Sodium MRI
Sodium MRI (also known as 23Na-MRI) is a specialised magnetic resonance imaging technique that uses strong magnetic fields, magnetic field gradients, and radio waves to generate images of the distribution of sodium in the body, as opposed to more common forms of MRI that utilise protons (or hydrogen) present in water (1H-MRI).[1][2] Like the proton, sodium is naturally abundant in the body, so can be imaged directly without the need for contrast agents or hyperpolarization. Furthermore, sodium ions play a role in important biological processes via their contribution to concentration and electrochemical gradients across cellular membranes, making it of interest as an imaging target in health and disease.[3]
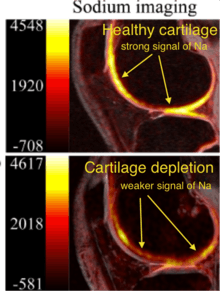
In contrast to conventional MRI of the proton, Sodium MRI is complicated by the low concentrations of Na nuclei relative to concentration of H2O molecules in biological tissues[4] (10-45 mM) and the lower gyromagnetic ratio of the 23Na nucleus as compared to a 1H nucleus,.[5][6] This causes low NMR sensitivity and the requirement for a stronger magnetic field for equivalent spatial resolution. The quadrupolar 23Na nucleus also has a faster transverse relaxation rates and multiple quantum coherences as compared to the 1H nucleus,[6] requiring specialized and high performance MRI sequences to capture information before the contrast used to image the body is lost.
Biological significance
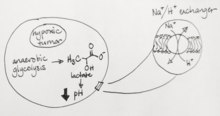
Tissue sodium concentration (TSC) is tightly regulated by healthy cells and are altered by energy status and cellular integrity, making it an effective marker for disease states.[4][6][7] Cells maintain a low intracellular Na+ concentration by actively pumping Na ions out via the Na+/K+ ATPase channel, and any challenge to the cell’s metabolism causing low ATP supply or compromise of the cell’s membrane integrity will drastically increase intracellular Na+ concentrations. After exhaustive exercise, for example, 23Na MRI can detect Na+ levels in tissues rising sharply, and can even visualize a sodium-rich meal in a patient’s stomach. Malignant tumors in particular alter their metabolism drastically, often to account for hypoxic intratumor conditions, leading to an decrease in cytosolic pH. To compensate, Na+ ions from the extracellular space are exchanged for protons in the Na+/H+ antiport,[6] the loss of which often attenuates cancer growth.[8] Therefore, 23Na MRI is a useful clinical tool for detecting a number of disease states, including heart disease[9] and cancer, as well as monitoring therapy. Tissue damage in stroke patients can be evaluated using 23Na MRI, with one study showing that a change of 50% higher TSC than the TSC in healthy brain tissue is consistent with complete infarction,[10] and therefore can be used to determine tissue viability and treatment options for the patient. Tumor malignancy can also be evaluated based on the increases in TSC of rapidly proliferating cells. Malignant tumors have approximately 50-60% increased TSC relative to that of healthy tissues[10] – however, increases in TSC cannot be determined to be due to changes in extracellular volume, intracellular sodium content or neovascularization. Another interesting use of 23Na MRI is in evaluating multiple sclerosis, wherein accumulation of sodium in axons can lead to axon degeneration.[11] Preliminary studies have shown that there is a positive correlation between elevated TSC and disability.
Uses in Prostate Cancer
Recently, work has been undertaken to assess the utility of using sodium-MRI to characterize prostate cancer lesions in men.[12] In this study, patients were imaged with sodium MRI prior to surgical removal of the prostate. TSC was extracted from the images and compared to the Gleason score of imaged lesions. This work showed statistically significant increases in TSC as prostate cancer increased in aggression. This preliminary study suggests that sodium MRI can accurately characterize the stage of prostate cancer. This suggests the potential use of sodium-MRI to better management and stage patients with prostate cancer into treatment schemes.
Advantages
23Na MRI measures cellular metabolic rate as well as disease-related change in tissues and organs.[13] It had improved from 45min length to only 15 mins at 1.5T.[6][10] Unlike other MRI scanning, sodium MRI captures only sodium signals inside bodies. For cartilage degeneration, proteoglycan degrades with negative charge, and positively charged sodium ion bond with proteoglycan.[2] Both proteoglycan and sodium level decrease, so less signals are observed by sodium MRI. 23Na MRI is very sensitive and specific to change in proteoglycan, so it is good to use for monitoring of proteoglycan degeneration in cartilage.[2][14]
References
- Ouwerkerk, Ronald; Morgan, Russell H. (2007-10-01). "23Na MRI: From Research to Clinical Use". Journal of the American College of Radiology. 4 (10): 739–741. doi:10.1016/j.jacr.2007.07.001. ISSN 1546-1440. PMC 2084082. PMID 17903762.
- Borthakur, A; Shapiro, E. M; Beers, J; Kudchodkar, S; Kneeland, J. B; Reddy, R (2000-07-01). "Sensitivity of MRI to proteoglycan depletion in cartilage: comparison of sodium and proton MRI". Osteoarthritis and Cartilage. 8 (4): 288–293. doi:10.1053/joca.1999.0303. PMID 10903883.
- Madelin, Guillaume (2012-12-18). "Sodium Magnetic Resonance Imaging: Biomedical Applications". arXiv:1212.4400 [physics.med-ph].
- Romanzetti, Sandro; Mirkes, Christian C.; Fiege, Daniel P.; Celik, Avdo; Felder, Jörg; Shah, N. Jon (2014-08-01). "Mapping tissue sodium concentration in the human brain: a comparison of MR sequences at 9.4Tesla". NeuroImage. 96: 44–53. doi:10.1016/j.neuroimage.2014.03.079. ISSN 1095-9572. PMID 24721332.
- Madelin, Guillaume; Lee, Jae-Seung; Regatte, Ravinder R.; Jerschow, Alexej (2014-05-01). "Sodium MRI: Methods and applications". Progress in Nuclear Magnetic Resonance Spectroscopy. 79: 14–47. doi:10.1016/j.pnmrs.2014.02.001. PMC 4126172. PMID 24815363.
- Madelin, Guillaume; Regatte, Ravinder R. (2013-09-01). "Biomedical Applications of Sodium MRI In Vivo". Journal of Magnetic Resonance Imaging. 38 (3): 511–529. doi:10.1002/jmri.24168. ISSN 1053-1807. PMC 3759542. PMID 23722972.
- Ouwerkerk, Ronald (2011-01-01). "Sodium MRI". In Modo, Michel; Bulte, Jeff W.M. (eds.). Magnetic Resonance Neuroimaging. Methods in Molecular Biology. 711. Humana Press. pp. 175–201. doi:10.1007/978-1-61737-992-5_8. ISBN 9781617379918. PMID 21279602.
- Alevizopoulos, Konstantinos; Calogeropoulou, Theodora; Lang, Florian; Stournaras, Christos (2014-01-01). "Na+/K+ ATPase inhibitors in cancer". Current Drug Targets. 15 (10): 988–1000. ISSN 1873-5592. PMID 25198786.
- Bottomley, Paul A. (2016-02-01). "Sodium MRI in human heart: a review". NMR in Biomedicine. 29 (2): 187–196. doi:10.1002/nbm.3265. ISSN 1099-1492. PMC 4868405. PMID 25683054.
- Ouwerkerk, Ronald; Bleich, Karen B.; Gillen, Joseph S.; Pomper, Martin G.; Bottomley, Paul A. (2003-05-01). "Tissue Sodium Concentration in Human Brain Tumors as Measured with 23Na MR Imaging". Radiology. 227 (2): 529–537. doi:10.1148/radiol.2272020483. ISSN 0033-8419. PMID 12663825.
- Inglese, M.; Madelin, G.; Oesingmann, N.; Babb, J. S.; Wu, W.; Stoeckel, B.; Herbert, J.; Johnson, G. (2010-03-01). "Brain tissue sodium concentration in multiple sclerosis: a sodium imaging study at 3 tesla". Brain. 133 (3): 847–857. doi:10.1093/brain/awp334. ISSN 0006-8950. PMC 2842511. PMID 20110245.
- Broeke, NC.; Peterson, J.; Lee, J.; Martin, PA.; Farag, A.; Gomez, J.; Moussa, M.; Gaed, M.; Chin, J.; Pautler, SE.; Ward, A.; Bauman, G.; Bartha, R.; Scholl, TJ. (2018-08-24). "Characterization of Clinical Human Prostate Cancer Lesions Using 3.0-T Sodium MRI Registered to Gleason-Graded Whole-Mount Histopathology". Journal of Magnetic Resonance Imaging. 49: 1409–1419. doi:10.1002/jmri.26336. PMID 30430700.
- Madelin, Guillaume; Kline, Richard; Walvick, Ronn; Regatte, Ravinder R. (2014-04-23). "A method for estimating intracellular sodium concentration and extracellular volume fraction in brain in vivo using sodium magnetic resonance imaging". Scientific Reports. 4: 4763. Bibcode:2014NatSR...4E4763M. doi:10.1038/srep04763. PMC 4762219. PMID 24755879.
- Newbould, R.D.; Miller, S.R.; Tielbeek, J.A.W.; Toms, L.D.; Rao, A.W.; Gold, G.E.; Strachan, R.K.; Taylor, P.C.; Matthews, P.M. (2012-01-01). "Reproducibility of sodium MRI measures of articular cartilage of the knee in osteoarthritis". Osteoarthritis and Cartilage. 20 (1): 29–35. doi:10.1016/j.joca.2011.10.007. ISSN 1063-4584. PMC 3270258. PMID 22040861.