal activation and connectivity associated with developmental changes in temporal discounting". NeuroImage. 54 (2): 1344–1354. doi:10.1016/j.neuroimage.2010.08.067. ISSN 1095-9572. PMID 20816974.
- Rubia, Katya; Smith, Anna B.; Woolley, James; Nosarti, Chiara; Heyman, Isobel; Taylor, Eric; Brammer, Mick (December 2006). "Progressive increase of frontostriatal brain activation from childhood to adulthood during event-related tasks of cognitive control". Human Brain Mapping. 27 (12): 973–993. doi:10.1002/hbm.20237. ISSN 1065-9471. PMC 6871373. PMID 16683265.
- Rubia, Katya; Lim, Lena; Ecker, Christine; Halari, Rozmin; Giampietro, Vincent; Simmons, Andrew; Brammer, Michael; Smith, Anna (2013). "Effects of age and gender on neural networks of motor response inhibition: From adolescence to mid-adulthood". NeuroImage. 83: 690–703. doi:10.1016/j.neuroimage.2013.06.078. PMID 23845427.
- Tashjian, Sarah M.; Goldenberg, Diane; Galván, Adriana (2017). "Neural connectivity moderates the association between sleep and impulsivity in adolescents". Developmental Cognitive Neuroscience. 27: 35–44. doi:10.1016/j.dcn.2017.07.006. PMID 28777996.
- Tottenham, Nim; Hare, Todd A.; Casey, B. J. (2011). "Behavioral Assessment of Emotion Discrimination, Emotion Regulation, and Cognitive Control in Childhood, Adolescence, and Adulthood". Frontiers in Psychology. 2: 39. doi:10.3389/fpsyg.2011.00039. ISSN 1664-1078. PMC 3110936. PMID 21716604.
- Verbruggen, Frederick; Notebaert, Wim; Liefooghe, Baptist; Vandierendonck, André (2006-04-01). "Stimulus- and response-conflict-induced cognitive control in the flanker task". Psychonomic Bulletin & Review. 13 (2): 328–333. doi:10.3758/bf03193852. ISSN 1069-9384.
- Klopfer, Dale S. (2016-05-06). "Stroop Interference and Color-Word Similarity". Psychological Science. 7 (3): 150–157. doi:10.1111/j.1467-9280.1996.tb00348.x.
- Johnstone, Stuart J.; Dimoska, Aneta; Smith, Janette L.; Barry, Robert J.; Pleffer, Carly B.; Chiswick, Dale; Clarke, Adam R. (2007). "The development of stop-signal and Go/Nogo response inhibition in children aged 7–12 years: Performance and event-related potential indices". International Journal of Psychophysiology. 63 (1): 25–38. doi:10.1016/j.ijpsycho.2006.07.001. PMID 16919346.
- Unsworth, Nash; Schrock, Josef C.; Engle, Randall W. (2004). "Working Memory Capacity and the Antisaccade Task: Individual Differences in Voluntary Saccade Control". Journal of Experimental Psychology: Learning, Memory, and Cognition. 30 (6): 1302–1321. CiteSeerX 10.1.1.331.840. doi:10.1037/0278-7393.30.6.1302. PMID 15521806.
- Roper v. Simmons 543 U.S. 551 (2005)
- Graham v. Florida, 560 U.S. 48 (2010)
- Miller v. Alabama, 567 U.S. 460 (2012)
- Thompson v. Oklahoma, 487 U.S. 815 (1988)