Direct air capture
Direct air capture (DAC) is a process of capturing carbon dioxide (CO
2) directly from the ambient air (as opposed to capturing from point sources, such as a cement factory or biomass power plant) and generating a concentrated stream of CO
2 for sequestration or utilization. Carbon dioxide removal is achieved when ambient air makes contact with chemical media, typically an aqueous alkaline solvent[1] or functionalized sorbents.[2] These chemical media are subsequently stripped of CO2 through the application of energy (namely heat), resulting in a CO2 stream that can undergo dehydration and compression, while simultaneously regenerating the chemical media for reuse.
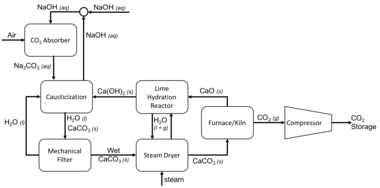
DAC is still in early stages of development,[3][4] though several commercial plants are in operation or planning across Europe and the US. Large-scale DAC deployment may be accelerated through pairing to utilization (e.g., enhanced oil recovery, production of carbon-neutral synthetic fuel and plastics, as well as beverage carbonation) or policy incentives such as 45Q or the California Low Carbon Fuel Standard. When combined with long-term storage of CO
2, DAC can act as a carbon dioxide removal tool whereby net negative emissions may be achieved, subject to a full cradle-to-grave lifecycle assessment.
DAC is not an alternative to traditional, point-source carbon capture and storage (CCS), but can be used to manage emissions from distributed sources, like exhaust fumes from cars.
The idea of using many small dispersed DAC scrubbers—analogous to live plants—to create environmentally significant reduction in CO
2 levels, has earned the technology a name of artificial trees in popular media.[5][6]
Methods of capture
Commercial techniques require large fans to push ambient air through a filter. There, a liquid solvent—usually amine-based or caustic—absorbs CO
2 from a gas.[7] For example, a common caustic solvent: sodium hydroxide reacts with CO
2 and precipitates a stable sodium carbonate. This carbonate is heated to produce a highly pure gaseous CO
2 stream.[8][9] sodium hydroxide can be recycled from sodium carbonate in a process of causticizing.[10] Alternatively, the CO
2 binds to solid sorbent in the process of chemisorption.[7] Through heat and vacuum, the CO
2 is then desorbed from the solid.[9][11]
Among the specific chemical processes that are being explored, three stand out: causticization with alkali and alkali-earth hydroxides, carbonation,[12] and organic−inorganic hybrid sorbents consisting of amines supported in porous adsorbents.[3]
Other explored methods
Moisture swing sorbent
In cyclical a process designed in 2012 by professor Klaus Lackner, the director of the Center for Negative Carbon Emissions (CNCE), dilute CO
2 can be efficiently separated using an anionic exchange polymer resin called Marathon MSA, which absorbs air CO
2 when dry, and releases it when exposed to moisture. The technology requires further research to determine its cost-effectiveness.[13][14][15]
Metal-organic frameworks
Other substances which can be used are Metal-organic frameworks (or MOF's).[16]
Environmental impact
Proponents of DAC argue that it is an essential component of climate change mitigation.[17][11][15] Researchers posit that DAC could help contribute to the goals of the Paris Climate Agreement (namely limiting the increase in global average temperature to well below 2 °C above pre-industrial levels). However, others claim that relying on this technology is risky and might postpone emission reduction under the notion that it will be possible to fix the problem later,[4][18] and suggest, that reducing emissions may be a better solution.[8][19]
DAC relying on amine-based absorption demands significant water input. It was estimated, that to capture 3.3 Gigatonnes of CO
2 a year would require 300 km3 of water, or 4% of the water used for irrigation. On the other hand, using sodium hydroxide needs far less water, but the substance itself is highly caustic and dangerous.[4]
DAC also requires much greater energy input in comparison to traditional capture from point sources, like flue gas, due to the low concentration of CO
2.[8][18] The theoretical minimum energy required to extract CO
2 from ambient air is about 250 kWh per tonne of CO
2, while capture from natural gas and coal power plants requires respectively about 100 and 65 kWh per tonne of CO
2.[17] Because of this implied demand for energy, some geoengineering promoters have proposed to use "small nuclear power plants" connected to DAC installations, potentially introducing a whole new set of environmental impacts.[4]
When DAC is combined with a carbon capture and storage (CCS) system, it can produce a negative emissions plant, but it would require a carbon-free electricity source. The use of any fossil-fuel-generated electricity would end up releasing more CO
2 to the atmosphere than it would capture.[18] Moreover, using DAC for enhanced oil recovery would cancel any supposed climate mitigation benefits.[4][9]
Economic viability
Practical applications of DAC include:
- enhanced oil recovery,[4]
- production of carbon-neutral synthetic fuel and plastics,[19][11][4]
- beverage carbonation,[20]
- carbon sequestration,[17]
- improving concrete strength,[20]
- creating carbon-neutral concrete alternative,[20]
- enhancing productivity of algae farms,[21]
- air purification of greenhouses.[21]
These applications require differing concentrations of CO
2 product formed from the captured gas. Forms of carbon sequestration such as geological storage require pure CO
2 products (concentration > 99%), while other applications such as agriculture can function with more dilute products (~ 5%). Since the air being processed through DAC originally contains 0.04% CO
2 (or 400 ppm), creation of a pure product through DAC requires a large amount of thermal energy to facilitate CO
2 bonding and thus is more expensive than a dilute product.[21]
DAC is not an alternative to traditional, point-source carbon capture and storage (CCS), rather it is a complementary technology that could be utilized to manage carbon emissions from distributed sources, fugitive emissions from the CCS network, and leakage from geological formations.[17][19][8] Because DAC can be deployed far from the source of pollution, synthetic fuel produced with this method can use already existing fuel transport infrastructure.[20]
One of the largest hurdles to implementing DAC is a cost required to separate CO
2 and air [21]. A study from 2011 estimated that a plant designed to capture 1 megatonne of CO
2 a year would cost $2.2 billion.[8] Other studies from the same period put the cost of DAC at $200–1000 per tonne of CO
2[17] and $600 per tonne.[8]
An economic study of a pilot plant in British Columbia, Canada, conducted from 2015 to 2018, estimated the cost at $94–232 per tonne of atmospheric CO
2 removed.[11][1] It is worth noting that the study was done by Carbon Engineering, which has financial interest in commercializing DAC technology.[1][9]
As of 2011, CO
2 capture costs for hydroxide based solvents generally cost $150 per tonne CO
2. Current liquid amine-based separation is $10–35 per tonne CO
2. Adsorption based CO
2 capture costs are between $30–200 per tonne CO
2. It is difficult to find a specific cost for DAC because each method has wide variation in sorbent regeneration and capital costs.[8]
Development
Carbon Engineering
It is a commercial DAC company founded in 2009 and backed, among others, by Bill Gates and Murray Edwards.[20][19] As of 2018, they run a pilot plant in British Columbia, Canada that has been in use since 2015[11] and is able to extract about a tonne of CO
2 a day.[4][19] An economic study of their pilot plant conducted from 2015 to 2018 estimated the cost at $94–232 per tonne of atmospheric CO
2 removed.[11][1]
While partnering with California energy company Greyrock, they convert a portion of its concentrated CO
2 into synthetic fuel, including gasoline, diesel, and jet fuel.[11][19]
The company uses a potassium hydroxide solution. It reacts with CO
2 to form potassium carbonate, which removes a certain amount of CO
2 from the air.[20]
Climeworks
Their first industrial scale DAC plant, which started operation in May, 2017 in Hinwil, in the canton of Zurich, Switzerland, is capable of capturing 900 tonnes of CO
2 per year. To lower its energy requirements, the plant uses heat from a local waste incineration plant. The CO
2 is used to increase vegetable yields in a nearby greenhouse.[22]
The company stated that it costs around $600 to capture one tonne of CO
2 from the air.[23][7]
Climeworks partnered with Reykjavik Energy in CarbFix project launched in 2007. In 2017, CarbFix2 project was started[24] and received funding from European Union's Horizon 2020 research program. The CarbFix2 pilot plant project runs alongside a geothermal power plant in Hellisheidi, Iceland. In this approach, CO
2 is injected 700 meters under the ground and mineralizes into basaltic bedrock forming carbonate minerals. DAC plant uses low-grade waste heat from the plant, effectively eliminating more CO
2 than they both produce. [4][25]
Global Thermostat
It is private company founded in 2010, located in Manhattan, New York, with a plant in Huntsville, Alabama.[20] Global Thermostat uses amine-based sorbents bound to carbon sponges to remove CO
2 from the atmosphere. The company has projects ranging from 40 to 50,000 tonne/year.[26]
The company claims to remove CO
2 for a $120 per tonne at its facility in Huntsville.[20]
Global Thermostat has closed deals with Coca-Cola (which aims to use DAC to source CO
2 for its carbonated beverages) and ExxonMobil which intends to pioneer a DAC‑to‑fuel business using Global Thermostat's technology.[20]
Prometheus Fuels
Is a start-up company based in Santa Cruz which launched out of Y Combinator in 2019 to remove CO2 from the air and turn it into zero-net-carbon gasoline and jet fuel.[27][28] The company uses a DAC technology, adsorbing CO2 from the air directly into process electrolytes, where it is converted into alcohols by electrocatalysis. The alcohols are then separated from the electrolytes using carbon nanotube membranes, and upgraded to gasoline and jet fuels. Since the process uses only electricity from renewable sources, the fuels are carbon neutral when used, emitting no net CO2 to the atmosphere.
Other companies
- Infinitree – earlier known as Kilimanjaro Energy and Global Research Technology. Part of US-based Carbon Sink. Demonstrated a pre-prototype of economically viable DAC technology in 2007.[9][29]
- Skytree – a company from Netherlands,[25]
- UK Carbon Capture and Storage Research Centre,[19]
- Antecy – a Dutch company founded in 2010,[30]
- Center for Negative Carbon Emission of Arizona State University
References
- Keith, David W.; Holmes, Geoffrey; St. Angelo, David; Heide, Kenton (June 7, 2018). "A Process for Capturing CO2 from the Atmosphere". Joule. 2 (8): 1573–1594. doi:10.1016/j.joule.2018.05.006.
- Beuttler, Christoph; Charles, Louise; Wurzbacher, Jan (2019). "The Role of Direct Air Capture in Mitigation of Anthropogenic Greenhouse Gas Emissions". Frontiers in Climate. 1. doi:10.3389/fclim.2019.00010. ISSN 2624-9553.
- Sanz-Pérez, E. S.; Murdock, C. R.; Didas, S. A.; Jones, C. W. (August 25, 2016). "Direct Capture of CO
2 from Ambient Air". Chem. Rev. 116 (19): 11840–11876. doi:10.1021/acs.chemrev.6b00173. PMID 27560307 – via ACS Publications. - "Direct Air Capture (Technology Factsheet)". Geoengineering Monitor. 2018-05-24. Retrieved 2019-08-27.
- Biello, David (2013-05-16). "400 PPM: Can Artificial Trees Help Pull CO2 from the Air?". Scientific American. Retrieved 2019-09-04.
- Burns, Judith (2009-08-27). "'Artificial trees' to cut carbon". BBC News | Science & Environment. Retrieved 2019-09-06.
- Smit, Berend; Reimer, Jeffrey A.; Oldenburg, Curtis M.; Bourg, Ian C (2014). Introduction to carbon capture and sequestration. London: Imperial College Press. ISBN 9781783263295. OCLC 872565493.
- "Direct Air Capture of CO2 with Chemicals: A Technology Assessment for the APS Panel on Public Affairs" (PDF). APS physics. 1 June 2011. Retrieved 2019-08-26.
- Chalmin, Anja (2019-07-16). "Direct Air Capture: Recent developments and future plans". Geoengineering Monitor. Retrieved 2019-08-27.
- Lackner, K. S.; Ziock, H.; Grimes, P. (1999). Carbon Dioxide Extraction from Air: Is It an Option?. Proceedings of the 24th Annual Technical Conference on Coal Utilization & Fuel Systems. pp. 885–896.
- Service, Robert F. (2018-06-07). "Cost plunges for capturing carbon dioxide from the air". Science | AAAS. Retrieved 2019-08-26.
- Nikulshina, V.; Ayesa, N.; Gálvez, M. E.; Stainfeld, A. (2016). "Feasibility of Na–Based Thermochemical Cycles for the Capture of CO
2 from air. Thermodynamic and Thermogravimetric Analyses". Chem. Eng. J. 140 (1–3): 62–70. doi:10.1016/j.cej.2007.09.007. - "Carbon Capture". Lenfest Center for Sustainable Energy. Archived from the original on 2012-12-20. Retrieved 2019-09-06.
- Biello, David (2013-05-16). "400 PPM: Can Artificial Trees Help Pull CO2 from the Air?". Scientific American. Retrieved 2019-09-04.
- Schiffman, Richard (2016-05-23). "Why CO2 'Air Capture' Could Be Key to Slowing Global Warming". Yale E360. Retrieved 2019-09-06.
- Yarris, Lynn (2015-03-17). "A Better Way of Scrubbing CO2". News Center. Retrieved 2019-09-07.
- "Novel carbon capture and utilisation technologies: research and climate aspects" (PDF). Science Advice for Policy by European Academies: 50. 23 May 2018. doi:10.26356/carboncapture. ISBN 978-3-9819415-6-2. ISSN 2568-4434.
- Ranjan, Manya; Herzog, Howard J. (2011). "Feasibility of air capture". Energy Procedia. 4: 2869–2876. doi:10.1016/j.egypro.2011.02.193. ISSN 1876-6102.
- Vidal, John (2018-02-04). "How Bill Gates aims to clean up the planet". The Observer. ISSN 0029-7712. Retrieved 2019-08-26.
- Diamandis, Peter H. (2019-08-23). "The Promise of Direct Air Capture: Making Stuff Out of Thin Air". Singularity Hub. Retrieved 2019-08-29.
- National Academies of Sciences, Engineering, and Medicine (2019). Negative Emissions Technologies and Reliable Sequestration: A Research Agenda. Washington, DC: The National Academies Press. doi:10.17226/25259. ISBN 978-0-309-48452-7.CS1 maint: multiple names: authors list (link)
- Doyle, Alister (2017-10-11). "From thin air to stone: greenhouse gas test starts in Iceland". Reuters. Retrieved 2019-09-04.
- Tollefson, Jeff (7 June 2018). "Sucking carbon dioxide from air is cheaper than scientists thought". Nature. Retrieved 2019-08-26.
- "Public Update on CarbFix". Climeworks. 2017-11-03. Retrieved 2019-09-02.
- Proctor, Darrell (2017-12-01). "Test of Carbon Capture Technology Underway at Iceland Geothermal Plant". POWER Magazine. Retrieved 2019-09-04.
- "Global Thermostat". Global Thermostat. Retrieved 2018-12-07.
- Service, Robert F. (2019-07-03). "This former playwright aims to turn solar and wind power into gasoline". Science | AAAS. Retrieved 2020-01-23.
- Brustein, Joshua (2019-04-30). "In Silicon Valley, the Quest to Make Gasoline Out of Thin Air". Bloomberg. Retrieved 2020-01-23.
- "First Successful Demonstration of Carbon Dioxide Air Capture Technology Achieved by Columbia University Scientist and Private Company". Columbia University. 2007-04-24. Archived from the original on 2010-06-22. Retrieved 2019-08-30.
- "Home". ANTECY. Retrieved 2019-08-27.