Two-dimensional semiconductor
A two-dimensional semiconductor (also known as 2D semiconductor) is a type of natural semiconductor with thicknesses on the atomic scale. The rising research attention towards 2D semiconductors started with a discovery by Geim and Novoselov et al. in 2004,[1] when they reported a new semiconducting material graphene, a flat monolayer of carbon atoms arranged in a 2D honeycomb lattice. A 2D monolayer semiconductor is significant because it exhibits stronger piezoelectric coupling than traditionally employed bulk forms, which enables 2D materials applications in new electronic components used for sensing and actuating.[2] In this emergent field of research in solid-state physics, the main focus is currently on designing nanoelectronic components by the use of graphene as electrical conductor, hexagonal boron nitride as electrical insulator, and a transition metal dichalcogenide as semiconductor.[3][4]
Materials
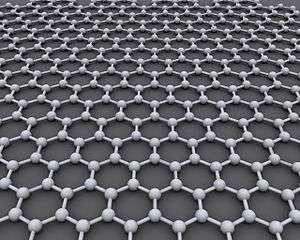
Graphene
Graphene's two surfaces are single sheets of carbon atoms arranged in a hexagonal honeycomb lattice. Having two surfaces and lacking bulk makes it the thinnest possible material but also 5 times stronger than steel due to pi and sigma orbital bonds. Graphene has high electron mobility and high thermal conductivity. Although graphene can be used in different applications, one issue regarding graphene is its lack of a band gap, which poses a problem in particular with digital electronics because it is unable to switch off field-effect transistors (FETs).[3] Nanosheets of other group-IV elements (Si, Ge and Sn) present structural and electronic properties similar to graphene.[5]
-side-3D-balls.png)
Hexagonal boron nitride
Monolayer hexagonal boron nitride (h-BN), also known as โwhite grapheneโ, is structurally similar to graphite and features a honeycomb arrangement with alternating boron and nitrogen atoms in place of carbon. h-BN has a higher energy gap (5.97 eV) than graphene, thus functions as an insulator instead of a semimetal.[6] However, it can also function as a semiconductor with enhanced conductivity due to its zigzag sharp edges and vacancies. h-BN is often used as substrate and barrier due to its insulating property. Furthermore, h-BN also has a large thermal conductivity and mechanical strength. Thus, it can be employed as a support for metal catalyst due to its chemical, thermal, acid-base stability and high thermal conductance.

Transition metal dichalcogenides
Transition metal dichalcogenide monolayers (TMDs or TMDCs) are a class of two-dimensional materials, which have the chemical formula MX2, where M represents transition metals from group VI, V and VI, and X represents a chalcogen such as sulfur, selenium or tellurium.[7] MoS2, MoSe2, MoTe2, WS2 and WSe2 are TMDCs. TMDCs have layered structure with a plane of metal atoms in between two planes of chalcogen atoms as shown in Figure 1. Each layer is bonded strongly in plane, but weakly in interlayers. Therefore, TMDCs can be easily exfoliated into atomically thin layers through various methods. TMDCs show layer-dependent optical and electrical properties. When exfoliated into monolayers, the band gaps of several TMDCs change from indirect to direct,[8] which lead to broad applications in nanoelectronics[3], optoelectronics[9][10], and quantum computing [11] .
Synthesis
2D semiconductor materials are often synthesized using a chemical vapor deposition (CVD) method. Because CVD can provide large-area, high-quality, and well-controlled layered growth of 2D semiconductor materials, it also allows synthesis of two-dimensional heterojunctions.[12] When building devices by stacking different 2D materials, mechanical exfoliation followed by transferring is often used.[4][7] Other possible synthesis methods include chemical exfoliation, hydrothermal synthesis, and thermal decomposition.
Device applications
Some devices applications include electronic devices[13], photonic and energy harvesting devices, and flexible and transparent substrates. Furthermore, applications on quantum computing qubit devices have been proposed[11].
Electronic devices
2D Semiconductors can be used as transistors for digital electronics.[3] The impure charges at the interfaces that are free of dangling bonds, allow for 2D semiconductors to run low-power devices. The 2D semiconductor interface has future potential in nano circuits due to its ability to optimize and regulate thermal transfer.
Energy and harvesting devices
2D semiconductors have potential for application in the harvesting of solar energy. The atomically thin structure allows for lower surface recombination velocity, which leads to better photocurrent conduction. An improvement on solar cell performance has been shown, while stacking 2D semiconductors with multilayers of graphene.[15]
Flexible and transparent substrates
The thin layer of 2D materials can be used for flexible electronics. In particular, 2D MoS2 can be used to create thin displays and wearable electronics due to its out of plane flexibility, strong covalent bonds, and diverse electronic properties.[7]
Magnetic NEMS
2D layered magnetic materials are attractive building blocks for nanoelectromechanical systems (NEMS): while they share high stiffness and strength and low mass with other 2D materials, they are magnetically active. Among the large class of newly emerged 2D layered magnetic materials, of particular interest is few-layer CrI3, whose magnetic ground state consists of antiferromagnetically coupled ferromagnetic (FM) monolayers with out-of-plane easy axis. The interlayer exchange interaction is relatively weak, a magnetic field on the order of 0.5 T in the out-of-plane (๐) direction can induce spin-flip transition in bilayer CrI3. Remarkable phenomena and device concepts based on detecting and controlling the interlayer magnetic state have been recently demonstrated, including spin-filter giant magnetoresistance, magnetic switching by electric field or electrostatic doping, and spin transistors. The coupling between the magnetic and mechanical properties in atomically thin materials, the basis for 2D magnetic NEMS, however, remains elusive although NEMS made of thicker magnetic materials or coated with FM metals have been studied.
References
- Novoselov, K. S. (2004). "Electric Field Effect in Atomically Thin Carbon Films". Science. 306 (5696): 666โ669. arXiv:cond-mat/0410550. Bibcode:2004Sci...306..666N. doi:10.1126/science.1102896. ISSN 0036-8075. PMID 15499015.
- Song, Xiufeng; Hu, Jinlian; Zeng, Haibo (2013). "Two-dimensional semiconductors: recent progress and future perspectives". Journal of Materials Chemistry C. 1 (17): 2952. doi:10.1039/C3TC00710C.
- Radisavljevic, B.; Radenovic, A.; Brivio, J.; Giacometti, V.; Kis, A. (2011). "Single-layer MoS2 transistors". Nature Nanotechnology. 6 (3): 147โ150. Bibcode:2011NatNa...6..147R. doi:10.1038/nnano.2010.279. PMID 21278752.
- Geim, A. K.; Grigorieva, I. V. (2013). "Van der Waals heterostructures". Nature. 499 (7459): 419โ425. arXiv:1307.6718. doi:10.1038/nature12385. ISSN 0028-0836. PMID 23887427.
- Garcia, J. C.; de Lima, D. B.; Assali, L. V. C.; Justo, J. F. (2011). "Group IV graphene- and graphane-like nanosheets". J. Phys. Chem. C. 115 (27): 13242. arXiv:1204.2875. doi:10.1021/jp203657w.
- Dean, C. R.; Young, A. F.; Meric, I.; Lee, C.; Wang, L.; Sorgenfrei, S.; Watanabe, K.; Taniguchi, T.; Kim, P.; Shepard, K. L.; Hone, J. (2010). "Boron nitride substrates for high-quality graphene electronics". Nature Nanotechnology. 5 (10): 722โ726. arXiv:1005.4917. Bibcode:2010NatNa...5..722D. doi:10.1038/nnano.2010.172. ISSN 1748-3387. PMID 20729834.
- Wang, Qing Hua; Kalantar-Zadeh, Kourosh; Kis, Andras; Coleman, Jonathan N.; Strano, Michael S. (2012). "Electronics and optoelectronics of two-dimensional transition metal dichalcogenides". Nature Nanotechnology. 7 (11): 699โ712. Bibcode:2012NatNa...7..699W. doi:10.1038/nnano.2012.193. ISSN 1748-3387. PMID 23132225.
- Kuc, A.; Zibouche, N.; Heine, T. (2011). "Influence of quantum confinement on the electronic structure of the transition metal sulfideTS2". Physical Review B. 83 (24): 245213. arXiv:1104.3670. Bibcode:2011PhRvB..83x5213K. doi:10.1103/PhysRevB.83.245213. ISSN 1098-0121.
- Wilson, J.A.; Yoffe, A.D. (1969). "The transition metal dichalcogenides discussion and interpretation of the observed optical, electrical and structural properties". Advances in Physics. 18 (73): 193โ335. Bibcode:1969AdPhy..18..193W. doi:10.1080/00018736900101307. ISSN 0001-8732.
- Yoffe, A D (1973). "Layer Compounds". Annual Review of Materials Science. 3 (1): 147โ170. Bibcode:1973AnRMS...3..147Y. doi:10.1146/annurev.ms.03.080173.001051. ISSN 0084-6600.
- B. Lucatto; et al. (2019). "Charge qubit in van der Waals heterostructures". Physical Review B. 100 (12): 121406. arXiv:1904.10785. doi:10.1103/PhysRevB.100.121406.
- Duan, Xidong; Wang, Chen; Shaw, Jonathan C.; Cheng, Rui; Chen, Yu; Li, Honglai; Wu, Xueping; Tang, Ying; Zhang, Qinling; Pan, Anlian; Jiang, Jianhui; Yu, Ruqing; Huang, Yu; Duan, Xiangfeng (2014). "Lateral epitaxial growth of two-dimensional layered semiconductor heterojunctions". Nature Nanotechnology. 9 (12): 1024โ1030. Bibcode:2014NatNa...9.1024D. doi:10.1038/nnano.2014.222. ISSN 1748-3387. PMID 25262331.
- "Stanford 2D Device Trends".
- Ong, Zhun-Yong; Bae, Myung-Ho (2019). "Energy dissipation in van der Waals 2D devices". 2D Materials. 6 (3): 032005. arXiv:1904.09752. doi:10.1088/2053-1583/ab20ea.
- Shanmugam, Mariyappan; Jacobs-Gedrim, Robin; Song, Eui Sang; Yu, Bin (2014). "Two-dimensional layered semiconductor/graphene heterostructures for solar photovoltaic applications". Nanoscale. 6 (21): 12682โ12689. Bibcode:2014Nanos...612682S. doi:10.1039/C4NR03334E. ISSN 2040-3364. PMID 25210837.