Cuprate superconductor
Cuprate superconductors are high-temperature superconductors made of layers of copper oxides (CuO2) alternating with layer of charge reservoirs (CR), which are oxides of other metals.
History
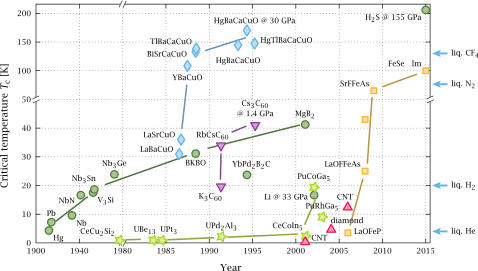
Interest in cuprates sharply increased in 1986 with the discovery of high-temperature superconductivity in the non-stoichiometric cuprate lanthanum barium copper oxide. The Tc for this material was 35 K, well above the previous record of 23 K.[1] Thousands of publications examine the superconductivity in cuprates between 1986 and 2001,[2] and Bednorz and Müller were awarded the Nobel Prize in Physics only a year after their discovery.[3]
From 1986, many cuprate superconductors were identified, and can be put into three groups on a phase diagram critical temperature vs. oxygen hole content and copper hole content:
- lanthanum barium- (LB-CO), Tc=-240 °C (35 K).
- yttrium barium- (YB-CO), Tc=-215 °C (60 K).
- the Bi, Tl, Hg-based:
- bismuth strontium calcium- (BiSC-CO), Tc=-180 °C (95 K).
- thallium barium calcium- (TBC-CO), Tc=-150 °C (125 K).[4]
- mercury barium calcium- (HGBC-CO) 1993, with Tc=-140 °C (135 K), currently the highest cuprate critical temperature.[5][6]
Structure

Cuprate superconductors usually feature copper oxides in both the oxidation states 3+ and 2+. For example, YBa2Cu3O7 is described as Y3+(Ba2+)2(Cu3+)(Cu2+)2(O2−)7. All superconducting cuprates are layered materials having a complex structure described as a superlattice of superconducting CuO2 layers separated by spacer layers, where the misfit strain between different layers and dopants in the spacers induce a complex heterogeneity that in the superstripes scenario is intrinsic for high-temperature superconductivity.
Applications
BSCCO superconductors already have large-scale applications. For example, tens of kilometers of BSCCO-2223 at 77 K superconducting wires are being used in the current leads of the Large Hadron Collider at CERN.[7] (but the main field coils are using metallic lower temperature superconductors, mainly based on niobium–tin).
See also
Bibliography
References
- J. G. Bednorz; K. A. Mueller (1986). "Possible high TC superconductivity in the Ba-La-Cu-O system". Z. Phys. B. 64 (2): 189–193. Bibcode:1986ZPhyB..64..189B. doi:10.1007/BF01303701.
- Mark Buchanan (2001). "Mind the pseudogap". Nature. 409 (6816): 8–11. doi:10.1038/35051238. PMID 11343081.
- Nobel prize autobiography.
- Sheng, Z. Z.; Hermann A. M. (1988). "Bulk superconductivity at 120 K in the Tl–Ca/Ba–Cu–O system". Nature. 332 (6160): 138–139. Bibcode:1988Natur.332..138S. doi:10.1038/332138a0.
- Schilling, A.; Cantoni, M.; Guo, J. D.; Ott, H. R. (1993). "Superconductivity above 130 K in the Hg–Ba–Ca–Cu–O system". Nature. 363 (6424): 56–58. Bibcode:1993Natur.363...56S. doi:10.1038/363056a0.
- Lee, Patrick A. (2008). "From high temperature superconductivity to quantum spin liquid: progress in strong correlation physics". Reports on Progress in Physics. 71: 012501. arXiv:0708.2115. Bibcode:2008RPPh...71a2501L. doi:10.1088/0034-4885/71/1/012501.
- Amalia Ballarino (November 23, 2005). "HTS materials for LHC current leads". CERN.