Alluvial fan
An alluvial fan is an accumulation of sediments shaped like a section of a shallow cone,[1] with its apex at a point source of sediments, such as a narrow canyon emerging from an escarpment.[2] They are characteristic of mountainous terrain in arid to semiarid climates,[3][4] but are also found in more humid environments subject to intense rainfall.[1] They range in area from less than 1 square kilometre (0.39 sq mi)[4][5] to almost 20,000 square kilometres (7,700 sq mi).[6]
Alluvial fans typically form where flow emerges from a confined channel and is free to spread out and infiltrate the surface of the fan. This reduces the carrying capacity of the flow and results in deposition of sediments.[1] The flow can take the form of infrequent debris flows or one or more ephemeral or perennial streams.[6]
Some of the largest alluvial fans are found along the Himalaya mountain front on the Indo-Gangetic plain.[6] A shift of the feeder channel (a nodal avulsion) can lead to catastrophic flooding, as occurred on the Kosi River fan in 2008.[7]
Size

Alluvial fans can exist on a wide spectrum of size scales, from only a few meters across at the base to as much as 150 kilometers across, with a slope of 1.5 to 25 degrees.[5] When numerous rivers and streams exit a mountain front onto a plain, the fans can combine to form a continuous apron. In arid to semi-arid environments, this is referred to as a bajada and in humid climates the continuous fan apron is called a piedmont alluvial fan.[8]
Formation
Alluvial fans usually form where a confined feeder channel exits a mountain front.[9][10] As the flow exits the feeder channel onto the fan surface, it is able to spread out into wide, shallow channels or to infiltrate the underlying fan. This reduces the carrying power of the flow and results in deposition of sediments. Blockage of channels by accumulated sediments causes flow to periodically break out (nodal avulsion) and shift to a part of the fan with a steeper gradient, where deposition resumes.[10]
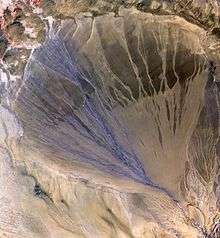
Alluvial fans can be debris-flow-dominated or stream-flow-dominated.[11][12] Debris-flow-dominated alluvial fans occur in all climates but are more common where the source rock is mudstone or matrix-rich saprolite rather than coarser, more permeable regolith. The abundance of fine-grained sediments encourages the initial hillslope failure and subsequent cohesive flow of debris. Saturation of clay-rich colluvium by locally intense thunderstorms promotes slope failure. The resulting debris flow travels down the feeder channel and onto the surface of the fan. Debris-flow-dominated alluvial fans are found to consist of a network of mostly inactive distributary channels in the upper fan that gives way to mid- to lower-level lobes. The channels tend to be filled by subsequent cohesive debris flows. Usually only one lobe is active at a time, and inactive lobes may develop desert varnish or develop a soil profile from eolian dust deposition, on time scales of 1,000 to 10,000 years.[13]
Stream-flow-dominated alluvial fans occur where there is perennial, seasonal, or ephemeral stream flow that feeds a system of distributary channels on the fan. In arid or semiarid climates, deposition is dominated by infrequent but intense rainfall that produces flash floods. As the flood recedes, it often leaves a lag of gravel deposits that have the appearance of a network of braided streams. Where the flow is more continuous, as with spring snow melt, a true network of meandering streams may develop. Such stream-flow-dominated alluvial fans can become enormous, and include the Kosi and other fans along the Himalaya mountain front in the Indo-Gangetic plain.[14]
An example of an active stream-flow-dominated alluvial fan is found in the semi-arid region between the Kunlun and Altun mountain ranges that form the southern border of the Taklamakan Desert in northwest China.[15] This particular fan is 60 kilometres (37 miles) in total length. One lobe of the fan has flowing streams that are continually depositing sediment so that the fan is still prograding into the alluvial plain. The feeder channels consist of straight channels as well as instances of braided channels because of the large volume of sediment sourced from the local uplands.[15]
Geomorphology
The sediment that results from erosion in elevated or mountainous regions ultimately flows into the primary streams in the region where the streams act as a drainage system and carries the sediment to the alluvial plain.[15] Due to the high degree of slope, the river/streams are typically classified as straight channels. Directly at the mouth of the feeder stream in the alluvial plain, the fan is narrow and is still subjected to high energy from the high degree of slope.[16] Once the sediment exits the feeder stream, the sediment is no longer confined to the channel walls. With this unconfinement, the sediments begin to fan out. The alluvial fan becomes wider with increasing distance from the mouth of the canyon.[17] When there is enough space in the alluvial plain for all of the sediment deposits to fan out without contacting other valleys walls or rivers, an unconfined alluvial fan develops. Unconfined alluvial fans allow sediments to naturally fan out and the shape of the fan is not influenced by other topological features.[8] When the alluvial plain is narrow or short parallel to depositional flow, the fan shape is ultimately affected. The biggest natural hazard on alluvial fans are floods and debris flows.[15] Floods on alluvial fans are commonly flash floods: they occur with little to no warning, usually have high velocities and sediment-transporting capability, and are of relatively short (several hours) duration.[15] Debris flows are a type of landslide that has been defined as a spatially continuous, rapidly moving mass of water and material that is composed mainly of coarse debris; typically, 20 to 80 percent of the particles are greater than 2 mm in diameter.[15]
Controls on depositional system evolution
Various environmental and geologic factors exhibit control on the deposition of alluvial fan deposits. The primary factor in alluvial fan environments is sediment supply. The sediment that eventually comprise the bedload and suspended load of the regional streams is sourced from the erosion of the associated highlands in the area.[8] Therefore, a high erosion rate corresponds to an increase in sediment in the streams which ultimately affects stream morphology. For example, a high sediment load is often associated with braided streams entering and within the alluvial plain.[15] Medium to low sediment loads in the feeder stream results in straight channels.4 Alluvial fans are built in response to erosion induced by tectonic uplift to create nearby mountain ranges/highlands.[8] This uplift is necessary for a source of erosion where the sediments ultimately are deposited in an alluvial fan regime in the alluvial plain. Tectonics can also affect the degree of stream gradients and cause changes in base level which may lead to incision into fan surfaces in the distal zone of the deposit.[17] An increase in precipitation would allow a higher water level in the streams which would allow for a greater amount of sediment to be carried along with it to be deposited in the alluvial plain.
Depositional/facies model
There are three primary zones, or facies, that exist within an alluvial fan deposit which include the proximal fan, medial fan, and distal fan with an overall proximal to distal fining.[5] In the proximal fan sub-environment, located just at the exit of the mountain range, coarse-grained massive gravel and blocks which contain relatively large portions of fine-grained matrix are highly prevalent.[8] The fan sediments gradually change to a higher proportion of sand and even powder or silty detritus further downstream in the lower parts of the fan.{cn}}The inclinations of conglomerate bodies in the proximal parts are typically 10-15 degrees and on the outskirts, they are up to 30 degrees. In the medial fan, the morphology depicts characteristics of frequent changes of inclined planar layered gravels, clast-supported and trough inclined stratified gravels, massive matrix-supported muddy gravels, and planar inclined sands. The dimensions of the alluvial fan deposit are often extremely wide in the distal areas. In the distal region, there is a prevalence of sands with planar and trough slanted stratification over clast-supported pebbles with trough slope stratification along with horizontally laminated silty sediments. After initial deposition of the fan, the deposits are commonly overlain by clay/marl sediments.5 In rare cases, this can be a potential trap for hydrocarbons and a possible exploration target in the sands underneath.[8] A fining upwards sequence exists in aggrading alluvial fans with distal fining laterally from the mouth. A coarsening upwards sequence comprises a prograding fan with down-fan fining.[15] Controls on aggradation and progradation include sediment supply and accommodation space for the region.[17]
In arid climates
Alluvial fans are often found in desert areas often subjected to periodic flash floods from nearby thunderstorms in local hills. The typical watercourse in an arid climate has a large, funnel-shaped basin at the top, leading to a narrow defile, which opens out into an alluvial fan at the bottom. Multiple braided streams are usually present and active during water flows.
Phreatophytes are plants that are often concentrated at the base of alluvial fans. They have long tap roots 30 to 50 feet (9.1 to 15.2 m) to reach water that has seeped through the fan and hit an impermeable layer, sometimes collecting in springs and seeps. These stands of shrubs cling to the soil at their bases and often form islands of habitat for many animals as the wind blows the sand around the bushes away.
In humid climates
Alluvial fans also develop in wetter climates. In Nepal the Koshi River has built a megafan covering some 15,000 km2 (5,800 sq mi) below its exit from Himalayan foothills onto the nearly level plains where the river traverses into India before joining the Ganges. Along the upper Koshi tributaries, tectonic forces elevate the Himalayas several millimeters annually. Uplift is approximately in equilibrium with erosion, so the river annually carries some 100 million cubic meters (3.5 billion cu ft) of sediment as it exits the mountains. Deposition of this magnitude over millions of years is more than sufficient to account for the megafan.[17]
All along the interface between the Indo-Gangetic Plain and the Himalaya in India, Pakistan, Nepal and Bhutan the outermost, lowest Siwalik foothills are built of poorly consolidated sedimentary rocks that have eroded into a wide, continuous alluvial apron called Bhabar in Hindi and Nepali. Despite human overpopulation on the plains, this bhabar zone is highly malarial and has remained largely uninhabited.
In North America, streams flowing into California's Central Valley have deposited smaller but still extensive alluvial fans, such as that of the Kings River flowing out of the Sierra Nevada which creates a low divide, turning the south end of the San Joaquin Valley into an endorheic basin without a connection to the ocean.
Flood hazards
Alluvial fans are subject to flooding[16][15] and can be even more dangerous than the upstream canyons that feed them. Their slightly convex perpendicular surfaces cause water to spread widely until there is no zone of refuge. If the gradient is steep, active transport of materials down the fan creates a moving substrate that is inhospitable to travel on foot or wheels. But as the gradient diminishes downslope, water comes down from above faster than it can flow away downstream, and may pond to hazardous depths.
In the case of the Koshi River, the huge sediment load and megafan's slightly convex transverse surface conspire against engineering efforts to contain peak flows inside manmade embankments. In August 2008 high monsoon flows breached the embankment, diverting most of the river into an unprotected ancient channel and across surrounding lands with high population density. Over a million people were rendered homeless, about a thousand lost their lives and thousands of hectares of crops were destroyed. The Koshi is known as the Sorrow of Bihar for contributing disproportionately to India's death tolls in flooding, which exceed those of all countries except Bangladesh.
In the Solar System
Mars
Alluvial fans are also found on Mars descending from some crater rims over their flatter floors.[18] Three alluvial fans have been found in Saheki Crater. These fans confirmed past fluvial flow on the planet and further supported the theory that liquid water was once present in some form on the Martian surface.[5] In addition, observations of fans in Gale crater made by satellites from orbit have now been confirmed by the discovery of fluvial sediments by the Curiosity rover.[19]
Titan
Alluvial fans have been observed by the Cassini-Huygens mission on Titan using the Cassini orbiter's synthetic aperture radar (SAR) instrument.[20] These fans are more common in the drier mid-latitudes at the end of methane/ethane rivers where it is thought that frequent wetting and drying occur due to precipitation, much like arid fans on Earth. Radar imaging suggests that fan material is most likely composed of round grains of water ice or solid organic compounds about two centimetres in diameter.
Gallery
Ground-level view of a large alluvial fan in Death Valley Alluvial Fan in southern Iran. Image from NASA's Terra satellite - Alluvial fan in Death Valley, California, seen from source area of Black Mountains at Dante's View.
See also
- Alluvium – Loose soil or sediment that is eroded and redeposited in a non-marine setting
- Alluvial plain – Region on which rivers have deposited sediment
- Floodplain – Land adjacent to a stream or river which is flooded during periods of high discharge
- Placer deposit
- River delta – Silt deposition landform at the mouth of a river
- Subaqueous fan
References and notes
- Boggs, Sam, Jr. (2006). Principles of sedimentology and stratigraphy (4th ed.). Upper Saddle River, N.J.: Pearson Prentice Hall. pp. 246–250. ISBN 0131547283.
- Leeder, Mike (2011). Sedimentology and sedimentary basins : from turbulence to tectonics (2nd ed.). Chichester, West Sussex, UK: Wiley-Blackwell. pp. 282–294. ISBN 9781405177832.
- Shelton, John S. (1966). Geology Illustrated. San Francisco and London: W.H. Freeman and Company. p. 154.
- Blatt, Harvey; Middletone, Gerard; Murray, Raymond (1980). Origin of sedimentary rocks (2d ed.). Englewood Cliffs, N.J.: Prentice-Hall. pp. 629–632. ISBN 0136427103.
- Morgan, A. M.; Howard, A. D.; Hobley, D. E. J.; Moore, J. M.; Dietrich, W. E.; Williams, R. M. E.; Burr, D. M.; Grant, J. A.; Wilson, S. A. (February 1, 2014). "Sedimentology and climatic environment of alluvial fans in the martian Saheki crater and a comparison with terrestrial fans in the Atacama Desert" (PDF). Icarus. 229: 131–156. Bibcode:2014Icar..229..131M. doi:10.1016/j.icarus.2013.11.007.
- Leeder 2011, p.285
- Leeder 2011, p.289
- American Geological Institute. Dictionary of Geological Terms. New York: Dolphin Books, 1962.
- Boggs 2006, pp.246-248
- Leeder 2011, pp.285-289
- Boggs 2006, p.247
- Leeder 2011, pp.287-289
- Leeder 2011, pp.287-288
- Leeder 2011, pp.288-289
- Committee on Alluvial Fan Flooding, Water Science and Technology Board, Commission on Geosciences, Environment, and Resources, National Research Council. (1996). Alluvial fan flooding. Washington, D.C.: National Academy Press. ISBN 978-0-309-05542-0.CS1 maint: uses authors parameter (link)
- Cazanacli, Dan; Paola, Chris; Parker, Gary (2002). "Experimental Steep, Braided Flow: Application to Flooding Risk on Fans". Journal of Hydraulic Engineering. 128 (3): 322. doi:10.1061/(ASCE)0733-9429(2002)128:3(322).
- National Aeronautics and Space Administration. "Geomorphology from Space; Fluvial Landforms, Chapter 4: Plate F-19". Archived from the original on September 27, 2011. Retrieved April 18, 2009.
- Kraal, Erin R.; Asphaug, Erik; Moore, Jeffery M.; Howard, Alan; Bredt, Adam (March 2008). "Catalogue of large alluvial fans in martian impact craters". Icarus. 194 (1): 101–110. Bibcode:2008Icar..194..101K. doi:10.1016/j.icarus.2007.09.028. ISSN 0019-1035.
- Harwood, William; Wall, Mike (September 27, 2012). "Mars rover Curiosity finds ancient stream bed". CBS News. Retrieved January 21, 2016.
- J. Radebaugh; et al. (2013). "Alluvial Fans on Titan Reveal Materials, Processes and Regional Conditions" (PDF). 44th Lunar and Planetary Science Conference. Retrieved January 21, 2016.
External links
![]() |
Wikimedia Commons has media related to Alluvial fans. |
- Howard, J.M.; Moore, A.D. (2005). "Large alluvial fans on Mars" (PDF). Journal of Geophysical Research. 110: E04005. Bibcode:2005JGRE..11004005M. doi:10.1029/2004JE002352.