Somatic embryogenesis
Somatic embryogenesis is an artificial process in which a plant or embryo is derived from a single somatic cell.[1] Somatic embryos are formed from plant cells that are not normally involved in the development of embryos, i.e. ordinary plant tissue. No endosperm or seed coat is formed around a somatic embryo.
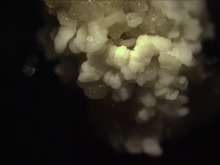
Cells derived from competent source tissue are cultured to form an undifferentiated mass of cells called a callus. Plant growth regulators in the tissue culture medium can be manipulated to induce callus formation and subsequently changed to induce embryos to form the callus. The ratio of different plant growth regulators required to induce callus or embryo formation varies with the type of plant.[2] Somatic embryos are mainly produced in vitro and for laboratory purposes, using either solid or liquid nutrient media which contain plant growth regulators (PGR’s). The main PGRs used are auxins but can contain cytokinin in a smaller amount.[3] Shoots and roots are monopolar while somatic embryos are bipolar, allowing them to form a whole plant without culturing on multiple media types. Somatic embryogenesis has served as a model to understand the physiological and biochemical events that occur during plant developmental processes as well as a component to biotechnological advancement.[4] The first documentation of somatic embryogenesis was by Steward et al. in 1958 and Reinert in 1959 with carrot cell suspension cultures.[5][6]
Direct and indirect embryogenesis
Somatic embryogenesis has been described to occur in two ways: directly or indirectly.[7]
Direct embryogenesis
occurs when embryos are started directly from explant tissue creating an identical clone.
Indirect embryogenesis
occurs when explants produced undifferentiated, or partially differentiated, cells (often referred to as callus) which then is maintained or differentiated into plant tissues such as leaf, stem, or roots. 2,4-Dichlorophenoxyacetic acid (2,4-D), 6-Benzylaminopurine (BAP) and Gibberellic acid (GA) has been used for development of indirect somatic embryos in strawberry (Fragaria ananassa) [8]
Plant regeneration by somatic embryogenesis
Plant regeneration via somatic embryogenesis occurs in five steps: initiation of embryogenic cultures, proliferation of embryogenic cultures, prematuration of somatic embryos, maturation of somatic embryos and plant development on nonspecific media. Initiation and proliferation occur on a medium rich in auxin, which induces differentiation of localized meristematic cells. The auxin typically used is 2,4-D. Once transferred to a medium with low or no auxin, these cells can then develop into mature embryos. Germination of the somatic embryo can only occur when it is mature enough to have functional root and shoot apices [3]
Factors influencing
Factors and mechanisms controlling cell differentiation in somatic embryos are relatively ambiguous. Certain compounds excreted by plant tissue cultures and found in culture media have been shown necessary to coordinate cell division and morphological changes.[9] These compounds have been identified by Chung et al.[10] as various polysaccharides, amino acids, growth regulators, vitamins, low molecular weight compounds and polypeptides. Several signaling molecules known to influence or control the formation of somatic embryos have been found and include extracellular proteins, arabinogalactan proteins and lipochitooligosaccharides. Temperature and lighting can also affect the maturation of the somatic embryo.
Applications
Applications of this process include: clonal propagation of genetically uniform plant material; elimination of viruses; provision of source tissue for genetic transformation; generation of whole plants from single cells called protoplasts; development of synthetic seed technology.[1]
Uses of somatic embryogenesis
- Plant transformations[11]
- Mass propagation[12]
Forestry related example
The development of somatic embryogenesis procedures has given rise to research on seed storage proteins (SSPs) of woody plants for tree species of commercial importance, i.e., mainly gymnosperms, including white spruce. In this area of study, SSPs are used as markers to determine the embryogenic potential and competency of the embryogenic system to produce a somatic embryo biochemically similar to its zygotic counterpart (Flinn et al. 1991, Beardmore et al. 1997).[13][14]
Grossnickle et al. (1992)[15] compared interior spruce seedlings with emblings during nursery development and through a stock quality assessment program immediately before field outplanting. Seedling shoot height, root collar diameter, and dry weight increased at a greater rate in seedlings than in emblings during the first half of the first growing season, but thereafter shoot growth was similar among all plants. By the end of the growing season, seedlings were 70% taller than emblings, had greater root collar diameter, and greater shoot dry weight. Root dry weight increased more rapidly in seedlings than in emblings during the early growing season
During fall acclimation, the pattern of increasing dormancy release index and increasing tolerance to freezing was similar in both seedlings and emblings. Root growth capacity decreased then increased during fall acclimation, with the increase being greater in seedlings.
Assessment of stock quality just prior to planting showed that: emblings had greater water use efficiency with decreasing predawn shoot water potential compared with seedlings; seedlings and emblings had similar water movement capability at both high and low root temperatures; net photosynthesis and needle conductance at low root temperatures were greater in seedlings than in emblings; and seedlings had greater root growth than emblings at 22 °C root, but root growth among all plants was low at 7.5 °C root temperature.
Growth and survival of interior spruce 313B Styroblock® seedlings and emblings after outplanting on a reforestation site were determined by Grossnickle and Major (1992).[16] For both seedlings and emblings, osmotic potential at saturation (ψsat) and turgor loss point (ψtip) increased from a low of -1.82 and -2.22 MPa, respectively, just prior to planting to a seasonal high of -1.09 and -1.21 MPa, respectively, during active shoot elongation. Thereafter, seedlings and emblings (ψsat) and (ψtip) declined to -2.00 and -2.45 MPa, respectively, at the end of the growing season, which coincided with the steady decline in site temperatures and a cessation of height growth. In general, seedlings and emblings had similar ψsat and ψtip values through the growing season, and also had similar shifts in seasonal patterns of maximum modulus of elasticity, sympalstic fraction, and relative water content at turgor loss point.
Grossnickle and Major (1992)[16] found that year-old and current-year needles of both seedlings and emblings had a similar decline in needle conductance with increasing vapour pressure deficit. Response surface models of current-year needles net photosynthesis (Pn) response to vapour pressure deficit (VPD) and photosynthetically active radiation (PAR) showed that emblings had 15% greater Pn at VPD of less than 3.0 kPa and PAR greater than 1000 μmol m−2s−1. Year-old and current-year needles of seedlings and emblings showed similar patterns of water use efficiency.
Rates of shoot growth in seedlings and emblings through the growing season were also similar to one another. Seedlings had larger shoot systems both at the time of planting and at the end of the growing season. Seedlings also had greater root development than emblings through the growing season, but root:shoot ratios for the 2 stock types were similar at the end of the growing season, when the survival rates for seedlings and emblings were 96% and 99%, respectively.
Tracking and fate maps
Understanding the formation of a somatic embryo through establishment of morphological and molecular markers is important for construction of a fate map. The fate map is the foundation in which to build further research and experimentation. Two methods exist to construct a fate map: synchronous cell-division and time-lapse tracking. The latter typically works more consistently because of cell-cycle-altering chemicals and centrifuging involved in synchronous cell-division.[17]
Angiosperms
Embryo development in angiosperms is divided into several steps. The zygote is divided asymmetrically forming a small apical cell and large basal cell. The organizational pattern is formed in the globular stage and the embryo then transitions to the cotyledonary stage.[18] Embryo development differs in monocots and dicots. Dicots pass through the globular, heart-shaped, and torpedo stages while monocots pass through globular, scutellar, and coleoptilar stages.[19]
Many culture systems induce and maintain somatic embryogenesis by continuous exposure to 2,4-dichlorophenoxyacetic acid. Abscisic acid has been reported to induce somatic embryogenesis in seedlings. After callus formation, culturing on a low auxin or hormone free media will promote somatic embryo growth and root formation. In monocots, embryogenic capability is usually restricted to tissues with embryogenic or meristematic origin. Somatic cells of monocots differentiate quickly and then lose mitotic and morphogenic capability. Differences of auxin sensitivity in embryogenic callus growth between different genotypes of the same species show how variable auxin responses can be.[20]
Carrot Daucus carota was the first and most understood species with regard to developmental pathways and molecular mechanisms.[17] Time-lapse tracking by Toonen et al. (1994) showed that morphology of competent cells can vary based on shape and cytoplasm density. Five types of cells were identified from embryonic suspension: spherical cytoplasm-rich, spherical vacuolated, oval vacuolated, elongated vacuolated, and irregular shaped cells. Each type of cell multiplied with certain geometric symmetry. They developed into symmetrical, asymmetrical, and aberrantly-shaped cell clusters that eventually formed embryos at different frequencies.[21] This indicates that organized growth polarity do not always exist in somatic embryogenesis.[17]
Gymnosperms
Embryo development in gymnosperms occurs in three phases. Proembryogeny includes all stages prior to suspensor elongation. Early embryogeny includes all stages after suspensor elongation but before root meristem development. Late embryogeny includes development of root and shoot meristems.[18] Time-lapse tracking in Norway Spruce Picea abies revealed that neither single cytoplasmic-rich cells nor vacuolated cells developed into embryos. Proembryogenic masses (PEMs), an intermediate between unorganized cells and an embryo composed of cytoplasmic-rich cells next to a vacuolated cell, are stimulated with auxin and cytokinin. Gradual removal of auxin and cytokinin and introduction of abscisic acid (ABA) will allow an embryo to form.[17] Using somatic embryogenesis has been considered for mass production of vegetatively propagated conifer clones and cryopreservation of germplasm. However, the use of this technology for reforestation and tree breeding of conifers is in its infancy.[22][23]
See also
References
- Sahoo, Jyoti Prakash (2018-06-11). "Organogenesis and somatic embryogenesis". doi:10.13140/rg.2.2.26278.57928. Cite journal requires
|journal=
(help) - http://www.accessexcellence.org/LC/ST/st2bgplant.html Plant Tissue Culture
- E.F. George et al. (eds.), Plant Propagation by Tissue Culture 3rd Edition, 335-354.
- Quiroz-Figueroa, F. R., Rojas-Herrera, R., Galaz-Avalos, R. M., and Loyola- Vargas, V. M. 2006. Embryo production through somatic embryogenesis can be used to study cell differentiation in plants. Plant Cell Tiss. Org. Cult. 86: 285–301.
- Steward, F.C., Mapes, M.O., and Smlth, J. (1958). Growth and organized development of cultured cells. I. Growth and division of freely suspended cells. Am. J. Bot. 45, 693-703.
- Reinert J (1959) Uber die kontrolle der morphogenese und die induktion von adventivembryonen an gew- ebekulturen aus karotten. Planta 53:318–333
- Sharp et al. (1980). In: Horticultural Reviews, Vol. 2. (janick, J., ed.). AVI Publishing Co, Westport, Conn., USA, p. 268.
- Waghmare, S. G., Pawar, K. R., and Tabe, R. 2017. Somatic embryogenesis in Strawberry (Fragaria ananassa) var. Camarosa. Global Journal of Bioscience and Biotechnology 6(2): 309 - 313.
- Warren, G.S., Fowler, M.W. 1981. Physiological interactions during the initial stages of embryogenesis in cultures of Daucus carota L. New Phytol 87:481-486.
- Chung, W., Pedersen, H., Chin, C-K. 1992. Enhanced somatic embryo production by conditioned media in cell suspension cultures of Daucus carota. Biotechnol Lett 14:837-840.
- Clapham; et al. "Gene Transfer by Particle Bombardment to Embryogenic Cultures of Picea abies and the Production of Transgenic Plantlets". Scandinavian Journal of Forest Research. 15: 151–160. doi:10.1080/028275800750014957.
- Jiménez V.M., Guevara E., Herrera J. and Bangerth F. 2001. Endogenous hormone levels in habituated nucellar Citrus callus during the initial stages of regeneration. Plant Cell Rep. 20: 92–100.
- Flinn, B.S.; Roberts, D.R.; Webb, D.T.; Sutton, B.C. 1991. Storage protein changes during zygotic embryogenesis in interior spruce. Tree Physiol. 8:71–81. (Cite in Beardmore et al. 1997).
- Beardmore, T.L.; Wetzel, S.; Regan, S.M. 1997. Poplar seed storage proteins. Chapt. 17, p. 131–142 in Klopfenstein, N.B.; Chun, Y.W.; Kim, M.S.; Ahuja, M.R. (Eds.), Dillon, M.C.; Carman, R.C.; Eskew, L.G. (Tech. Eds.) 1997. Micropropagation, genetic engineering, and molecular biology of Populus. USDA, For. Serv., Rocky Mountain Res. Sta., Fort Collins CO, Gen. Tech. Rep. RM-GTR-297.
- Grossnickle, S.C.; Roberts, D.R.; Major, J.E.; Folk, R.S.; Webster, F.B.; Sutton, B.C.S. 1992. Integration of somatic embryogenesis into operational forestry: comparison of interior spruce emblings and seedlings during production of 1+0 stock. p. 106–113 in Landis, T.D. (Tech. Coord.). Proc. Intermountain Forest Nursery Association, Aug. 1991, Park City UT. USDA, For. Serv., Rocky Mount. For. Range Exp. Sta., Fort Collins CO, Gen. Tech. Rep. RM-211.
- Grossnickle, S.C.; Major, J.E. 1992. Interior spruce seedlings compared to emblings produced from somatic embryogenesis. 2. Physiological response and morphological development on a reforestation site. p. 98 (abstr.) in Colombo, S.J.; Hogan, G.; Wearn, V. (Compilers & Eds.), Proc. 12th North Amer., For. Biol. Workshop: The Role of Physiology and Genetics in Forest Ecosystem Research and Monitoring, Sault Ste. Marie ON, Aug. 1992. Ont. Min. Nat. Res., Ont. For. Res. Instit., and For. Can., Ont. Region.
- Yang, Xiyan and Zhang, Xianlong(2010) 'Regulation of Somatic embryogenesis in Higher Plants', Critical Reviews in Plant Sciences, 29: 1, 36 — 57
- Von Arnold S, Sabala I, Bozhkov P, Dyachok J and Filonova L (2002) Developmental pathways of somatic embryogenesis. Plant Cell Tiss Org. Cult. 69: 233–249
- Jime ́nez VM, Thomas C (2005) Participation of plant hormones in determination and progression of somatic embryogenesis. in: Mujib A, Šamaj J (eds) Somatic embryogenesis. Springer, Berlin, pp 103–118.
- Fehér, Attila. Why somatic plant cells start to form embryos? In: Mujid, Abdul and Samaj, Josef. eds. Somatic Embryogenesis. Plant Cell Monographs, Springer; Berlin/Heidelberg, 2005, vol. 2, p. 85-101.
- Toonen, M. A. J., Hendriks, T., Schmidt, E. D. L., Verhoeven, H. A., van Kammen, A., and De Vries, S. C. 1994. Description of somatic-embryo- forming single cells in carrot suspension cultures employing video cell tracking. Planta 194: 565–572.
- Häggman H.; Vuosku J.; Sarjala T.; Jokela A.; Niemi K. Somatic Embryogenesis of pine species: from functional genomics to plantation forestry. Dig. Plant Cell Monogr. 2: 119–140; 2006.
- Mullin; Lee, eds. (2013). BEST PRACTICE FOR TREE BREEDING IN EUROPE. Skogforsk. ISBN 978-91-977649-6-4.