Cold hardening
Cold hardening is the physiological and biochemical process by which an organism prepares for cold weather.
Plants
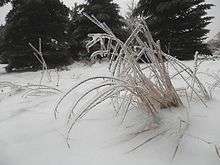
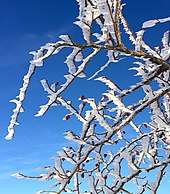
Plants in temperate and polar regions adapt to winter and sub zero temperatures by relocating nutrients from leaves and shoots to storage organs.[1] Freezing temperatures induce dehydrative stress on plants, as water absorption in the root and water transport in the plant decreases.[2] Water in and between cells in the plant freezes and expands, causing tissue damage. Cold hardening is a process in which a plant undergoes physiological changes to avoid, or mitigate cellular injuries caused by sub-zero temperatures.[1] Non-acclimatized individuals can survive −5 °C, while an acclimatized individual in the same species can survive −30°C. Plants that originated in the tropics, like tomato or maize, don't go through cold hardening and are unable to survive freezing temperatures.[3] The plant starts the adaptation by exposure to cold yet still not freezing temperatures. The process can be divided into three steps. First the plant perceives low temperature, then converts the signal to activate or repress expression of appropriate genes. Finally, it uses these genes to combat the stress, caused by sub-zero temperatures, affecting its living cells. Many of the genes and responses to low temperature stress are shared with other abiotic stresses, like drought or salinity.[2]
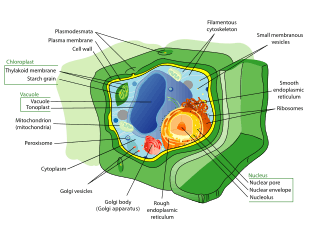
When temperature drops, the membrane fluidity, RNA and DNA stability, and enzyme activity change. These, in turn, affect transcription, translation, intermediate metabolism, and photosynthesis, leading to an energy imbalance. This energy imbalance is thought to be one of the ways the plant detects low temperature. Experiments on arabidopsis show that the plant detects the change in temperature, rather than the absolute temperature.[2] The rate of temperature drop is directly connected to the magnitude of calcium influx, from the space between cells, into the cell. Calcium channels in the cell membrane detect the temperature drop, and promotes expression of low temperature responsible genes in alfalfa and arabidopsis. The response to the change in calcium elevation depends on the cell type and stress history. Shoot tissue will respond more than a root cells, and a cell that already is adapted to cold stress will respond more than one that has not been through cold hardening before. Light doesn't control the onset of cold hardening directly, but shortening of daylight is associated with fall, and starts production of reactive oxygen species and excitation of photosystem 2, which influences low-temp signal transduction mechanisms. Plants with compromised perception of day length have compromised cold acclimation.[2]
Cold increases cell membrane permeability[4] and makes the cell shrink, as water is drawn out when ice is formed in the extracellular matrix between cells.[2] To retain the surface area of the cell membrane so it will be able to regain its former volume when temperature rises again, the plant forms more and stronger Hechtian strands. These are tubelike structures that connect the protoplast with the cell wall. When the intracellular water freezes, the cell will expand, and without cold hardening the cell would rupture. To protect the cell membrane from expansion induced damage, the plant cell changes the proportions of almost all lipids in the cell membrane, and increases the amount of total soluble protein and other cryoprotecting molecules, like sugar and proline.[3]
Chilling injury occurs at 0–10 degrees Celsius, as a result of membrane damage, metabolic changes, and toxic buildup. Symptoms include wilting, water soaking, necrosis, chlorosis, ion leakage, and decreased growth. Freezing injury may occur at temperatures below 0 degrees Celsius. Symptoms of extracellular freezing include structural damage, dehydration, and necrosis. If intracellular freezing occurs, it will lead to death. Freezing injury is a result of lost permeability, plasmolysis, and post-thaw cell bursting.
When spring comes, or during a mild spell in winter, plants de-harden, and if the temperature is warm for long enough – their growth resumes.[1]
Insects
Cold hardening has also been observed in insects such as the fruit fly and diamondback moth. The insects use rapid cold hardening to protect against cold shock during overwintering periods.[5][6] Overwintering insects stay awake and active through the winter while non-overwintering insects migrate or die. Rapid cold hardening can be experienced during short periods of undesirable temperatures, such as cold shock in environment temperature, as well as the common cold months. The buildup of cryoprotective compounds is the reason that insects can experience cold hardening.[5] Glycerol is a cryoprotective substance found within these insects capable of overwintering. Through testing, glycerol requires interactions with other cell components within the insect in order to decrease the body's permeability to the cold.[5] When an insect is exposed to these cold temperatures, glycerol rapidly accumulates. Glycerol is known as a non-ionic kosmotrope forming powerful hydrogen bonds with water molecules. The hydrogen bonds in the glycerol compound compete with the weaker bonds between the water molecules causing an interruption in the makeup of ice formation.[7] This chemistry found within the glycerol compound and reaction between water has been used as an antifreeze in the past, and can be seen here when concerning cold hardening. Proteins also play a large role in the cryoprotective compounds that increase ability to survive the cold hardening process and environmental change. Glycogen phosphorylase (GlyP) has been a key protein found during testing to increase in comparison to a controlled group not experiencing the cold hardening.[8] Once warmer temperatures are observed the process of acclimation begins, and the increased glycerol along with other cryoprotective compounds and proteins are also reversed. There is a rapid cold hardening capacity found within certain insects that suggests not all insects can survive a long period of overwintering. Non-diapausing insects can sustain brief temperature shocks but often have a limit to which they can handle before the body can no longer produce enough cryoprotective components.
.jpg)
Inclusive to the cold hardening process being beneficial for insects survival during cold temperatures, it also helps improve the organisms performance.[9] Rapid cold hardening (RCH) is one of the fastest cold temperature responses recorded.[9] This process allows an insect to instantly adapt to the severe weather change without compromising function. The Drosophila melanogaster (common fruit fly) is a frequently experimented insect involving cold hardening. A proven example of RCH enhancing organisms performance comes from courting and mating within the fruit fly. It has been tested that the fruit fly mated more frequently once RCH has commenced in relation to a controlled insect group not experiencing RCH.[9] Most insects experiencing extended cold periods are observed to modify the membrane lipids within the body. Desaturation of fatty acids are the most commonly seen modification to the membrane.[9] When the fruit fly was observed under the stressful climate the survival rate increased in comparison to the fly prior to cold hardening.
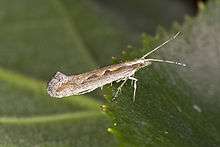
In addition to testing on the common fruit fly, Plutella xylostella (diamondback moth) also has been widely studied for its significance in cold hardening. While this insect also shows an increase in glycerol and similar cryoprotective compounds, it also shows an increase in polyols. These compounds are specifically linked to cryoprotective compounds designed to withstand cold hardening. The polyol compound is freeze-susceptible and freeze tolerant.[10] Polyols simply act as a barrier within the insect body by preventing intracellular freezing by restricting the extracellular freezing likely to happen in overwintering periods.[10] During the larvae stage of the diamondback moth, the significance of glycerol was tested again for validity. The lab injected the larvae with added glycerol and in turn proved that glycerol is a major factor in survival rate when cold hardening. The cold tolerance is directly proportional to the buildup of glycerol during cold hardening.[10]
Cold hardening of insects improves the survival rate of the species and improves function. Once environmental temperature begins to warm up above freezing, the cold hardening process is reversed and the glycerol and cryprotective compounds decrease within the body. This also reverts the function of the insect to pre-cold hardening activity.
References
- Thorsen, Stig Morten; Höglind, Mats (2010-12-15). "Modelling cold hardening and dehardening in timothy. Sensitivity analysis and Bayesian model comparison". Agricultural and Forest Meteorology. 150 (12): 1529–1542. doi:10.1016/j.agrformet.2010.08.001.
- Smallwood, Maggie; Bowles, Dianna J. (2002-07-29). "Plants in a cold climate". Philosophical Transactions of the Royal Society B: Biological Sciences. 357 (1423): 831–847. doi:10.1098/rstb.2002.1073. ISSN 0962-8436. PMC 1692998. PMID 12171647.
- McKhann, Heather I.; Gery, Carine; Bérard, Aurélie; Lévêque, Sylvie; Zuther, Ellen; Hincha, Dirk K.; De Mita, S.; Brunel, Dominique; Téoulé, Evelyne (2008-01-01). "Natural variation in CBF gene sequence, gene expression and freezing tolerance in the Versailles core collection of Arabidopsis thaliana". BMC Plant Biology. 8: 105. doi:10.1186/1471-2229-8-105. ISSN 1471-2229. PMC 2579297. PMID 18922165.
- Forbes, James C.; Watson, Drennan (1992-08-20). Plants in Agriculture. Cambridge University Press. ISBN 9780521427913.
- Chen, CP; Denlinger, DL; Lee, RE (1987). "A rapid cold-hardening process in insects". Science. 238 (4832): 1415–7. doi:10.1126/science.238.4832.1415. PMID 17800568. S2CID 39842087.
- Lee, RE; Czajka, MC (1990). "A rapid cold-hardening response protecting against cold shock injury in Drosophila melanogaster". J Exp Biol. 148: 245–54. PMID 2106564.
- Duman, J (2002). "The inhibition of ice nucleators by insect antifreeze proteins is enhanced by glycerol and citrate". Journal of Comparative Physiology B. 172 (2): 163–168. doi:10.1007/s00360-001-0239-7. PMID 11916110.
- Overgaard, J.; Sørensen, J. G.; Com, E.; Colinet, H. (2013). "The rapid cold hardening response of Drosophila melanogaster: Complex regulation across different levels of biological organization". Journal of Insect Physiology. 62: 46–53. doi:10.1016/j.jinsphys.2014.01.009. PMID 24508557.
- Lee, R. E.; Damodaran, K.; Yi, S. X.; Lorigan, G. A. (2006). "Rapid Cold-Hardening Increases Membrane Fluidity and Cold Tolerance of Insect Cells". Cryobiology. 52 (3): 459–463. doi:10.1016/j.cryobiol.2006.03.003. PMID 16626678.
- Park, Y.; Kim, Y. (2014). "A specific glycerol kinase induces rapid cold hardening of the diamondback moth, Plutella xylostella". Journal of Insect Physiology. 67: 56–63. doi:10.1016/j.jinsphys.2014.06.010. PMID 24973793.